All nuclear fission power reactors run on fuel containing uranium and other isotopes, but fueling a nuclear reactor is a lot more complicated than driving up to them with a dump truck filled with uranium ore and filling ‘er up. Although nuclear fission is simple enough that it can occur without human intervention as happened for example at the Oklo natural fission reactors, within a commercial reactor the goal is to create a nuclear chain reaction that targets a high burn-up (fission rate), with an as constant as possible release of energy.
Each different fission reactor design makes a number of assumptions about the fuel rods that are inserted into it. These assumptions can be about the enrichment ratio of the fissile isotopes like U-235, the density of individual fuel pellets, the spacing between the fuel rods containing these pellets, the configuration of said fuel rods along with any control, moderator and other elements. and so on.
Today’s light water reactors, heavy water reactors, fast neutron reactors, high temperature reactors and kin all have their own fuel preferences as a result, with high-assay low-enriched (HALEU) fuel being the new hot thing for new reactor designs. Let’s take a look at what goes into these fuel recipes.
Gathering Ingredients
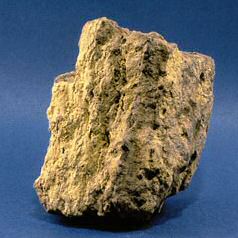
The raw ingredients are usually mined from the ground, with the mining of uranium from seawater a relatively new development. The countries with the most uranium ore available for extraction are Australia (28%), Kazakhstan (15%) and Canada (9%), with Canada having the highest-grade uranium ore. Generally, the product from uranium mines is U3O8, which contains about 85% uranium. The next step depends on whether the natural uranium has to be enriched, meaning the amount of fissile (U-235) material increased from the naturally occurring level.
Once at a fuel manufacturing plant the original natural uranium will be in one of two forms, either uranium hexafluoride (UF6) or uranium trioxide (UO3), with the former being the case when enrichment has taken place. Before this can be turned into fuel pellets, this form will need to be converted to uranium dioxide (UO2). These pellets are what is usually referred to as ceramic fuel pellets, which contrasts with the more rarely used metallic fuel types (e.g. ZrU) that saw use in some reactors until the 1980s. A major advantage of UO2 ceramic pellets is the high melting point of 2,865°C, which is a good property for the high-temperature environment of a nuclear fission reactor.
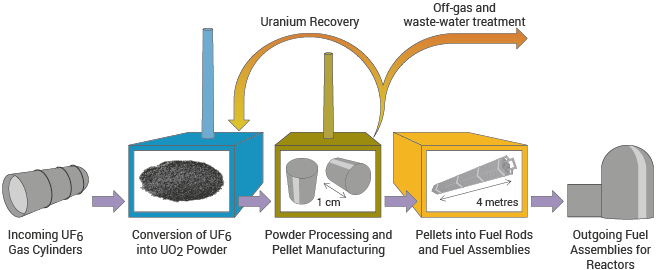
The ceramic fuel pellets are produced under several hundred MPa of pressure, after which they are sintered at 1,750°C under an oxygen-free atmosphere (usually argon-hydrogen). The sintered pellets are then machined as a final step. This gets them to the exact dimensions required to fit into the fuel rods, with any removed material returned to an earlier part of the pellet manufacturing process. For most reactor types these pellets are roughly 1 cm in both diameter and length.
An interesting addition to some pellets can be a burnable neutron absorber such as gadolinium (in oxide form), that is added to remove neutrons early on in the fuel cycle, thus reducing reactivity and allowing for longer fuel life. More commonly, zirconium diboride is added as a burnable absorber in the form of a thin coating on pellets. This is used in most US reactors, including the AP1000 type (and derivatives) that is also used in China.
