We’ve had nuclear fission reactors in operation all over the world for ages, but nuclear fusion always seems to be a decade or two away. While one cannot predict when we’ll reach the goal of sustained nuclear fusion, the cutting edge in test hardware is advancing at a rapid pace that makes us optimistic. Beginning as soon as this month and extending over a few years, we’re living through a very exciting time for nuclear fusion and plasma physics.
The Mega Ampere Spherical Tokamak (MAST) got a big upgrade to test a new cooled divertor design. JET (Joint European Torus) will be testing the deuterium-tritium fuel mixture that will be powering the ITER (the research project whose name began as an acronym for International Thermonuclear Experimental Reactor but has since been changed to just ITER). And the Wendelstein 7-X stellarator is coming back online with upgraded cooled divertors by next year.
Here the MAST Upgrade’s Super-X divertors have so far shown a ten-fold decrease in the temperature which the divertor is exposed to while carrying thermal energy out of the tokamak reactor. This means a divertor design and ultimately a fusion reactor that will last longer between maintenance sessions. On the stellarator side of things, Wendelstein 7-X’s new divertors may allow it to demonstrate the first continuous operation of a stellarator fusion reactor. Meanwhile, JET’s fuel experiments should allow us to test the deuterium-tritium fuel while ITER is working towards first plasma by 2025.
Nuclear Physics on Hard Mode
The reason why we figured out how to use nuclear fission in a commercial setting so quickly can be essentially summarized as it being a process that easily happens by itself. The difficulty with nuclear fission is more with controlling the nuclear chain reaction, requiring a balance between neutron moderation and absorption in the case of thermal neutrons and the common light water reactors (LWRs).
On the other hand, nuclear fusion is a completely different game. Instead of using some of the heaviest isotopes around and lobbing some neutrons at them to convert a part of them to energy, nuclear fusion uses the lightest isotopes (hydrogen isotopes) possible and attempts to fuse them. This is obviously not a process that easily happens by itself, unless you happen to take a very large amount of hydrogen and allow immense gravitational pressure to kickstart the fusion process, as is the case in stars.
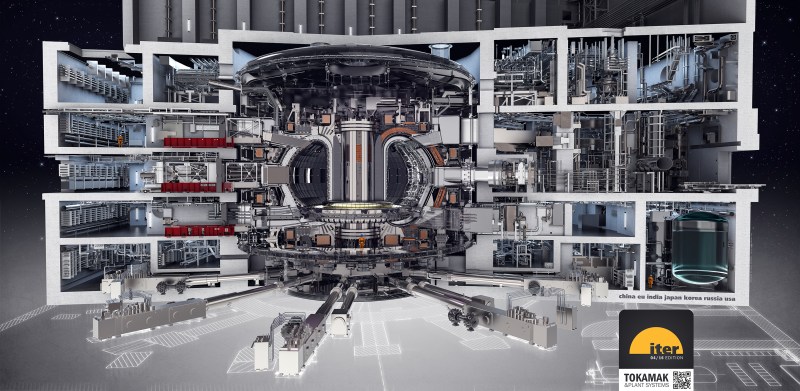
While on Earth we can find the remnants of natural fission reactors today from a time when there was more natural uranium-235 around, fusion has obviously remained limited to stars, including our Sun. As a result, the only way that we are going to accomplish nuclear fusion in a sustained reaction on Earth is by creating the right pressure, temperature, and other conditions that will allow hydrogen isotopes to conquer their fear of the Coulomb barrier and fuse so that they can release a lot of energy in the process.
This is in short the reason why it has taken over a hundred years now since the first discovery of the nuclear fusion process. A hundred years of careful experimentation and the use of new materials and analytical technologies to improve our understanding of high-temperature plasma physics and our ability to create and sustain the conditions for fusion in such a contained plasma.
Going for Break-Even
An essential metric in determining how well a nuclear fusion reactor design works is through the Q-factor. This is the ratio between input and output energy. In order for a fusion reactor to start operating, it has to literally warm up which costs energy. Sustaining these operating temperatures of 100 million degrees Celsius or more requires a certain energy input; preventing the cooling of the plasma is one area of the research.
Ideally the reaction becomes self-sustaining, in which the fusion reaction provides the energy required for sustaining the plasma temperature and fusion reaction. Yet even with some input energy required, the Q factor must be over 1 to result in any actual energy output. This basically means more energy output than what is required by the reactor’s magnetic field generator and other elements.
