After decades of nuclear fusion power being always ten years away, suddenly we are looking at a handful of endeavours striving to be the first to Q > 1, the moment when a nuclear fusion reactor will produce more power than is required to drive the fusion process in the first place. At this point the Joint European Torus (JET) reactor holds the world record with a Q of 0.67.
At the same time, a large international group is busily constructing the massive ITER tokamak test reactor in France, although it won’t begin fusion experiments until the mid-2030s. The idea is that ITER will provide the data required to construct the first DEMO reactors that might see viable commercial fusion as early as the 2040s, optimistically.
And then there’s Commonwealth Fusion Systems (CFS), a fusion energy startup. Where CFS differs is that they don’t seek to go big, but instead try to make a tokamak system that’s affordable, compact and robust. With their recent demonstration of a 20 Tesla (T) high-temperature superconducting (HTS) rare-earth barium copper oxide (ReBCO) magnet field coil, they made a big leap towards their demonstration reactor: SPARC.
A Story of Tokamaks
CFS didn’t appear out of nowhere. Their roots lie in the nuclear fusion research performed since the 1960s at MIT, when a scientist called Bruno Coppi was working on the Alcator A (Alto Campo Toro being Italian for High Field Torus) tokamak, which saw first plasma in 1972. After a brief period with a B-revision of Alcator, the Alcator C was constructed with a big power supply upgrade.
Based on the research findings, an improved Alcator DCT with superconducting field coils was proposed, but never saw the light of day due to the budget crunch for fusion research in the 1980s. Instead Alcator C was modified (hence C-Mod) as a compromise solution. Alcator C-Mod would continue to be used for fusion research at MIT’s Plasma Science and Fusion Center (PSFC) until it was shutd own in 2016.

CFS is a spin-off of MIT’s PSFC, which seeks to combine decades worth of data and lessons learned from the Alcator tokamaks with brand-new ideas and brand-new materials. Much of their business plan is contained in a paper published in 2015 in Fusion Engineering And Design, by B.N. Sorbom et al. (ArXiv preprint) titled ARC: A compact, high-field, fusion nuclear science facility and demonstration power plant with demountable magnets.
Here ARC stands for both Affordable, Robust and Compact, as well as being a nod to Howard Stark’s Arc reactor in the fictional Iron Man universe.
High-Tech and Easy to Assemble
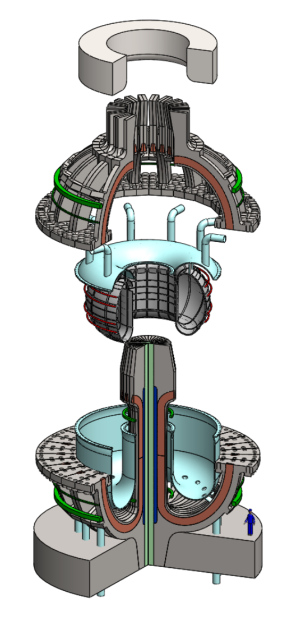
What makes the ARC reactor and the prototype SPARC reactor so fascinating compared to other tokamaks is its highly modular nature, while adding design elements that are not found in other tokamaks. The major design element that was demonstrated with the 20 T HTS magnet this month is fascinating not just because of its immense field strength for its size and power input, but also in that it can be easily disassembled.
The entire ARC reactor is built around the premise of a core vacuum vessel in which the fusion reactions take place, with this vacuum vessel surrounded by a FLiBe (Fluorine Lithium Beryllium) liquid blanket that provides neutron moderation, shielding and cooling functionality, as well as breeding the tritium that forms part of the deuterium-tritium fuel which powers the reactor.
Surrounding the vacuum vessel and containment for the FLiBe liquid are the ReBCO HTS magnets in a characteristic D-shape that was experimentally determined via the Alcator tokamaks. Yet rather than being singular magnets, they can open, to allow for the inner assembly including the vacuum vessel to be easily swapped for maintenance and for trying out different test configurations.
As also noted in the 2015 paper by B. N. Sorbom et al., these magnets are the only major risk in the entire design, in the sense that nobody had before used YBCO (yttrium barium copper oxide) HTS materials for electromagnets like this. What the recent demonstration shows, however, is that CFS now seems to have tackled this problem.
