All of us probably know what neutrons are, or have at least heard of them back in physics class. Yet these little bundles of quarks are much more than just filler inside an atom’s nucleus. In addition to being an essential part of making matter as stable as it (usually) is, free neutrons can be used in a variety of manners.
From breaking atoms apart (nuclear fission), to changing the composition of atoms by adding neutrons (transmutation), to the use of neutrons in detecting water and inspecting materials, neutrons are an essential tool in the sciences, as well as in medicine and industrial applications. This has meant a lot of development toward the goal of better neutron sources. While nuclear fission is an efficient way to get lots of neutrons, for most applications a more compact and less complicated approach is used, some of which use nuclear fusion instead.
In this article we’ll be taking a look at the many applications of neutron sources, and these neutron sources themselves.
The Humble Neutron
Strictly defined, a neutron is a hadron, just like a proton. What this means is that they both are composite particles which consist out of two or more valence quarks. In the case of both the proton and neutron we find that they contain three quarks. This makes them the baryon sub-type of hadrons. The difference is that a neutron has two down quarks and one up quark, whereas a proton has two up quarks and one down quark. Confusing as this may sound, it’s essential to understanding how the sub-atomic world affects everything around us.
Outside of a nucleus, a neutron is unstable, with a half-life of approximately 10 minutes and 11 seconds, after which it beta decays into a proton. One of the neutron’s down quarks emits a W– boson which then decays into an electron and an anti-neutrino:
Or using the quark notation:
About 1 in 1,000 of these beta decays also produce gamma radiation, which is a form of internal bremsstrahlung. This occurs when the emitted beta particle (the electron) interacts with the (positively charged) proton.
Generally a Bit Unstable
Inside a nucleus a neutron isn’t necessarily stable, either. A nuclide (collection of bound neutrons and protons) forms a quantum mechanical system, which may or may not form a stable energy state. Essentially, if there is a lower energy state available within the nuclide, neutron decay will occur. An example of this is carbon-14 (6 protons, 8 neutrons) which decays to the more stable nitrogen-14 (7 protons, 7 neutrons).
During bound neutron decay we see the same process as for free neutron decay. This is distinct from inverse beta decay and electron capture, two processes through which a proton in a nuclide can transform into a neutron. The latter type being useful for our purposes, as we will see in the next section.
Generating Free Neutrons at Will
Since neutrons cannot exist for extended periods of time outside of a nuclide, this means that free neutrons have to be generated where they are needed. The easiest way to accomplish this is to take an isotope which exhibits neutron emission as part of its radioactive decay chain, such as californium-252 or berylium-13:
The obvious disadvantage of using a radioactive isotope as neutron source is that it cannot be turned off, and will emit fewer and fewer neutrons per time unit as more of its nuclides settle into a new state which will be either stable or not emit neutrons when they decay further. A partial solution here is the use of photodisintegration, whereby a gamma ray is used to excite a nuclide to the point where it emits a neutron. For example in the case of the only stable form of berylium:
This interestingly leads us to another fascinating source of neutrons: nuclear fusion of deuterium and tritium (D-T):
The relative ease of using (cheap) D-T fuel in a simple inertial electrostatic confinement (IEC) device (like a fusor) has led to them to become a popular neutron source for both scientific research and medicine, allowing for a device that generates neutrons at will, and at a constant rate over the device’s lifetime.
Neutrons on Mars
The Curiosity rover which has been doing science on Mars for years now has an instrument on board which is called the DAN, which is short for Dynamic Albedo of Neutrons. It uses a neutron beam aimed at the ground from about 80 cm height. The neutrons which are scattered back to its sensors after interacting with the soil provide information about the soil’s contents, specifically its moisture content. This is caused by the interaction of neutrons and hydrogen.