With next month’s JET deuterium-tritium fuel experiments the goal is to see whether the energy output can be boosted through these fuel mixtures. Currently JET has achieved a Q of 0.67 which it hopes to improve on. It’s projected that ITER’s successor (DEMO) would need to hit a Q of at least 25 to make the economic case for fusion reactors. ITER is slated to hit a Q of 10.
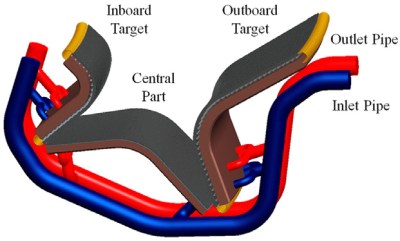
The Super-X divertors that are being tested by MAST Upgrade could be helpful here with improving efficiency of the fusion reaction. One of the purposes of these divertors is to purify the plasma by removing unwanted elements, such as the helium atoms that are produced as a result of the fusion process. Keeping the plasma stable and pure are two essential factors in making a fusion reactor work efficiently.
Even so, the magnetic fields in these tokamak designs that contain the plasma continue to form a source of constant headaches, as the plasma isn’t content to keep moving in simple, predictable patterns. Between kink instability and banana orbits (neoclassical transport) the plasma tends to show sudden movements away from the center of the magnetic field. Effectively dealing with this is still a major concern, also because it can result in fuel being lost as it escapes the magnetic field.
This is one area where the stellarator design may have a leg up on the tokamak design.
Cool Flow
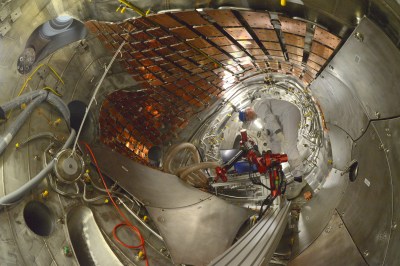
Compared to the simple, round tokamak design, stellarators seem quaint at the best of times, but this is a result of these fusion reactors following the plasma behavior instead of seeking to control it. The almost organic-looking magnetic coil configuration is the result of modeling of the plasma to find a configuration which essentially works with the super-heated plasma instead of against it.
As a modern stellarator design, the Wendelstein 7-X (W7-X) incorporates all of the know-how about these complex devices. Since first plasma in 2015, W7-X’s performance has been essentially flawless, so that in 2018 the next round of upgrades began. This involves among other changes the installation of the water-cooled divertors.
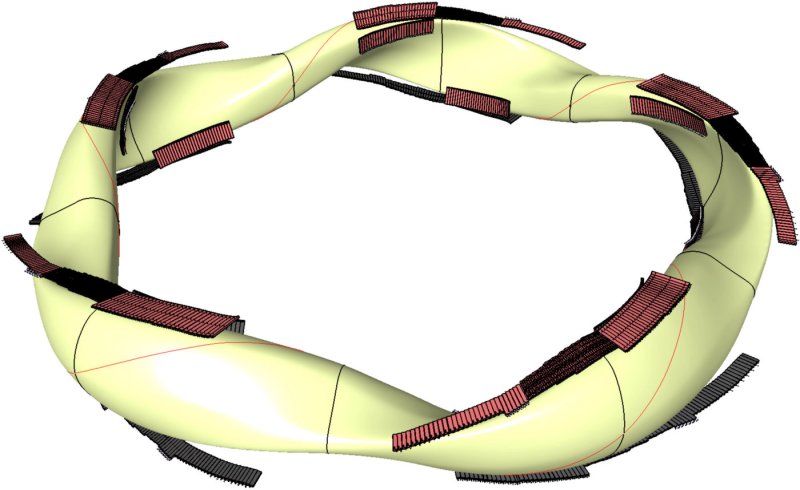
With the uncooled divertors operational time was limited, but the goal with the new and improved W7-X is to perform a continuous run for up to 30 minutes. Considering that for tokamak designs a run-time for more than a minute is considered a pretty good length, this would be a major achievement.
Although the W7-X is not intended to produce more power than is put into it, it serves the important function of demonstrating that the stellarator fusion reactor design is at least as capable as the tokamak design. This makes the next series of experiments rather exciting. Unfortunately, due to the SARS-CoV-2 pandemic that has kept the world occupied for over a year now, the W7-X upgrades have been delayed, so that the resumption of experiments likely won’t take place until next year.
A Lot to Look Forward To
Much about nuclear fusion reactors has been an intense and rather unforgiving course in plasma physics and other elementary areas of physics. When the optimistic assumption that was made in the 1950s about nuclear fusion being ‘only a few years away’ was snuffed out harshly when Z-pinch designs proved to be unworkable, the field seemed to fade away in the public’s opinion for many years.
