In the 1850s British railway companies started introducing a single standard time to make their timetables consistent. Before that, every city would set its own clock based on the observation of the position of the sun. Nowadays, precise time standards are not only needed so people don’t miss their trains but also make modern communication technologies and satellite navigation work.
Generally, there are two methods of defining time, one is based on the local passage of time as measured by atomic clocks, while the other relies on the exact measurement of Earth’s rotation. The latter is not an easy exercise that involves extragalactic radio sources or huge laser-based gyroscopes. The constant survey of the Earth’s spin tells us that days are constantly getting longer, but surprisingly, severe earthquakes and weather phenomena can also take little discrete bites out of the planet’s supply of rotational kinetic energy.
How do we keep our ultra precisely measured time, the rotation of the Earth, and our position in the heavens in line?
TAI, UTC, and UT1
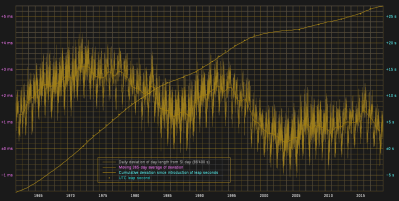
Credit: Ⅱ Ⅶ Ⅻ / Public domain
There are a confusingly large number of different time standards but the most fundamental is International Atomic Time (TAI). It is defined by the International Bureau of Weights and Measures in France and calculated from the weighted average of over 400 atomic clocks located worldwide that tick away at an incredibly constant rate.
On the other end of the spectrum is Universal Time (UT1) which in turn is purely based on Earth’s rotation.
Between these two is Coordinated Universal Time (UTC). UTC seconds are the same length as TAI seconds, but UTC is adjusted to ensure that the difference between the UTC and UT1 readings will never exceed 0.9 seconds, which is where leap seconds come in. Because Earth’s rotation is slowing down, TAI leads UTC: currently, TAI is 37 seconds ahead.
Monitoring Earth Rotation with Quasars and Laser Gyroscopes
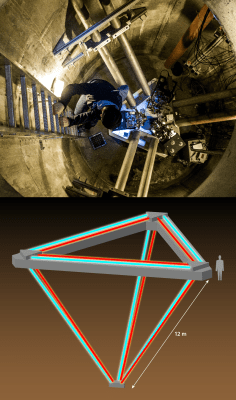
Credit: J. Igel/ETH Zurich, APS/Alan Stonebraker
If keeping a network of atomic clocks in sync is hard, measuring UT1 is even harder. The permanent monitoring of the Earth’s 3D rotation vector is the task of the International Earth Rotation and Reference Systems Service (IERS). The gold standard for this measurement is very long baseline interferometry (VLBI). VLBI measures the time differences between the arrival of microwave signals from various extragalactic radio sources (mostly quasars) with a global network of Earth-based radio telescopes. Thereby, the relative positions of the telescopes within the celestial reference frame can be determined with an accuracy of a few millimeters.
Currently, the Earth’s rotation is not monitored in real-time because there is some latency due to the required observation time for each measurement. Add, the involved telescopes can only dedicate a fraction of their up-time to the VLBI measurement. Further, the VLBI measurement technique is not directly linked to the rotational axis of Earth but needs to be calculated from the telescope positions.
There are other measurement techniques that address these problems and one of them is the recently completed laser gyroscope ROMY, located at an underground facility near Munich, Germany. ROMY consists of four 12 m long triangular ring lasers arranged as a tetrahedron. In each triangle, two separate laser beams circulate in opposite directions. A rotation introduces a frequency shift between both lasers according to the Sagnac effect and the resulting interference can be used to determine the angular velocity. From all four triangles, the full 3D rotation vector of the Earth can be reconstructed. Although the long-term stability is still not comparable to VLBI, ROMY can provide complementary real-time observations with high resolution.
Why is Earth’s Rotation Changing?
The rotation of the Earth is influenced by various factors. First of all the rotation axis changes relative to the Earth’s surface which is referred to as polar motion. The main component of polar motion is the so-called Chandler wobble. Although it was already discovered by American astronomer Seth Carlo Chandler in 1891 the exact cause of this change of Earth’s spin with a period of about 14 months is still being debated. One of the more recent publications surmises that it is dominantly excited by pressure fluctuations in the ocean.