Packing It Up
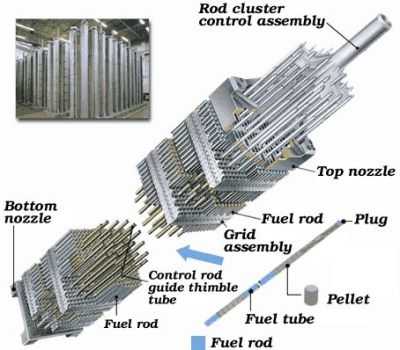
The fuel rods themselves are generally made out of a zirconium alloy, which has the beneficial properties of having a high melting point, high resistance to chemical corrosion, vibrations and impacts. These alloys are also essentially transparent to neutrons, meaning that they do not interfere in any noticeable way in the reactor’s nuclear fission chain reaction. These fuel rods contain a large number of individual ceramic fuel pellets, which after filling is flushed and ultimately filled with pressurized helium gas at a few MPa. Some space remains between the end caps and fuel pellets that’s usually filled with a spring that provides compression to the stack of pellets.
These individual rods are then fixed in a steel framework, which allows for individual rod types (fuel, moderator, empty slots) to be controlled as required by the target reactor. For an average pressurized water reactor (PWR) type reactor, these fuel assemblies are between 4 m and 5 m tall and 20 cm across. Nearly 200 of these assemblies will go into a 1 GWe PWR, containing around 18 million pellets. While Western fuel assemblies tend to be rectangular, Russian PWR (VVER) reactors use hexagonal fuel assemblies. This does not affect the basic operation, however.
When a PWR is refueled, only about a third or a quarter of the fuel assemblies are removed and replaced with fresh assemblies, with the rest being rearranged to optimize the reactor operation and total burn-up of both the old and new fuel assemblies.
The second most popular type of fission reactor is the boiling water reactor (BWR) design, which as the name suggests directly boils water to create steam, instead of using a pressurized primary loop as with PWR designs. Although BWRs cannot reach the same level of efficiency as PWRs, they are much better at load-following operations, which is due to the water being led in channels through the fuel assembly. As the water both moderates and cools, varying the flowrate changes the energy output.
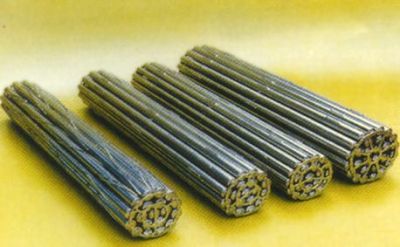
In addition to this, BWR fuel assemblies have a number of other notable differences from PWR fuel assemblies, but none as drastic as with the fuel assemblies for the Canadian CANDU pressurized heavy water reactor (PHWR). This type of fission reactor doesn’t use light water (H2O), but rather heavy water (D2O) that has heavier deuterium instead of hydrogen atoms. This difference enables PHWRs to use unenriched uranium fuel, which are assembled in short bundles that are loaded into fuel channels in the reactor. This loading and unloading can be done while the reactor is running at full power, with unloading happening at the other end of the fuel channel. This means that a PHWR does not have to shut down for refueling or reshuffling of the fuel.
PHWRs are the most flexible fission reactors currently in common use, able to use unenriched, natural uranium, enriched uranium, mixed oxide and other fuels like thorium. They have some overlap with the Soviet RBMK design that can also run on unenriched uranium fuel due to its use of graphite as a moderator, but PHWRs are significantly more versatile.
Exotic Taste
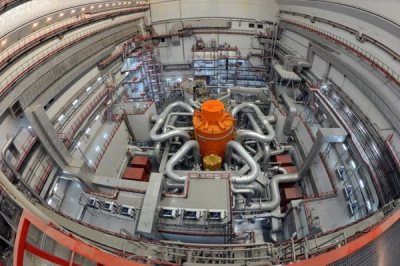
Since virtually all fission reactors in the world today are either a light water reactor (LWR) like the common PWRs and BWRs, or a PHWR (e.g. most of Canada’s reactors), most nuclear fuel produced is some kind of PWR, BWR or PHWR fuel, but there are more exotic reactors that have very different fuel demands yet again. One of these are the fast neutron reactors (FNR), which demand yet another fueling strategy. FNRs generally use the concept of ‘seed’ and ‘blanket’ regions, with the seed regions having high reactivity and neutron production. Neutrons from the seed regions are then captured by the fertile isotopes in the blanket regions, turning them fissile.