Fast-forward countless scientific papers over many decades later and it appears that we may now cautiously consider ourselves to be on the cusp of truly workable nuclear fusion reactors. While it’s not quite a ‘within the next few years’ kind of deal, ITER and related tokamaks such as China’s CFETR give us hope for tokamak-style reactors. Meanwhile, W7-X’s progress forms an alternate approach that may work as well, worse or better than tokamaks.
With ITER’s deuterium-tritium fuel plasma still at least fourteen years away, we have a lot to look forward to with these current tokamak experiments at the JET and MAST laboratories. When we get the first experimental results from W7-X in the coming years, perhaps they will be promising enough to kickstart a stellarator version of ITER. Whatever the case may be, this decade looks to be one in which nuclear fusion is slowly shuffling back into the limelight.
“fusion has obviously remained limited to stars”
Err, and hydrogen bombs?
Not for harvest-able energy that CONTRIBUTES to humanity……
Wait till your first big near-earth asteroid gets a bit too close for comfort….
harvestable no but
https://interestingengineering.com/soviet-engineers-detonated-a-nuke-miles-underground-to-put-out-a-gas-well-fire
putting out a giant gas fire I would catagorize as “contributes to humanity”
That probably was not fusion though.
I think Kuwait was pleased that the oil contractors scaled back to 55 gallon drums full of dynamite.
“CONTRIBUTES to humanity”
Like preventing a world war for 76 years and counting? That’s just MAD, no?
Ok, how about “sustainable fusion” remains limited to stars, so far.
“sustainable fusion”, if you consider billions of years “sustainable”.
B^)
It’ll keep you warm until you come up with something better.
and 14 year old kids who read about David Hahn and still thought it was a good idea.
It’s important to note that W7-X and MAST are purely plasma facilities – there’s no actual fusion involved. Which means no radiation, no neutrons, etc. The JET D-T tests are actually fusion tests.
There’s this weird idea that somehow facilities like ITER and W7-X are somehow competing, which is nuts. Part of the reason why ITER’s so huge is that it’s *not* being designed as a plasma facility. It’s being designed as a *fusion* facility, which means all of the “we’ll figure it out later” details that get skipped over finally need to be worked out. In other words, ITER in some sense is more of a radioactive materials research facility than fusion plant.
Just as a single example, for instance, the development of a workable tritium breeder blanket is something that’s essential to any fusion reactor and we currently have never done it – there’s not enough tritium in the world right now to run a commercial reactor (of *any* design) for more than a few months.
None of these facilities are really competing at all. They’re just targeting different problems in developing a fusion reactor, which is *way* more than just plasma physics.
Better confinement is substantial for prolonged operation. But touché.
Yes, but the benefits of continuous operation are pointless until you get to the DEMO stage. Because you can’t generate power because you don’t have the fuel. Literally. The entire world’s supply of tritium is measured in small numbers of killograms, and a DEMO-scale plant (of any type) would use a good fraction of a kilogram *per day*.
There are plenty of other technologies that don’t care about the plasma configuration, too. Which is why you have a bunch of different facilities, all testing different aspects.
Yes, but why does it make sense to build tokamaks if a stellarator works so much better? It’s like testing different grades of gasoline or different parts in an engine that fundamentally refuses to run more than three revolutions because it has no flywheel.
ITER was conceived in 1985 as a political project between US/EU/Japan and the USSR and didn’t even enter its design phase until the 90’s while the predecessor to the W7-X, the Wendelstein 7-AS had already proved the concept and the stability of stellarators in 1988. It was already known way back that this design is going to be the worse if ever works at all – it’s really just a cold-war boondoggle that they keep going just because they’ve gone so far already.
The main problem they’re targeting is how to get continued funding – whether that results in anything useful in terms of fusion power.
Too cynical.
ITER was designed to work to get a Qfusion > 1 (around 8 is the design point). That meant it had to use a conservative design to ensure it got there. The guaranteed approach is just to increase the linear dimensions over and above the JET forerunner project.
Sure, new commercial projects are happy to take risks by trying to take shortcuts to save money.
But probably no more than 10% of them will work, and possibly none of them at all. ITER is the backstop. It will demonstrate a working experimental fusion process (but no electricity generation). Then comes more hard work to enhance that design to become a working demo power plant, with electricity generation. E.g. The DEMO successor to ITER.
Where possible, the design concepts for ITER have been retrofitted into the existing JET project, to provide early practical validation.