Through the use of these ReBCO-based magnets, a SPARC reactor should be able to generate immensely strong fields with very little power input. This is key to ARC’s success as the model for a potential commercial reactor and bodes well for the SPARC reactor.
Another major benefit is that the use of HTS magnets means that SPARC won’t be need the exotic and expensive cryogenic helium that cools ITER’s magnets, but can use much cheaper liquid nitrogen instead.
Next Steps
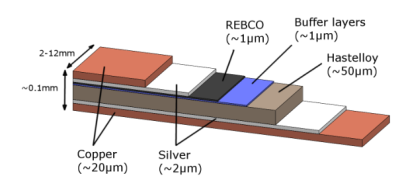
Commonwealth Fusion Systems has laid out a roadmap on its website detailing which milestones are still left to be tackled. Step one was to get the basics together using the Alcator C-Mod, as noted earlier in this article. Step two was to sort out the HTS magnets, which now appears to have been checked off the list as well. This then takes us to step three, which is the construction of a SPARC reactor.
The fourth and final step is the construction of an ARC reactor, which is labelled by CFS as the commercialization stage. What this essentially means is that with the magnet stage tackled, CFS can now move on to proving that the ARC concept works.
According to the CFS website, a site has been selected for the construction of SPARC and construction is underway. The subsequent events would be first plasma and net electricity generation, with a predicted Q > 2, much of which would depend on any potential issues found during construction and the initial testing.
An obvious item that was not covered in the original 2015 paper, and which has not since been covered in great detail to this writer’s knowledge are the divertors in SPARC (and subsequently ARC). These are devices that allow for impurities to be removed from the plasma while the reactor is active. They are essential for sustained operation of a fusion reactor.
Generally, fuel would constantly be added to the fusion reaction, while the fused helium would be removed, to ensure that the fusion reaction is not interrupted by the waste products. In Alcator C-Mod divertors were implemented along the top and bottom of the vacuum vessel, while in ITER and JET the bottom of the vessel is used for the divertor.
As divertors are the region where the plasma comes closest to the wall, so these have to be cooled. In the case of ARC and SPARC, this would have to be done using the FLiBe fluid. A major research and development question with SPARC is likely to be the exact configuration and operation of these divertors. Naturally, since rapid swapping of the vacuum vessel and surrounding structures is a feature of SPARC, it should be possible to work through a wide number of configurations in little time.
Looking Ahead
With the ARC reactor it is hoped to create a fusion reactor with a radius of 3.3 m that can achieve a Q of > 13, with a 270 MW electrical output. This compares to a major radius of 6.2 m for ITER with an on-axis magnetic field of 5.3 T, where ARC would have an on-axis magnetic field of 9.2 T. The exciting prospect with ARC is thus essentially that of achieving a similar thermal and electrical output in a much smaller reactor.
As with all such projects it’s hard to tell for sure what will work out, as demonstrated last century with the Z-pinch fusion reactors that seemed to be mere years away from commercialization in the 1950s. Even so, our knowledge of plasma physics and related fields has increased significantly since those early days. Whether ARC, another tokamak design, or perhaps a stellarator derived from the Wendelstein project will be the first to show the way to economically viable commercial nuclear fusion is still up in the air.
One thing that is however quite clear, and that is that the old adage about fusion power always being a decade away is at severe risk of being shelved for good within the coming years.
[Heading image: Conceptualized image of a SPARC reactor cross-section with humans for scale. (Credit: Commonwealth Fusion Systems)]
Looks like something Tony Stark would come up with. In a cave… with a box of scraps.
In Tony’s defense, the MCU Arc Reactor was already proven, he just had to miniaturize it. Still far more impossible than building a palm-sized internal combustion engine in a cave from scraps, but he had his hands on a working prototype prior to his capture and presumably knew the system inside-and-out.
He’s also a fictional character which helps a lot.
If you want to start making your own, here’s the spec for the tape.
https://www.superpower-inc.com/specification.aspx
Your superpower can then be having a VERY magnetic personality. 😂
I would rather invest in this than in Tesla.