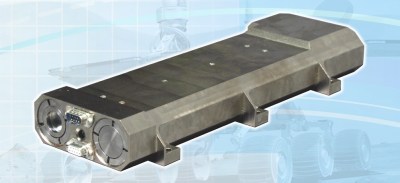
This DAN instrument uses an ING-10K neutron tube-based pulse neutron generator, manufactured by VNIIA (a Rosatom company). It emits 107 neutrons per pulse, rated for 107 pulses during a 3-year lifespan. These neutron generators generally use D-T fusion, using a linear accelerator to accelerate deuterium, tritium, or a combination thereof into a metal hydride target, which also contains these isotopes. With enough energy to overcome the coulomb barrier, these isotopes’ nuclides will fuse and neutrons are emitted.
Neutrons in Medicine
Neutron tubes are similar to the colliding beam fusion concept, along with that of the aforementioned fusor and the polywell in that they use the principle of IEC to achieve fusion. The fusor uses two spherical grids, using opposing charges to accelerate the isotopes, whereas the polywell does essentially the same, but seeks to eliminate these physical grids, to increase the efficiency of the fusion reaction.
Regardless of the exact configuration, such IEC devices have found significant interest in the field of medical
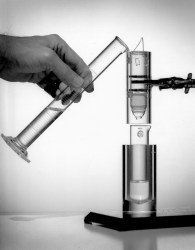
isotopes, specifically molybdenum-99 (99Mo). This particular isotope is the precursor to metastable technetium-99m (99mTc) — its decay product — before the 99mTc decays to 99Tc with a half-life of 6.01 hours. Here, 99mTc is crucial in medicine as a radioactive tracer, seeing regular use for imaging studies of the body as it emits clearly detectable, 104 keV gamma rays.
Unfortunately, the main source of 99Mo is from a small number of fission reactors in which uranium-235 targets are bombarded with neutrons. With these reactors suffering extended downtime due to maintenance and replacement reactors having been cancelled, a shortage of 99Mo is becoming critical. This has led to alternatives being explored, one of which is the use of IEC fusion devices as the source of neutrons.
A promising approach by a company called Phoenix LLC uses a linear accelerator fusion device to generate neutrons which irradiate a uranium target, causing it to fission and generate the 99Mo and other isotopes. The 99Mo can then be separated and transported to hospitals in a technetium-99m generator as is already commonly done every day. This 99Mo production is supposed to start in 2021 with commercial scaling projected for 2022, according to Phoenix.
Neutron Imaging
The imaging of objects using neutrons is very similar to that of using X-rays, with the main difference being the way
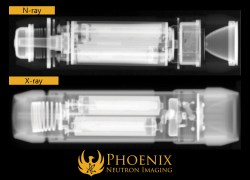
that they produce an image. With X-rays, the resulting image depends on the density of the materials encountered, so that the final image depends on how much the X-rays were attenuated. With neutron imaging, the interaction with the materials determines how many neutrons will reach the sensor and what their physical (molecular) properties are. The result is something similar to an X-ray, but with important differences due to how neutrons interact with the object relative to X-rays, as the image to the right illustrates.
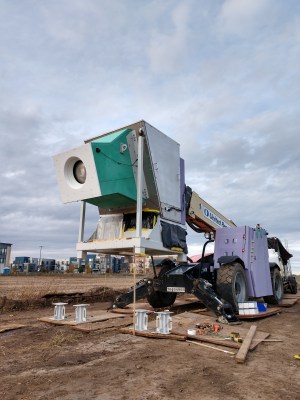
In neutron imaging, after the neutrons have been produced, they have to be slowed down to the desired speed for the imaging. The speed of the neutrons will affect the penetration depth and the final imaging results, allowing for fine-tuning the process. The use of neutron imaging ranges from inspecting finished products — including welds, cast parts, turbine blades, nuclear fuel rods and high-precision parts in industry and beyond — to proposed uses like detecting explosives, such as in a war zone.
Distinct from neutron imaging is neutron activation analysis (NAA), which is essentially what the Curiosity’s DAN module does.