Your comments about funding are wide of the mark. A lot of the funding is provided as designed and built components from the countries participating. That slows things down, but ensures all funding countries develop relevant expertise in the technology.
When you compare it with other long-term big science projects, ITER isn’t that bad.
The LIGO gravitational wave principle first had practical devices in the 1960s, so it took a good 50 years before the first breakthrough observations were made.
The Higgs boson was postulated in 1964, and it took 40 years of different experimental devices before the Higgs was finally identified in the LHC in 2012.
If all this stuff was easy, it would have been done long ago. It isn’t. You have to keep at it and keep going. The countries of the world understand that, so were prepared to fund ITER. We know it is going to meet its targets but will take time. The only question is whether another commercial or research approach manages to beat it and shave a decade or so off fusion development.
Besides, ITER is planned for its first actual D-T plasma in 2035, which is still 14 years away. Only then can they begin testing the neutron shields and tritium breeding for real – if the timetable holds.
The W7-X was built between 2002-2014 or 12 years with a total funding of only €1.06 billion. ITER will cost over 20 times as much and counting. All the indicators are that the Wendelstein team will simply leapfrog over ITER because they’re soon at a stage where they might as well build another stellarator to test the concepts that ITER was supposed to test, and do it faster and cheaper.
Yes, I’m sure the W7-X team will just randomly branch out into tritium breeder blanket, ECRH, diverter, and plenty of other design aspects all on their own! That makes *total* sense.
It’s not a freaking race. W7-X is at the Planck institute, which is part of the overall European fusion research community anyway.
“ITER will cost over 20 times as much and counting.”
You’re confusing the cost to *build the device* with the *cost to run the program*. Suppose they build ITER, and W7-X demonstrates continuous burn with no problems. Now they propose building a larger stellerator, which completes just shortly after ITER starts D-T burn.
Does that mean ITER’s funding was wasted? God no, because half that freaking research goes *directly into* the other device’s construction in the first place.
Again, you could put ALL that funding into the other device’s construction in the first place. Why build the tokamak when you know it’s not gonna work well?
>You’re confusing the cost to *build the device* with the *cost to run the program*
Not really. “The total investment for the stellarator itself over 1997–2014 amounted to €370 million, while the total cost for the IPP site in Greifswald including investment plus operating costs (personnel and material resources) amounted to €1.06 billion for that 18-year period.”
“Why build the tokamak when you know it’s not gonna work well?”
It’s going to work *fine* for ITER’s main purpose, which fundamentally is figuring out the *fusion* portion, not the *plasma* portion!
ITER isn’t intended to produce power because, again, we *literally do not have the capability to do that right now*. If W7-X suddenly comes out with a press release and says “ha, fooled you, we’ve actually got continuous plasma operation right now, we’re good to go!” it would *still* be 10+ years before you could even *design* a power-producing reactor, because we do *not* have the fuel, and we do *not* have the materials radiation experience.
Tokamaks also have significant advantages for developing breeder blankets, for instance, because the physical geometry is better. Stellarators have physical constraints which make breeder blanket design more complicated. In other words, building a stellarator to test breeder blanket design would be nuts: it’d be like trying to design an engine already inside a car, rather than working on it at a testbench.
And then even at the DEMO stage it might not make sense to go with a stellarator because the main goal of DEMO is not to be a competitive power plant – it’s to figure out the logistics issues. Great, your breeder blanket generates tritium! Fantastic. How do you figure out when to shut the reactor down to remove them? How long does it take to extract the tritium and refurbish the blankets? We don’t know *any* of that yet.
It’s great that stellarators look promising for more practical power plant design. Awesome. But again, just go read the W7-X team’s 2017 paper on steps to a prototype (HELIAS) reactor. It’s *flat out* stated, *right* there. Explicitly. Let me quote it for you.
“A final decision on the most suitable blanket concept for a HELIAS power plant should only be made after detailed numerical investigations (next section) and experimental tests as e.g. foreseen with the ITER Test Blanket Modules.”
Is that clear enough? What else do you want? The part where they say “However, many of these challenges will be addressed in the ITER and W7-X era within the next two decades.”
This. Is. Not. A. Race. ITER and W7-X are both part of a *single* EUROfusion roadmap. Seriously. Go read it. You can download it. Search for “stellarator.” It’s all over it. Let me quote from *it* as well.
“In the long run, it is expected that this strategy, together with the technology results from a tokamak DEMO and developments of plasma exhaust and plasma facing components from Mission 2, could allow a stellarator fusion power plant to be built.”