I work for a similar fusion company. Would invest in Tesla to make money and use the word “donate” with regard to fusion.
Until it’s all patented and sold to the rest of the developed world for billions if not trillions. Fusion power will probably help save us against global warming, but it’s not going to mean free power. It’s simply going to mean higher profits.
Renewables are alrady pushing energy prices negative on occasion, what makes you think a surplus of energy would somehow result in a “worse” situation for consumers?
The reason why the energy prices go negative is because the consumers are paying taxes to subsidize the renewable generators, who in turn sell at negative prices to earn the subsidy because it is tied to the amount of energy produced, whether the system needs it or not.
And the consumers are never paying negative prices – they always pay positive prices PLUS the surcharges or taxes for the subsidy.
Zero point energy is by its nature counter to capitolism and profit.
Fusion or not, we still need EVs and batteries which are very real. Fusion energy would be awesome but it’s still a multi-decade gamble and we have real needs now.
After decades of fusion power being “ten years away”, now it’s only
*checks article*
twenty years away…
>A System that is sufficiently large, complex, and ambitious can reduce output far below “random” levels, since everyone’s attention is now focused on making the existing System (i.e., the wrong answer) work. Thus, a Federal Program to Conquer Cancer may tie up all the competent researchers in the field, leaving the Problem to be solved by someone else
– John Gall, The Systems Bible
This is a great quote! Thanks for sharing! :D
Another said he would make America grate again. He´s now ground up.
For the curious:
https://www.quora.com/Did-Joe-Biden-really-say-he-will-cure-cancer-if-he-gets-elected
Hey look! Someone dragging unrelated politics into an otherwise normal discussion! Surprise!
Hey, SimCity 2000 had us developing fusion power by 2050, so by those standards we’re right on schedule!
Personally I think it’s more likely we are on schedule for what was predicted in the Universe 25 experiment.
Possibly but if we have fusion power then there is a real chance of being able to terraform Mars and Venus as well as putting the possibility of interstellar travel within reach in the next few hundred years.
A fusion reactor has now been created at Lawrence Livermore with a positive output using lasers and a bb sized pellet of dutirium and tritium. FYI.
Yeah, uhm, kinda.
442 MJ input electrical energy yielded a 1.3 MJ thermal output from the target.
True, the actual energy absorbed by the target in the laser pulse was slightly less than the output, so it qualifies as “fusion happened”, but they have a long, long way to go to make this method even close to net energy positive.
And to be clear: This is fine — LLNL’s National Ignitiion Facility was never intended to actually be net power positive — it’s a research facility.
But to draw from this that “Commercial fusion power is near” is still a real stretch.
We’ve been doing thermonuclear fusion for almost 70 years now, since Ivy Mike. We have *extremely* strong incentive to make it work, and have spent many thousands of humans lifetimes in the effort.
We continue to make tiny, incremental, but exceedingly costly steps toward the goal of cheap fusion power. At what point do we decide the effort just costs too much?
Pft, 70 years is too long? It took humanity a few hundred thousand years to tame fire, then a few tens of thousands more to matter agriculture. Big problems only get solved with big picture thinking and patience.
NIF isn’t just a research facility. It’s a nuclear weapons research facility.
https://lasers.llnl.gov/science/nif-and-stockpile-stewardship
And that’s fine. Studying plasma and other material at high temperature and pressure conditions is still useful. It’s important, though, to not overstate the goals and achievements of the project.
Damn -this comment beat me to it! (However I think it does imply that fusion might always be less than a decade away)
i figure the number used is the time it takes to design, fund, build, and test a new tokamak facility. the current estimate is that we will need at least 2 more before we can build a power plant actual (with the first one of those being the 3rd). i suspect this will be sufficient considering where we are at as far as q is concerned and how far we have come in the last 50 years. at 10 years a piece (and iter has taken a lot longer than that), we will have fusion by the mid 21st century at an optimistic minimum, and around the turn of the 22nd century as the pessimistic maximum, or it gets shelved because were all dead.