FNR fuel is where the once-through uranium fuel cycle of mining-fission-disposal really breaks down, with LWR spent fuel containing significant amounts of fertile isotopes that can be turned into fissile fuel for use by the FNR as well as fresh LWR fuel. This overlaps somewhat with the traditional reprocessing of spent LWR fuel that removes the uranium and plutonium, allowing for this to be used in mixed oxide (MOX) fuel. MOX fuel is distinct again from REMIX fuel that mixed reprocessed spent fuel with low-enriched uranium.
What is a common factor among all of these fuel types, however, is that they target reactors that run at fairly mundane temperatures. Most recent development has focused on high temperature fuels, for use in high temperature reactors that operate at 750 °C to 950 °C with helium as coolant. The Chinese HTR-PM reactor and US-based X-energy’s Xe-100 design use spherical fuel elements in a pebble bed configuration, which results in a fuel loading and unloading procedure closer to that of PHWRs. China’s aim is to use the so far successful HTR-PM design in an HTR-PM600 configuration — three 200 MW reactors combined — that can replace boilers at coal plants, which will massively increase demand for this fuel type if successful.
HALEU
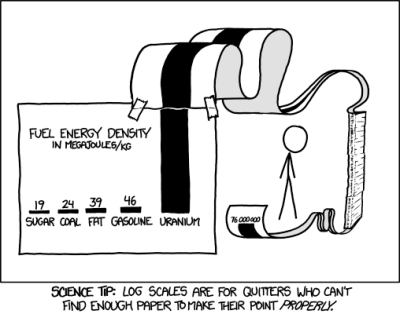
Observant readers may have noticed that over the past years there have been a lot of US-based nuclear power startups that have announced new reactor designs. Many of these require so-called high-assay low-enriched uranium (HALEU) fuel. While the US’s current fleet of fission reactors run on fuel that is enriched up to 5%, HALEU is enriched to between 5 and 20%, which enables more compact designs, higher efficiency and much more time between refueling. This compares to the small reactors in US submarines that run on >90% U-235 high-enriched fuel, granting them their 20-25 year refueling time. Above 20% U-235 is considered to be ‘high-enriched’ (HEU).
Since enriching uranium requires a certain level of production capacity that currently does not exist yet, there is a bit of a catch-up going on in the industrial industry. This has led to for example TerraPower announcing a delay in the start-up of their first Natrium SMR. Previously Russia had been a major supplier of HALEU, but since the war in Ukraine, the reliance has now shifted to the US’s domestic production capacity that is in the process of being rapidly expanded.
Geopolitics aside, what should be clear at this point is the immense variety in nuclear fuel types and their applications, with the new Generation IV reactors adding to this. With an increasing demand for reliable, low-carbon power, it would seem the game is on to extract as much energy as possible from each gram of uranium mined.
Please pardon the hijack but as almost every state has several plants it’s madness to keep burying this deadly stuff in NM or where ever-AND making more of it, instead of serious international efforts towards finding ways to re-use it.
It’s not really madness. It should be reprocessed as much as possible, but we could dispose all of our nuclear waste in a very tiny area buried very deep and all the hullabaloo about something happening to it or somebody in the Dune-scale future digging it up is FUD and nonsense.
Right now we are dumping our waste in our air. If we don’t fix that, there won’t be people in the Dune-scale future to find our tiny football-field plot of waste miles under New Mexico. And renewables—I’m not going to be diplomatic about this any longer—renewables are BULLSHIT. They’re fake. They’re a money-making scam and they’re wasting our time, which we have just about run out of.
But yes reprocess as much as possible. There is a concern that shipping it around all over the place for that will lead to more accidents than simply burying it all at a centralized site, but you know. It’s an engineering problem.
When a renewable creates more energy than what was taken to manufacture and install it, than it makes sense.
For example, I see your bullshit, and I raise you biogas.
Even when renewable produces more energy than needed to manufacture it, it can still be bad. For instance, with EV solar we’re hitting the point where there is too much electricity produced on sunny days, and spot price drops to negative amounts.
In some places there’s already too much wind power generation. One place is the Orkney Islands. They have wind turbines all over the place but the main power connection to the main UK islands is far too small to handle transmitting it all. Nobody in charge of that will put up the money and navigate the politics required to install the massive upgrade that connection needs.