Apart from the orientation, the velocity of Earth’s rotation also varies with other periodicities. As the tides move the mass distribution of the oceans around the solid parts of the Earth, its rotational inertia varies slightly with a period of 12 hours. There are also seasonal variations due to atmospheric circulation. Even slower variations are caused by flows in the Earth’s liquid core, and long-term climatic variations in the atmosphere.
On top of all this, Earth’s rotation is constantly slowing down due to tidal acceleration of the moon. The moon raises tides on the Earth, effectively stretching the earth a little bit. But because this water is spinning along with the Earth’s rotation, the asymmetry leads the moon’s orbit a little bit, pulling it along ever so slightly. This gravitational torque angular momentum transferred from the Earth to the Moon lengthens the day by 2.3 ms / century.
The length of a day can even be influenced by single large catastrophic events. One example is the 2004 Indian Ocean earthquake, which according to calculations by NASA scientists shortened the length of the day by three microseconds and shifted the position of the North Pole by 2.5 cm. An even more drastic example is the 1982-83 El Niño event which stretched the length of day by 0.2 ms.
With great accuracy comes great responsibility. On one hand, we can measure our position in the universe to within the breadth of a pencil eraser. On the other hand, the TAI’s averaged atomic clocks tick with an accuracy of one part in 1014. Nobody likes leap seconds, but they reflect the messy reality of living on a moving planet that’s subject to the laws of physics. It’s amazing that we can notice these tiny differences at all. Hooray for science!
Finally, an article teling about earth wobble! it was about time.
Ha time! Get it?
So we lose 2.3ms/century in spin to the moon. Does the lunar cycle gain (shorten) more than 2.3ms/century, or less? The moon is lighter, but that lever arm is enormous, so I assume it’s moment of inertia out there in it’s orbit is higher than the Earths and it’s gain in rotation about the barycenter is less than the Earth’s loss.
As the moon gets more energy, it goes to an orbit that is further away from the earth (because that has more potential energy), so it’s actually slower.
Heh, it’s slower because it’s faster.
Orbital mechanics hurts my head.
It’s easier to talk about more/less energy than it is to talk about speed.
It starts to feel genuine when you have played Kerbal Space Program for some time
From the book, Shockwave Rider”, by John Brunner ..
MIRROR, MIRROR
You are in circular orbit around a planet. You are being overtaken by another object, also in circular orbit, moving several km./sec. faster. You accelerate to try and catch up.
See you later, accelerator.
Much later.
FYI:
1. Time Standard
https://en.wikipedia.org/wiki/Time_standard
2. International Atomic Time [TAI]
https://en.wikipedia.org/wiki/International_Atomic_Time
3. Coordinated Universal Time [UTC]
https://en.wikipedia.org/wiki/Coordinated_Universal_Time
4. Universal Time [UT1]
https://en.wikipedia.org/wiki/Universal_Time
The nice thing about standards, is that there are so many to choose from!
currently UTC = TAI – 37. TAI ticks faster than UT so TAI is ahead of UTC.
Sorry, that was my fault, not Moritz’s. I even added in the clarifying “because the earth is slowing down” and then thinko-ed the conclusion.
Will fix.
https://www.nist.gov/pml/time-and-frequency-division/time-services/leap-second-and-ut1-utc-information has more detailed difference info.
Or look at the entire measured history of time difference and rotation rate of earth in plots at https://www.ucolick.org/~sla/leapsecs/dutc.html and the ongoing updates from Stephenson, Morrison, and Hohenkerk with the raw data at http://astro.ukho.gov.uk/nao/lvm/
Heck yeah! This is why I keep paying the rent on this comment section.
Just a little correction, an airliner laser ring gyro can sense earth rotation as well, as a suggestion it would be a nice article how aircraft inertial platforms are aligned prior to flight, it takes several minutes and involves sensing the local gravity force component and one of the reasons is that in this interval of time the laser ring gyros sense earth rotation and from there true heading is derived.