This is the first time I’ve heard of fusion always being 10 years away. The number I heard when I was young was perpetually 50 years. More recently I always heard perpetually 30 years away. Seems the closer we get, the number of years it is perpetually away seems to shrink so as to minimize the apparent consequence of the advances bringing it closer.
10 years is still in the territory where old professors can retire and young researchers can find a different job or topic if their efforts and promises don’t pan out, so they can just throw the number out there. 10,20,30,50, years is the same.
When the schedule gets to single digit years, that means you have a working theory and a real roadmap, not just “let’s do something and hope we’ll figure out what we’re supposed to be doing in the mean while.”
Does it matter? Nobody should ever expect fusion to be commercially viable. Forget about it. It is not going to happen. Still, it worth funding this research, because a lot of sweet sweet side effect technologies are produced in the process. So even if we’ll keep funding the impossible fusion efforts for hundreds of years, it’ll be totally worth it.
So meaningless. Every moment a fusion reactor operates, it does so at a deficit Now the deficit will not be as large and they will call it progress in order to get more funding. Fusion research is about selling snakeoil not commercializing fusion. The sun shines because of QT, and that is never going to be done in a controlled manner.
I mean, they used to say the same of fission, and before that petroleum, and before that electricity itself.
—-
So meaningless. Every moment those neutrons collide, it does so at a deficit. Now the deficit will not be as large and they will call it progress in order to get more funding. Fission research is about selling snakeoil not commercializing fission. The sun shines because of nuclear reactions, and that is never going to be done in a controlled manner.
So meaningless. Every moment those refiners operate, it does so at a deficit. Now the deficit will not be as large and they will call it progress in order to get more funding. Petroleum research is about selling snakeoil not commercializing oil. The sun shines with the heat of a thousand flames, and that is never going to be done in a controlled manner.
So meaningless. Every moment that dynamo turns, it does so at a deficit. Now the deficit will not be as large and they will call it progress in order to get more funding. Electricity research is about selling snakeoil not commercializing electricity. The sun shines because of ether energy, and that is never going to be done in a controlled manner.
So meaningless. Every moment those sticks rub together, it does so at a deficit. Now the deficit will not be as large and they will call it progress in order to get more meat. Fire research is about selling snakeoil not making fire. The sun shines because of fire, and that is never going to be done in a controlled manner.
—-
Please stop using electricity. It’s not as effective as the uncontrolled fusion of a star and therefore meaningless, by your assertion.
Epic comment. Naysayers gonna neigh.
+1
We need to stop thinking about fusion as investing in power generation. Think of it as investing in high energy plasma physics research and plasma engineering/technology. These pose advanced and difficult engineering challenges which could produce a lot of good engineering knowledge even if fusion never materializes. The journalists are at fault for continuing to push the fusion power dream.
Fusion may look good on the outside but there are a lot to it that articles on it fail to mention. It has more problems with radioactivity than fission, consumes a good chunk of power that it produces, and uses a rarer form of hydrogen (tritium) that needs to be produced from nuclear fusion.
This article is long but it points out the problems with fusion..
https://thebulletin.org/2017/04/fusion-reactors-not-what-theyre-cracked-up-to-be/
The radioactivity and tritium breeding are pretty much the entire point of the ITER project. ITER’s gotten tons of backlash for being so big and expensive and “taking so long” but the entire point of it is to build a reactor that’s *guaranteed* to work so that you have enough data to actually confirm you can manage those things.
All of the problems you’re thinking of are totally solvable, and there are plenty of designs to solve them. You just can’t demonstrate them until you have a reactor that’s actually fusing atoms.
> to build a reactor that’s *guaranteed* to work
The problem is that while ITER’s fusion gain is calculated and hoped to be 10, the actual “engineering Q” of the facility is still less than 1 because the whole system consumes more energy when it’s running than the reactor can put out, even if we count the raw 500 MW thermal output and ignore efficiency.
If you want to fuse atoms and make a bunch of plasma and throw neutrons around to test materials and structures, you can do that with various types of fusors and particle accelerators. We already have reactors that actually fuse atoms – just at a terrible efficiency. We didn’t need to build ITER for that – it’s main point is an exercise in plasma control using a design we already knew is terrible for it – the other contenders like the W7-X have already leapfrogged over ITER in that respect, and are on a quicker path to further iterations and first ignition with a tiny fraction of the budget.