The location is a place that makes sense for wind power because it’s very rare the Orkney’s don’t have enough wind to make power. It just needs a new and much larger “extension cord”.
We’ve got a working solution already, it’s orders of magnitude safer than anything else, and it’s only being passed over because the profit margins aren’t high enough. The scare tactics about nuclear is a fig leaf. If you think biogas will scale appropriately (without polluting the environment greatly) you’re dreamin’
One place biogas makes sense is tapping the methane from waste dumps. Once a section of a dump is large enough and gets capped, it will in a couple of years be producing enough methane from decomposition to be able to power engines to drive generators. How long that will last depends on how large each waste cell is and the overall mix of organic matter it contains.
Burning the methane for power generation is better than simply letting it vent or flaring it off to lose its energy uselessly.
What would also make sense is mining waste dump cells after they’re no longer producing a usable amount of methane. Tons of metals get thrown away. It would be better to recover those already refined metals from a dump than to mine more unrefined ores.
Better yet would be fully automated waste separating and recycling plants to recover all the metals and fuel or refinery feedstock useful chemicals before they go to a dump. All the required technology for this already exists and has existed since the late 1980’s, but nobody has bothered to put all of it into one plant. All of the waste sorting and recycling plants (some also burn waste for power generating) rely heavily on manual labor at some point.
When it is possible to build a plant that can take in raw sewage and solid wastes at one end and output electricity, clean water, metals, and a varying supply of chemicals suitable for various re-uses, why doesn’t it get done?
Reprocessing in the U.S. is prohibited and the reason we have add much nuclear waste as we do is simply politics. France reprocesses much of the world’s spent fuel at La Hague and has made it quite profitable while extracting the usable uranium and plutonium for MOX and other reactor types. The U.S. doesn’t even export spent fuel for others to reprocess, rather we just leave it for future generations to deal with.
2/3rds of the existing waste in the US is from the weapons program, and it’s in a form which is not easily processed or it has already been “processed” by mixing it with various other junk. What France is doing is just processing the spent fuel rods, not old jumpsuits spilled over with plutonium.
True, bit of we could start reprocessing our spent fuel, at least we could limit what we’re adding to the existing waste. What’s already there will likely never be properly disposed of.
Still waiting for the Yucca Mountain repository. It’s only been in the works since 1987
As of this writing and current government, Yucca mtn is being decommissioned 《again》
The government actually collected something like 29 billion dollars in taxes out of the nuclear industry specifically to fund the repositories, but refuses to spend any of it.
At the risk of being redundantly redundant, I’d heard that the Yucca project was abandoned when they realized that people would eat anything in a hotdog… OK, you”d heard that before – I’m old…
One part of processing high-level radioactive nuclear waste, is that it typically needs to be stored under water for about 30 years. The long-lived isotopes like will be much less intense (large half-life), than that of a short-lived isotope (small half-life). That is also true for the time before KBS-3 storage ( https://en.wikipedia.org/wiki/KBS-3 ) – “The waste is first stored in intermediate storage for 30 years.”
I always think of the line from Blade Runner: “The light that burns twice as bright burns half as long and you have burned so very very brightly” while thinking about Europium-155 (~5 years half-life), Krypton-85(~11), Cadmium-113m(~14), Strontium-90(~29) and Caesium-137(~30 years half-life).
I suspect that there is lots of potential energy that could be captured while this is happening over those 30 years, that currently is not. A supercritical carbon dioxide turbine in theory could be used to extract power from any heat source that is above it’s critical temperature (304.128 K, 30.9780 °C, 87.7604 °F). The lower the hot end temperature the less efficient it would be, but if it is waste energy does it matter ?
In a way, nuclear waste is a problem that only gets easier with time – at least in processing terms, even if the quantity builds up. Accident risk in temporary storage is pretty manageable.
We could bury it on the moon….. What could possibly go wrong?
We decided that was not a good idea 24 years ago.
