Down here at the bottom of our ocean of air, it’s easy to get complacent about the hazards our universe presents. We feel safe from the dangers of the vacuum of space, where radiation sizzles and rocks whizz around. In the same way that a catfish doesn’t much care what’s going on above the surface of his pond, so too are we content that our atmosphere will deflect, absorb, or incinerate just about anything that space throws our way.
Or will it? We all know that there are things out there in the solar system that are more than capable of wiping us out, and every day holds a non-zero chance that we’ll take the same ride the dinosaurs took 65 million years ago. But if that’s not enough to get you going, now we have to worry about gamma-ray bursts, searing blasts of energy crossing half the universe to arrive here and dump unimaginable amounts of energy on us, enough to not only be measurable by sensitive instruments in space but also to effect systems here on the ground, and in some cases, to physically alter our atmosphere.
Gamma-ray bursts are equal parts fascinating physics and terrifying science fiction. Here’s a look at the science behind them and the engineering that goes into detecting and studying them.
Collapsars and Neutron Stars
Although we now know that gamma-ray bursts are relatively common, it wasn’t all that long ago that we were ignorant of their existence, thanks in part to our thick, protective atmosphere. The discovery of GRBs had to wait for the Space Race to couple with Cold War paranoia, which resulted in Project Vela, a series of early US Air Force satellites designed in part to watch for Soviet compliance with the Partial Test Ban Treaty, which forbade everything except underground nuclear tests. In 1967, gamma ray detectors on satellites Vela 3 and Vela 4 saw a flash of gamma radiation that didn’t match the signature of any known nuclear weapon. Analysis of the data from these and subsequent flashes revealed that they came from space, and the race to understand these energetic cosmic outbursts was on.
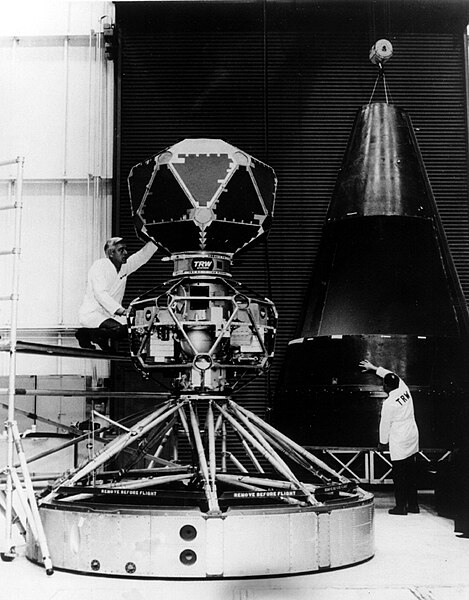
Gamma-ray bursts are the most energetic phenomena known, with energies that are almost unfathomable. Their extreme brightness, primarily as gamma rays but across the spectrum and including visible light, makes them some of the most distant objects ever observed. To put their energetic nature into perspective, a GRB in 2008, dubbed GRB 080319B, was bright enough in the visible part of the spectrum to just be visible to the naked eye even though it was 7.5 billion light years away. That’s more than halfway across the observable universe, 3,000 times farther away than the Andromeda galaxy, normally the farthest naked-eye visible object.
For all their energy, GRBs tend to be very short-lived. GRBs break down into two rough groups. Short GRBs last for less than about two seconds, with everything else falling into the long GRB category. About 70% of GRBs we see fall into the long category, but that might be due to the fact that the short bursts are harder to see. It could also be that the events that precipitate the long variety, hypernovae, or the collapse of extremely massive stars and the subsequent formation of rapidly spinning black holes, greatly outnumber the progenitor event for the short category of GRBs, which is the merging of binary neutron stars locked in a terminal death spiral.
The trouble is, the math doesn’t work out; neither of these mind-bogglingly energetic events could create a burst of gamma rays bright enough to be observed across half the universe. The light from such a collapse would spread out evenly in all directions, and the tyranny of the inverse square law would attenuate the signal into the background long before it reached us. Unless, of course, the gamma rays were somehow collimated. The current thinking is that a disk of rapidly spinning material called an accretion disk develops outside the hypernova or the neutron star merger. The magnetic field of this matter is tortured and twisted by its rapid rotation, with magnetic lines of flux getting tangled and torn until they break. This releases all the energy of the hypernova or neutron star merger in the form of gamma rays in two tightly focused jets aligned with the pole of rotation of the accretion disk. And if one of those two jets happens to be pointed our way, we’ll see the resulting GRB.