The reason why ITER keeps going is because it would be a massive egg on the face for the whole field if we admitted that it’s just a boondoggle.There are too many people with too much reputation to keep invested in it, so what’s another 100 billion between old friends?
Besides, supposing ITER did manage to do what it promises; if you’re going to wait for ITER to prove the concepts that the other reactors and projects would need in the next 10-20 years, it’s still going to be too little and too late. These other projects that are supposed to benefit from ITER’s funding through technology trickle-down actually need to develop and test their own right now, or twiddle their thumbs and waste money waiting on ITER.
Again: the design is there to generate *fusion* at appropriate plasma parameters. The energy balance overall doesn’t matter. That you know will scale when you make it bigger.
The bigger problems are the material handling and breeder blankets. No one’s ever done that. It simply hasn’t been done. That doesn’t mean you need to do it for all these designs: stellerators, compact high field designs, etc. They all get the same benefit from the breeder blanket/materials research.
It’s like building a reference design. It probably isn’t optimal, but you know it’ll *work* and you can work out the parts you don’t know with it.
As I said, we’re all waiting for the “reference design” to go anywhere.
The stellarator is already built, it proved its point, and its aiming for 30 minute plasma with break-even conditions this year. Are we going to then wait until 2035 or 2045 or 2055 when the ITER actually manages to fire up and produce the plasma conditions that they’re supposed to test?
No. Either the funding and the focus has to shift dramatically, or it would be painfully obvious that the entire field is stalling deliberately just to build ITER.
> you know will scale when you make it bigger.
Thing is, the main drawback of the tokamak design is that the heating plasma current is itself the source of the instabilities that prevent it from working properly, so when you increase power it becomes less stable. The system has been described as, “trying to contain jell-o by wrapping it with rubber bands.”.
There is actually no guarantee that the ITER design will scale up.
“The stellarator is already built, it proved its point, and its aiming for 30 minute plasma with break-even conditions this year. ”
It’s not a fusion-burning plasma. The device isn’t physically designed for that. You can’t just shove deuterium/tritium into some open hole on it and get fusion.
“Are we going to then wait until 2035 or 2045 or 2055 when the ITER actually manages to fire up and produce the plasma conditions that they’re supposed to test?”
Things happen in parallel. If there’s a machine capable of fusion burn before ITER (the tokamak) is available, then ITER (the programs) will divert to that one. There are already tests going on at JET, for instance.
“There is actually no guarantee that the ITER design will scale up.”
ITER (the tokamak) doesn’t need to scale up. It’s not a reactor design. Even if you never actually complete ITER (the tokamak), ITER (the program) is still needed, now. If there’s a better design for DEMO, you pivot to it.
Again: just to be extremely, extremely clear. W7-X cannot be a fusion demonstration facility. It is a *plasma* demonstration facility. In order to have a “stellarator” version of ITER, a *new* design would need to be designed and built. And if you believe that can happen sooner than ITER (the tokamak) is completed, I respectfully disagree.
Solar may look good on the outside but there are a lot to it that articles on it fail to mention. It has more problems with pollution and slave labor than fusion, consumes a good chunk of the power that it absorbs from the sun as heat, and uses a rarer form of silica (crystalline silicon) that needs to be produced via electric heating.
All technologies have downsides, especially technologies that HAVEN’T BEEN DEVELOPED YET. Look at any modern technology when it was in its infancy and your arguments will apply. Solar power, fission, petroleum, computers, electricity…
If you’re only interested in technologies that were 100% perfect the first time anyone tried, you’re going to need to give up pretty much all modern technology and live in a cave. Remember, early fires were probably pretty inefficient too.
Curious how this compares to this: https://www.tokamakenergy.co.uk/tokamak-energy-exceeds-target-of-20-tesla-with-hts-magnets/
The major difference is that the CFS magnet is significantly larger and has a large bore (on the scale of what will be required for SPARC). An average person could easily fit through the opening in the CFS magnet. The structural forces are way more intense when you increase the bore size so you have to account for those factors.