Correction or addition? Either way, you’re right that that would be a cool article! Even better would be a teardown… Off to check out eBay…
A teardown of laser gyroscope would be a nice addition to https://hackaday.com/2012/07/16/cracking-open-an-ancient-avionics-gyroscope/
Can they sense it in a way that gives time information, or just direction?
I don’t know how I’ll ever finish my astronomical clock design if I have to keep accounting for these ungearable phenomena!
No seriously, looking to make something on par with Jens Olson, but accounting for celestial drift in orbit periods
I’m still suspicious of the whole discrete leap seconds thing. The main purpose seems to be for civil time, not scientific stuff, a lot of that uses UT1 or TAI.
Why don’t we just switch to TAI(Or UTC but don’t add any more leap seconds) for everything internally, and display times in an idealized 24 hour exactly per solar day form?
We can convert between the two without the frequent leap seconds tables too.
If the long term trend is stable, all we need is a table of updates giving the trend over the last 25 years, the current offset at this exact moment, and the short term trend over the last year for high precision stuff.
You can update your records as often or not-often as you want, and once per decade will probably keep you within a few seconds.
Great for embedded systems, and removes ambiguity between devices that do and don’t have the latest leap second table, and it fails gracefully by getting less accurate rather than in discreet steps of 1s that are guaranteed to accumulate.
At the meetings which resulted in them the Time Lords presented reports indicating that leap seconds were fatally flawed for technical purposes. After the decision to implement them the TIme Lords in charge of operational systems announced that they would not be using leap seconds, and those systems still use TAI(-like) or UT as best suits that purpose. The original recommendation for leap seconds in broadcast time signals was accompanied by another recommendation which said that TAI was better for many applications, but that recommendation has been suppressed. Leap seconds are entirely for bureaucratic purposes required by international agreements, but discussions that led to leap seconds were held at unpublished meetings, and discussions after the decision were redacted to remove the dissatisfaction from the view of bureaucrats whose approval was needed in order for leap seconds to become the international agreement.
But think of how much it would mess up setting your sundial, in a few hundred years.
Surely they could just change the definition of the second every once in a while to match the Earth’s movements? (This year, a second is going to be 9 billion vibrations of a cesium atom, next year 9 billion and change.) Then this whole problem would go away!
The hubris of scientists to tell the rotating Earth what a second should be…
(I think I’m joking. But I’ve also got a point.)
Do big dams also increase the Earth’s moment of inertia?
They must!
Is it measurable? Cool question.
NASA estimated that the filling of the Three Gorges Dam in China would lengthen the day by 0.06 microseconds:
https://www.jpl.nasa.gov/news/news.php?feature=716
There have been discussions on how to calculate the resulting change in the Earth’s moment of inertia:
https://earthscience.stackexchange.com/questions/9978/was-the-filling-of-the-three-gorges-dams-impact-on-the-earths-rotation-rate-de
Please look at the orders of magnitude of the effects as seen in the plots of length of day https://www.ucolick.org/~sla/leapsecs/dutc.html
Every year the length of day varies by a millisecond. Every decade the length of day varies by several milliseconds. Every week the length of day varies by tens of microseconds. This is the weather in the oceans, outer core, and atmosphere. None of the earthquake or anthropogenic changes mentioned in comments here has ever been observable. A large winter storm is just barely believable as possible to observe, yet no researcher has ever said so.
Also, the Earth’s rotation changes with glacial/interglacial cycles. When the water is locked up as ice in high latitudes the Earth spins a bit faster than when the ice has melted and the water is more evenly distributed though the world’s oceans.
I can’t file time_ut1() function anywhere. Even after averaging 400+ atomic clocks, UT1 overrides it. That is the only real time for earthlings.
My favourite part of this stuff is the iteration of quality – the Very Long Baseline measurements require accurate positions. You use GPS/GNSS to get accurate positions. Then with your very accurate clocks in each location, you derive more accurate orbits and clock corrections for the GNSS satellites, which derives more accurate positions. Then you use the deformation of the earth crust and wobble to get more accurate position, and keep repeating…
Well, it’s faster (linear velocity) /and/ slower (angular velocity).
Just to be nasty and add to Elliot’s headache ;-D