Well nuclear waste seem a good research and development material for solar cell and photoelectric generators and solid state power generation (nuclear batteries in simple man English)
With a nuclear battery, you instead measure nominal/max average power output, while the charge is based on half life of nuclear material used, would never need to recharge them, cuz the battery life would probably out last you and your children
Take a nuclear battery and a super capacitor bank
And probably could use it to power almost anything
I mean it would maek sense to use nuclear waste because ionization radiation carries much more energy per photon than normal solar cell which typically convert infrared light and some visible light into electrical potential, using a solar cell designed for alpha, beta, and gamma radiation would be smaller and much more efficient because nuclear physics and quantum mechanics
In abstraction, our solution to current fossil fuel crisis is to stop using it. There is no serious or even semi-serious attempt mitigate the damage other than “use less going forward” and certainly about zero attempt to actively undo the damage (massive scale CO2 sequestration, etc). From that point of view, nuclear tech and disposal of the waste is already much, much better. Even if it means a hole in the ground in New Mexico. Yeah, it is a kick-the-can argument but hopefully the next major innovation in energy will be even better and learn from the fossil fuel then nuclear cycle. Who knows, huge space mirrors or something.
We don’t have the engineering competence for huge space mirrors anymore. People don’t like to talk about it, but we were better engineers in the 60s and 70s. We can’t do what they did anymore.
And that would be WAY more dangerous than simply using the nuclear we’ve got already.
That’s how you troll hackaday…
Good job TG!
Is reduced engineering competence the reason that the launch cost per kg in orbit has fallen by about an order of magnitude since the 1960s? Is it why the number of satellites in orbit is increasing so rapidly?
Not so much to do with engineering competence. It’s all about the capital. Convince enough starry-eyed people with lots of free cash to hand it over, and you can make and break a lot of things quickly, and get things *done*. 8.6 billion dollars of capitalization and another 6 billion-ish of fees buys you a lot of hardware and engineering time.
God bless rampant late-stage capitalism.
Yep. People forget that before you can call some venture cheap, it first has to pay back its investment. If you build a billion dollar factory to build one ten dollar hammer, that hammer actually costs you a billion dollars, plus ten.
If a company like SpaceX doesn’t have a sustainable business model that can pay back the money the private investors and the government have put into it, they haven’t actually managed to make rockets any cheaper – they just found some other schmuck to pay the difference.
Besides, since SpaceX is not a publicly traded company, they don’t have to tell you their real costs. Whatever cost estimates or prices you get from Elon Musk are simply whatever he wants to say – it has nothing to do with the actual cost to orbit for customers like NASA.
There have been studies into using boreholes in NM for civilian waste but it hasn’t led to anything yet. The nuclear waste currently being buried in NM is all from military uses in a geologically stable salt formation.
“China’s aim is to use the so far successful HTR-PM design in an HTR-PM600 configuration — three 200 Watt reactors combined” …… just what I was thinking to use to run my laptop and a few LED bulbs in my house.
Left out a mega or a kilo? Ha. Then again there’s RTGs like that, such as the strontium-powered ones for Russian lighthouses. But they aren’t talking about those.
Ah ha. Anybody got a spare chunk of strontium lying about in their junk pile? I’ll look on Ebay.
Like Nuclear Fusion, Nuclear Fission creates new heat energy on our athmosphere, which is a problem, just like releasing stored energy from hydrocarbons in the current massive scale. To achieve a future on a livable planet, for producing electricity, it would be best to use only renewable energy sources like geothermal energy, wind, Solar and water power. These power sources all have solar power as their original source and thus do not contribute more to heating our planet
I heard a podcast recently that was making noises about being able to make geothermal practical in virtually any location. I couldn’t help but think if geothermal became our primary energy source, how long would it be before we became alarmed about pulling too much heat out of the earth’s core/mantle/magma/whatever.
(It was an interesting concept they were cooking up: drilling with microwave energy to turn the rock to ash…working in the lab…you’ve heard the story before)
Earth captures 173PW of solar energy* on average. A few hundred GW of released potential energy from nuclear reactors is a fart in the wind in comparison.
(* https://en.wikipedia.org/wiki/Earth%27s_energy_budget )
Unfortunate analogy. It’s the farts and Vo2 which are the problem because of their greenhouse effect.