This article has some images that show the scale: https://www.cnbc.com/amp/2021/09/08/fusion-gets-closer-with-successful-test-of-new-kind-of-magnet.html
An important detail, rarely appreciated, is the mechanical force from these enormous magnetic fields.
A 20 Tesla magnet has an internal pressure of 40 MPa (6000 psi). The windings and their support structure are literally a pressure vessel that must withstand that internal pressure.
Can you imagine an air tank that large, containing 6000 psi? Terrifying.
And, just like an air tank with that much pressure in it, there is a tremendous amount of stored energy that would be released if there is a rupture in the containment: equivalent to many kg of TNT, easily enough to level a good-sized building.
Why is 6000 psi so terrifying?
There are plenty of 6000 psi pressure vessels available. This would also be the water depth equivalent of slight under 14000feet or 4225meters), quite a few people dove to and over that depth in pressure resistant vessels.
Sure, there is risk involved, and you wouldn’t want to take any unnecessary risks, but it’s looks more like a solved problem than something to be terrified of.
I own a tank for a paintball gun that is filled to 3000psi. SCUBA tanks are normally filled at 2400-3500psi. I have seen hydraulic systems operating above 10000psi… Chamber pressures for many firearms exceed 30000psi – the tiny .22LR generates 25000psi, a relatively humble 9mm round generates 35000psi. I use baling wire that has a tensile strength of 110000psi, and I have seen it fail.
So no, 6000psi does not cause me to mindlessly run away in terror. Your estimate of the energy potential of one of these is greatly exaggerated.
…estimate of the energy potential of one of these is greatly exaggerated.
10 cubic meters at 40 MPa is 400 MJ, or about 100 kg of TNT (or ANFO, if you prefer). A heck of a lot more than the puny 600 J or so from a 9mm. That’s less than a studio photoflash.
There’s very little stored energy in a (essentially incompressible) hydraulic system. Not analogous at all. Apples & oranges.
I’ve seen the aftereffects of a SCUBA tank rupture, and that’s a thousand times less energy than this thing. Still terrifying. But I still strapped one on and used it, many times, and respected its capacity to kill me instantly if I was stupid about it.
Sure, it’s just engineering. The fact that we can make fertilizer at scale (the Haber process) proves we can handle large pressure vessels like this, and have for a long time. But they made a lot of people dead to get to that point though. It’s worthy of healthy respect both to the physics, but also the engineering involved.
40 MPa is nothing if the structure is solid material, like a tank that is drawn into shape without seams. It starts to be a problem when you have welded seams and varying loads, because you have to consider each weld as a compromise in the structure with random voids and slag inclusions, crystal structure defects, chemical changes to the alloy…
It’s been a long while since I had to calculate welds, but as a some sort of rule-of-thumb, you start by halving the strength of your (regular) steel, from say 355 to 178 MPa to get below the fatigue limit, then you halve that to get the average permissible load, and then you halve that to account for the imperfections in welding so you don’t have to use some Very Special Technique that cost millions and only one guy in the world has the qualifications to weld it – and whoops now you’re actually at 45 MPa. You’d think using some UHS steel gets you away from that, but it actually doesn’t because it’s even more difficult to weld without spoiling the microstructure that makes it ultra-high tensile strength in the first place.
In other words, never stress your welds if at all possible. They’re surprisingly unreliable.
And whatever you do, don’t heat them up to fusion-plasma temperatures if they’re nearby supercooled superconducting magnets. Think of the thermal stress…
230MW of electrical output? What aspect of fusion actually generates usable electricity? My understanding is that this will be, like fission reactors, just a novel heat source for a steam engine. Granted, like fission reactors, a novel heat source with a more efficient, cleaner fuel cycle… but still part of a steam engine. Measuring its output in “megawatts of electricity” isn’t really representative of the “work” the reactor is doing, or its current state… which is why fission reactor power is measured in Watts(heat)… what’s done with that thermal energy can vary wildly.
Unless I am missing something fundamental in the function of these devices?