Crystals and Shadows
But how exactly do we detect gamma-ray bursts? The first trick is to get to space, or at least above the bulk of the atmosphere. Our atmosphere does a fantastic job shielding us from all forms of cosmic radiation, which is why the field of gamma-ray astronomy in general and the discovery of GRBs in particular had to wait until the 1960s. A substantial number of GRBs have been detected by gamma-ray detectors carried aloft on high-altitude balloons, especially in the early days, but most dedicated GRB observatories are now satellite-borne
Gamma-ray detection technology has advanced considerably since the days of Vela, but a lot of the tried and true technology is still used today. Scintillation detectors, for example, use crystals that release photons of visible light when gamma rays of a specific energy pass through them. The photons can then be amplified by photomultiplier tubes, resulting in a pulse of current proportional to the energy of the incident gamma ray. This is the technology used by the Gamma-ray Burst Monitor (GBM) aboard the Fermi Gamma-Ray Space Telescope, a satellite that was launched in 2008. Sensors with the GBT are mounted around the main chassis of Fermi, giving it a complete very of the sky. It consists of twelve sodium iodide detectors, each of which is directly coupled to a 12.7-cm diameter photomultiplier tube. Two additional sensors are made from cylindrical bismuth germanate scintillators, each of which is sandwiched between two photomultipliers. Together, the fourteen sensors cover from 8 keV to 30 MeV, and used in concert they can tell where in the sky a gamma-ray burst has occurred.
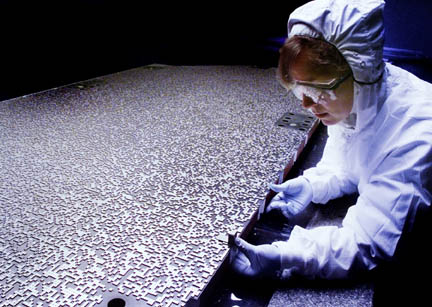
Ionization methods are also used as gamma-ray detectors. The Niel Gehrels Swift Observatory, a dedicated GRB hunting satellite that was launched in 2004, has an instrument known as the Burst Alert Telescope, or BAT. This instrument has a very large field of view and is intended to monitor a huge swath of sky. It uses 32,768 cadmium-zinc-telluride (CZT) detector elements, each 4 x 4 x 2 mm, to directly detect the passage of gamma rays. CZT is a direct-bandgap semiconductor in which electron-hole pairs are formed across an electric field when hit by ionizing radiation, producing a current pulse. The CZT array sits behind a fan-shaped coded aperture, which has thousands of thin lead tiles arranged in an array that looks a little like a QR code. Gamma rays hit the coded aperture first, casting a pattern on the CZT array below. The pattern is used to reconstruct the original properties of the radiation beam mathematically, since conventional mirrors and lenses don’t work with gamma radiation. The BAT is used to rapidly detect the location of a GRB and to determine if it’s something worth looking at. If it is, it rapidly slews the spacecraft to look at the burst with its other instruments and instantly informs other gamma observatories about the source so they can take a look too.
The B.O.A.T.
On October 9, 2022, both Swift and Fermi, along with dozens of other spacecraft and even some ground observatories, would get to witness a cataclysmically powerful gamma-ray burst. Bloodlessly named GRB 221009A but later dubbed “The BOAT,” for “brightest of all time,” the initial GRB lasted for an incredible ten minutes with a signal that remained detectable for hours. Coming from the direction of the constellation Sagittarius from a distance of 2.4 billion light years, the burst was powerful enough to saturate Fermi’s sensors and was ten times more powerful than any signal yet received by Swift.
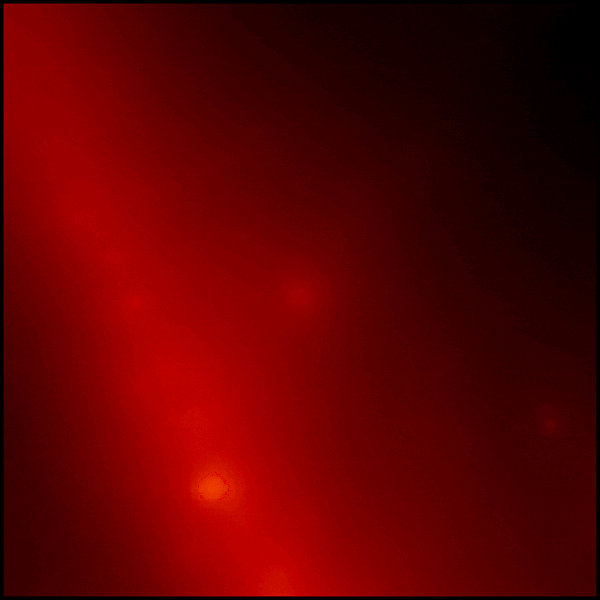
Almost everything about the BOAT is fascinating, and the superlatives are too many to list. The gamma-ray burst was so powerful that it showed up in the scientific data of spacecraft that aren’t even equipped with gamma-ray detectors, including orbiters at Mars and Voyager 1. Ground-based observatories noted the burst, too, with observatories in Russia and China noting very high-energy photons in the range of tens to hundreds of TeV arriving at their detectors.