The use of nuclear fusion-based neutron generators is becoming more common for neutron imaging and related, because of the potential for smaller, more efficient devices. The US military’s NEMESIS (Neutron-Emitting Mobile Explosives Sensing and Identification System) program is one example of this, which may enable the use of small devices that can detect explosives like improvised explosive devices (IEDs) and landmines with great ease, even for tricky metal-free landmines that metal detectors and ground-penetrating radar struggle to detect.
Involved in the NEMESIS program is the aforementioned Phoenix LLC, which expresses their belief that such NAA as well as neutron imaging devices can in the future be used for not only dangerous tasks, but also more routine ones such as bridge inspections and presumably inspections in avionics. According to Phoenix, most effort currently goes into improving the detection algorithms, and to make the equipment more rugged and economical.
Beyond Science Fiction
Although a lot of this may sound rather fantastical, such as the ability to ‘look’ inside the ground to find any buried explosives there, or to see fractures inside a turbine blade or weld, one can see this as the progression from commonly used technologies like X-rays. Whereas X-rays and their kin were found to be easy enough to produce during the early 20th century and beyond, the process of producing a large number of neutrons in an efficient manner that did not require the use of a nuclear fission reactor has held progress back for decades.
Fusion devices have many advantages, with maintenance being fairly uncomplicated as well. For non-sealed fusion devices, a continuous supply of deuterium-tritum (or deuterium-deuterium) fuel is supplied, with the device running maintenance-free except for the swapping out of components of the generator that become radioactive due to neutron activation. These components fall under low- to medium-radioactive waste, akin to what laboratories and hospitals produce, making for easy disposal.
While the prospect of a handheld scanning device that uses a neutron source to perform environmental analysis is still a while off, neutron imaging stands a good chance of improving life in a lot of ways, much like X-rays have done.
Thanks for the nice writeup on neutrons. Let me add a less known fact about the neutron here for all of you:
If you manage to slow the neutrons down to below about 6m/s, they reflect from many materials under any angle of incidence, i.e. they behave just like ping-pong balls.
We call these “ultracold neutrons”, see https://en.wikipedia.org/wiki/Ultracold_neutrons (a little outdated unfortunately) and we can do beautiful physics experiments with those, like precisely measureing the lifetime of the neutron, or if there’s an electric dipole to the neutron.
Physics is beautiful.
Nice post, thanks
Here is a neutron source modulated by electrical current so can be turned on and off at will…
https://share-ng.sandia.gov/news/resources/news_releases/neutron_generator/
An example of a larger neutron source…
https://www.nist.gov/ncnr/about-ncnr
Great write up Maya! Thanks for including Phoenix.
To learn more about Phoenix, visit our website: https://phoenixwi.com/
Are you a publicly traded company? Interested investor here.
Phoenix LLC…
The parent company / partnership of an LLC can be publicly traded.
I thing there might be a typo in the quark notation, should udd on the right side be uud?
When I was a physics student, we had a neutron howitzer in the department. Just like it sounds — it shoots neutrons in one direction out of a barrel. No accelerator, no electricity required. No ‘OFF’ switch either… https://en.wikipedia.org/wiki/Neutron_howitzer
Though I do like the TRIGA reactor method of producing great gigawatt-size gobs of them at once, on demand.
> Though I do like the TRIGA reactor method of producing great gigawatt-size gobs of them at once, on demand.
For people wondering, they rapidly eject a control rod from the core. This produces a huge pulse of neutrons that dies out very quickly as the reactor returns to equilibrium.
A: “Should we build an off shutter to stop the alpha particles triggering the beryllium?”
B: “Nah, just point it towards biochemistry when we’re not using it.”
Not sure how you’d get a shutter in there — AFAIK the magic powder is all mixed in together.
It did have some kind of wax (or maybe polyethylene) plug, but I can’t imagine that would do anything but slow the neutrons down some. Pretty sure there wasn’t any cadmium or boron cap for it, but the thing was stored in an underground storeroom though.