You’re not wrong. Like virtually every other common form of electricity generation fusion reactors will capture the heat through exchangers and use that heat to produce steam which will turn turbines.
The good news is that those turbines are getting more and more efficient every year, and we’re currently in the ballpark of 50% during average runtime, depending on a number of factors. The better news is that maximum turbine efficiency goes up with insulation materials and new turbine materials and shape, both of which are advancing too. The best part is that turbines can get better efficiency when engineered for a specific, steady input and a stable, sustained fusion reaction would provide one of the few sources of heat more constant than nuclear fission. It’s not 100% efficient, so a lot of that energy produced will be lost, yes. Of course, give it a few decades and we’ll be capturing that thermal energy more efficiently.
It worries me how much heat we are dumping into the atmosphere that wouldn’t naturally be there. Fusion, fission, gas, coal, fuel etc. all put extra heat into the atmosphere. I can’t see this included in calculations about global warming but it must also play a role in warming. Fussion power would still have this issue albeit without creating greenhouse gasses. From a rough calc I think it is globally 380 trillion watts per day thermal currently.
Other than instantly discrediting your post by using a nonsense unit “watts per day”, it’s an interesting point.
Humans use total of about 500-600 exajoules per year (according to a couple of random google hits). Or an average of 17-20 terawatts, 2.5 kilowatts per person. Which is astonishingly low, actually, when you consider how much energy food production takes. (oh, so 17 TW is ~400 TWh/d, which is likely where the nonsensical “380 trillion watts per day” comes from)
Sunlight hitting earth’s 140 M sq km disk, at 1.4 GW per square km, deposits 190 000 TW, ten thousand times what the puny humans can muster (so far).
No, in terms of just thermal load, humans can’t make a difference. I’m much more concerned about all the other crap we’re doing to the biosphere.
I had calculated it in Mbtu based on the co2 produced from oil, coal and gas then added in the thermal heat output of all fission reactors. I messed up with the conversion to watts should be 380TWh/d. I wondered how much of the energy from the sun hitting the earth ends up as heat. Some is reflected back, some is used by plants etc. I agree its small but still not helping.
Earth’s average albedo is about about 0.30
albedo is about about 0.30…
Yeah. And likely to go up if we evaporate much more ocean.
So it reduces’ humans’ contribution to one part in 3000. If that heat gain is compensated by simple radiation (4th-power law), that means a steady-state temperature increase average of 0.03 C.
Of course, human population density is rather lumpy, so the “heat island” effect is quite real in dense cities: Human heat input, still only a few percent of solar insolation, can lead to a temperature gain of several degrees.
This tech, and the even more compact aneutronic forms such as one project in Australia, is the only genuinely viable long term form of energy for running a civilisation, particularly one that wishes to expand into space and onto other planets. Every other option is going to either run out or is vulnerable to the sort of chaos the universe is want to throw at large delicate structures. People seem to overlook the fact that sustainable means, forever.
Nothing is sustainable forever without outside material or energy sources. Nuclear power of both sorts can theoretically go a very very very long time on the mass of suitable fuels you have on hand, but the sun driven renewable will all last till the sun actually dies or the later phases of its life destroys the natural processes that we are extracting the energy from…
There is nothing about expanding into space that makes ‘renewables’ impossible either, its all about managing power requirements to how far you are from the energy source/ your catching area… Certainly nuclear is more compact, and remains just as practical much further away from a star, but you could use other sources if you design for it and manage it appropriately. Even in the vast void between stars its technically possible to be solar powered, just rather hard to do needing a much larger structure – but that doesn’t necessarily mean its any more delicate – poke a hole in the collection mirror for you solar array, you still have a mirror, in one panel you still have many others etc – some repairs restore full function, but poke a hole in the smaller key areas of any power generator and you kill it outright – in the case of nuclear thats basically hit the reactor at all its dead, hit the steam turbine end its probably repairable before lack of power kills you…
https://lppfusion.com for shoe-string budget fusion — and because I have a capacitive discharge welder…
Before getting too enthousiastic about claimed Q factors, see this video: https://www.youtube.com/watch?v=LJ4W1g-6JiY