The total energy released by GRB 221009A is hard to gauge with precision, mainly because it swamped the very instruments designed to measure it. Estimates range from 1048 to 1050 joules, either of which dwarfs the total output of the Sun over its entire 10 billion-year lifespan. So much energy was thrown in our direction in such a short timespan that even our own atmosphere was impacted. Lightning detectors in India and Germany were triggered by the burst, and the ionosphere suddenly started behaving as if a small solar flare had just occurred. Most surprising was that the ionospheric effects showed up on the daylight side of the Earth, swamping the usual dampening effect of the Sun.
When the dust had settled from the initial detection of GRB 221009A, the question remained: What happened to cause such an outburst? To answer that, the James Webb Space Telescope was tasked with peering into space, off in the direction of Sagittarius, where it found pretty much what was expected — the remains of a massive supernova. In fact, the supernova that spawned this GRB doesn’t appear to have been particularly special when compared to other supernovae from similarly massive stars, which leaves the question of how the BOAT got to be so powerful.
Does any of this mean that a gamma-ray burst is going to ablate our atmosphere and wipe us out next week? Probably not, and given that this recent outburst was estimated to be a one-in-10,000-year event, we’re probably good for a while. It seems likely that there’s plenty that we don’t yet understand about GRBs, and that the data from GRB 221009A will be pored over for decades to come. It could be that we just got lucky this time, both in that we were in the right place at the right time to see the BOAT, and that it didn’t incinerate us in the process. But given that on average we see one GRB per day somewhere in the sky, chances are good that we’ll have plenty of opportunities to study these remarkable events.
The energy released here is mind-boggling.
Energy and mass are the same thing, right? E=m * c^2. If the energy was 10^50 Joules, then we can easily calculate the mass equivalent of that energy – 10^50 / (9 * 10^16) = 1.1 * 10^33 kg.
The mass of the sun is about 10^30 kg, so the energy released here is about a thousand times the mass of the sun. Not just the energy the sun will put out in its lifetime – only a small fraction of the sun’s mass is turned into energy. It’s a thousand times the energy you would get if the entire sun were converted to energy, released in a few minutes.
This argues for a sun that has a radius ~ 10x the radius of ours (or 20x the diameter) which, as I understand it, isn’t at the upper part of the scale of such things. Imagine what happens if a REALLY big one lets go!
Yeah, no, that’s not actually how this works: this is (unfortunately) terminology silliness.
That energy is something called the isotropic equivalent energy: it’s “this is the energy this would produce if the GRB produced the same energy in all directions.”
It doesn’t – it’s only produced in a narrow angle. It’s not actually putting out that much energy (which would be impossible, it’s more than the mass of the star that produced it).
It might seem silly to report it this way, but it’s because you report both the isotropic equivalent energy and the opening angle estimate separately (you can get the opening angle based on when the light curve breaks). The total energy is then roughly (angle squared)/2 times the energy because the angle is small (typically ~0.05 rad in scale).
So, for instance, 10^50 J “isotropic equivalent” here would actually be around 10^47 J, so it’d actually be about the equivalent of 1 solar mass converted to energy.
But – here’s the second caveat. That number isn’t associated with this GRB. It’s listed on Wikipedia, but the paper that it comes from is calculating what the maximum Eiso could be for a 10 Msun progenitor (maximally rotating), to figure out if the models they’re using are crazy or not. I don’t know how that got associated with this GRB.
The “best guess” for Eiso for this guy is 10^47 J (10^55 erg), and then a total energy of probably more like 10^44 J, or around 0.1% of the mass of the Sun.
So sadly, nothing totally crazy.
Damnit, not 10 Msun progenitor, 10 Msun remnant (as in, the size of the black hole left over). Oops. Although I guess technically it’s the progenitor of the GRB (the idea is that the GRB is formed by a remnant spinning stupid fast losing its rotational energy ludicrously quickly).
Argh, freaking ergs (although I should probably say ‘freaking joules’, since ergs are older) – it’s 10^48 J Eiso, or probably 10^45 J total, likely around 1% of the Sun, not 0.1%.
I still feel better than the astronomer who commented that ” if you took the entire sun and you converted all of it into pure energy, it still wouldn’t match this event”, which is wrong – he probably (like many other people!) forgot the beaming factor (that angle squared/2).
When I started college dyne, erg, cm, second were still a thing. Maybe even in the earliest editions of Haliday and Resnick!
It’s common in astronomy: the main reason MKS exists is to match electrical engineering units (volts/amps/watts) to physics ones (no way they’d convince the EEs to change!). Obviously, astronomy doesn’t care that much about volts/amps/ohms, so CGS with its ergs it stayed.
ET, pick up the phone, it’s for you!
This is like SF alien wars.
“The total energy released by GRB 221009A is hard to gauge with precision, mainly because it swamped the very instruments designed to measure it.”
“We’re going to need a bigger B.O.A.T!”
-JAWS (paraphrased)
B^)
Coded aperture imaging shown here is pretty neat stuff. Maybe a topic for a HaD article! Not unlike a 2D version of CDMA, or GPS’s Gold codes.
Are you thinking this could be artifact perhaps from telco/gps transmissions?