As you say, releasing a bit of heat isn’t the issue.
Are you one of TG’s alts?
Anyhow, also good trolling. I actually believe you are this stupid. TG not so much.
Sorry, no idea who or what TG is… here is an explanation by Stefan Ramstorf, one of the most cited climate scientists: https://twitter.com/rahmstorf/status/1603448183333568516
If the current Trend of heat production caused by human activities continues, within 100 years, this heat production alone will have heated the planet additional 0.2°C. It’s quite unlikely that the trend will continue like this, but if we had feasible Nuclear Fusion by that time and would use it excessively it might be a problem, depending on the scale. While renewables only use the already available power that is turned to heat eventually anyways
Geothermal also heats the atmosphere. You are moving heat from under the earth’s surface to the atmosphere. In fact Geothermal heat is produced by nuclear reactions.
Nice overview on manufacturing uranium based nuclear fuel and the various commercial power generation systems that currently produce large amounts of electricity for public use. I’d mention that power utilities are constantly shuffling the cores for maximum output and the amount of energy produced is much lower than what’s available from a given assembly which is why we have so much partially spent fuel in storage.
Gen V reactors e.g. MSR will happily eat that ‘spent’ nuclear fuel. Getting it out of the ceramic first though, that’s a hassle.
There are a couple of proposed fast-spectrum MSR designs that basically burn up their own actinide ‘wastes’, which are the ones with pesky-length half lives (~1000 years)
The fission products still need to be removed, but with a liquid fuel that’s possible (and an integral part of the design). The ‘chemical kidney’ is one of the main challenges
In no way, a hack.
Lol now I’ll try to be a troll.
It’s hack-a-day, so considering several articles a day are published and at least one of them is a hack, they are fulfilling their obligation. Maybe you’re looking for all hacks R’us. It’s next to hack hack hack. Or MaryAnne’s hacks. Down in the hacking district.
yea, its only a hack if something goes terribly wrong (points at chernobyl). then its hacks all day every day.
Well the Japanese had a criticality accident when they were mixing up fuel in buckets.
I’d give them at least amateur hacking status for that
Uranium Tailings Ponds: https://www.youtube.com/watch?v=MJ6667Noex0&t=1444s
Uranium Processing with plenty of Nitric Acid: https://www.youtube.com/watch?v=VOm7_Lf8PVo
Preparing Plutonium, the most poisonous thing on the planet: https://www.youtube.com/watch?v=tBlqv2AcpQI
SL-1 Accident: https://www.youtube.com/watch?v=AWi_w2H1saE
SRE Accident: https://www.youtube.com/watch?v=cgawvTu9agA
Mild Radioactive Waste Disposal: https://www.youtube.com/watch?v=HUHuX-Gbenc
Excellent article very informative, easy to understand and accurate. However you forgot to refer to TRISO which is also a very important type nuclear fuel.
Generation IV reactors
https://en.wikipedia.org/wiki/Generation_IV_reactor
The GEN IV Forum reframes the reactor safety paradigm from accepting that nuclear accidents can occur and should be mastered to eliminating the possibility of an accident. Active and passive safety systems would be at least as effective as those of Generation III systems and render the most severe accidents physically impossible.
Relative to Gen II-III, Gen IV reactors include:
Nuclear waste that remains radioactive for a few centuries instead of millennia
100–300x energy yield from the same amount of nuclear fuel
Broader range of fuels, including unencapsulated raw fuels (non-pebble MSR, LFTR).
Potential to burn existing nuclear waste and produce electricity: a closed fuel cycle.
Improved safety via features such as ambient pressure operation, automatic passive reactor shutdown, and alternate coolants.
Wen u realize uranium-235 and plutonium-239 aren’t the only heavy metals that can fissile, Lotta different lanthanides and other actinides that can work in a nuke just as good as u-235 or stankmetalshit-239
If it blows up in a nuke, it should boot up a power reactor just fine….
Some of em are probably way more easier to either mine than u-235 or mass produce in a reactor, like dat stankmetalshit-239 (p-u Dat shit stink)