Reminds me of a story from a friend at Brookhaven national labs: they built a wall with boxes of borax washing soda to act as a neutron absorber. Apparently it was the quickest and most cost-effective way to get the shielding needed.
Ah, I was thinking it was an alpha source beryllium target kind of arrangement.
And I might be mistaken yet again, but I thought dissolving the borax in water, saturated solution, worked better than the dry stuff. I am not sure it so much absorbs them as slows them the heck down, hence termed a moderator, turning fast neutrons into slow neutrons.
BTW WTH once a physics student, always a physics student.
I never get neutrons, I buy all my hack supply second hands, the ones that glow in the dark…
You can produce neutrons (and even fusion) with pyroelectric crystal : https://en.wikipedia.org/wiki/Pyroelectric_fusion
Ah, memories. When I was a kid in the 1970’s my Dad had a Californium 252 neutron source in his modest lab for doing what we would now consider primitive neutron activation analysis. It lived in a can about twice the size of a 55-gallon drum made mostly of lead, and was kept behind a boron-filled cinder block wall. I remember that it made aluminum samples profoundly, and even a bit dangerously radioactive.
Ohh, those times must have been funny XD
I’d love to see a picture of it.
Sadly, none of the film came out right.
I got mine from a stainless steel slug that contained plutonium and beryllium. It was kept in a steel container filled with paraffin. The neutrons were slowed or “thermalized” by collision with the paraffin and when slow enough, converted a hydrogen atom in the paraffin to deuterium, which gave off a gamma related to the binding energy of the deuteron.
None of this escaped the container. There were a couple “barrels” for the howitzer. These were ports that gave access to the un-thermalized neutrons. Normally full of plastic cylinders (also mostly hydrogen). We put samples inside to neutron activate them and then get a gamma ray spectrum of the isotopes. Great fun! The howitzer and a number of potent cobalt sources have been removed from the buildings in today’s hyper-aware safety enthusiasm. The pulse-height analyzer and scintillation crystals are also gone. I suppose they just mix food coloring in safe plastic micro-experiment trays now. Pretty colrs!
Yes, the beryllium-plutonium neutron source was provided to any number of colleges and universities for experimentation. We used to do a half-life experiment with a pre-1964 (90%silver) quarter in one of the barrels. The neutrons from the Pu-Be activated some of the silver, changing the 107 and 109 isotopes into 108 and 110. One of those decayed rather quickly, with a half-life of about 30 seconds. If you ran up the four staircases from the source to the classroom, you could get enough counts from the other isotope to determine the half-life, which was about 2.5 minutes. Some students who were particularly queasy about radioactivity chose to skip that lab. Of course, as the instructor, I got about a dozen times as much exposure as the nearest student would get…
I think we used indium for that. It let you accumulate a lot of pulses from the gamma. I think half-life is just under 1 hour and the sample was placed near the outer of the plastic blocks to get the right energy neutrons. We also always had Sodium 22, cobalt 60, and Cesium 136? All good for calibration. I think they all came from the reactor at the Univ. of Washington.
We uses a magnet on a string once to take the slug out and surround it with water to get rid of noise from carbon in the paraffin and measure the binding energy of deuterium. Dropped it once (pulley from ceiling worked from across the room) and someone had to make a dash and put it back in the howitzer. That is probably a report to a half dozen state and federal agencies today :-)
Nice article!
Don’t forget about spallation: https://en.wikipedia.org/wiki/Spallation, e.g. the Spallation Neutron Source at Oak Ridge National Laboratory https://neutrons.ornl.gov/sns
One of my all-time favorites is the neutron imaging footage of coffee brewing:
https://www.youtube.com/watch?v=DZrCc4fD5Qk
OK, that’s very cool. I love the contrast between water and aluminum — opposite that of X rays.
As I was watching I realized, I have the same coffee maker! Very cool to see exactly how it works.