And you completely misunderstood my comment regarding funding: I meant you’re confusing the cost for building *ITER* with the cost for running the program. ITER’s a different research platform than W7-X is. You can’t compare the two like you’re doing.
Aside from ending designs that’ll make fission look like cave man work. This may end up as something only nation states can afford, in the mean time the march to solve our energy demands continues on in other ways. At least we’ll know a lot more about plasma physics.
There are half a dozen different compact fusion approaches that are being investigated in smaller projects and one or more of them may well beat the big designs in the commercial fusion for electricity production race. Some are so light and compact that they would be practical to ship into orbit and off to mars or the moon, as well as drive the transport routes between Earth and those locations. At some point it may even be easier to just build huge reactors in orbit and bean the power down to the surface with maser arrays.
Including high-quality attributions, for making the comment more educational, would be nice.
Making you work out how google works would be even more educational. LOL
“There are half a dozen different compact fusion approaches that are being investigated in smaller projects and one or more of them may well beat the big designs in the commercial fusion for electricity production race.”
No, they won’t. They might beat the big design to, say, a Q=10 or 50 plasma threshold, but not to the electricity production race, because all of them need the research *from* the big designs to actually commercially produce electricity.
Now, you might ask, “well, if that’s true why are there commercial fusion startups?” Because if they can demonstrate a cheap, easy to build, patented high-Q plasma design, *their* reactors will have a significant advantage in the marketplace. The materials issues are a mostly independent problem from the plasma design. The reason there are commercial fusion startups is that they can see there’s an “end of the line” ahead where fusion plants *are* viable.
It’s like testing new train engine designs while the railroad’s being planned. You still have to build the railroad, figure out the logistics, etc. but you don’t wait to figure out the best design until the railroad’s done.
The big designs are big because they’re guaranteed to work for the research goals, not because they’re the right design for a commercial plant.
ITER was designed before magnets got a lot better, ITER is redundant.
ITER isn’t designed to be a *good* fusion system. It’s designed to *be* one. Yes, better HTS-based magnets might make a simpler/smaller system (as with SPARC), but it carries significantly more risk and it wasn’t an option at the time.
You’re conflating ITER the actual building/thing with ITER the research program. Just because we might, for instance, be able to build a SPARC-based design that would hit equivalent plasma Q values in less time doesn’t mean we would’ve been able to do ITER’s research program with a SPARC-based design in the same time. Plasma research isn’t the same thing as fusion research.
ITER is the testbed for DEMO, my point is that even DEMO is redundant. Honestly I would not be surprised if it never gets built.
You may find this interesting, https://www.nanalyze.com/2021/06/when-nuclear-fusion-energy/
I’m glad to see the on-going development of fusion research and infrastructure. That said, I suspect we’ll be 15 years from sustained breakeven reaction for further decades, until a nation or two decides they REALLY have to make it happen.
“I suspect we’ll be 15 years from sustained breakeven”
Nah, practical fusion power generation is always “just 20 years away” and has been for a lot longer than 20 years.
4th generation, walk away safe fission reactors, please, and maybe even LFTRs which do better than our current antiques that “burn” <1% or their fuel and leave the rest as very long half-life, highly radioactive transuranic elements.
Even without breakeven, perhaps unsustained, upheld fusion might be used as neutron source for inducing fission in nucleii of otherwise stable, abundant isotopes of heavy elements? Thus we would have process which wanes down if you emergency shut the fusion part down, like removing the spark from the plugs, instead of going into meltdown. No chain reaction, no local circularity of causation, system controls the circuit breaker all the time.
if commercial fusion happens in my lifetime I’ll be surprised. All the above is just piecemeal (though essential) research, and really just shows how far we have to go.. Yes, we are closer to commercial fusion than we are to time travel, FTL travel, or antigravity, but that isn’t saying much…
What I hope we are closer to (though it isn’t looking likely now) is substantialy better ‘battery’ tech…
“Yes, we are closer to commercial fusion than we are to time travel, FTL travel, or antigravity, but that isn’t saying much…”
Okay, *that’s* a bit much. We can’t even *imagine* any of the latter three being scientifically possible (and if you think any of the Alcubierre drive/wormhole papers disagree with that you need to read them closer), whereas the commercial fusion issues are more logistical than theoretical.
As in I can layout a research roadmap right now to a commercial fusion plant, but any of the three scifi crap you listed would have 100 steps of Underpants Gnomes-style ??? along the way.
I don’t disagree with your skepticism but commercial fusion clearly *can* happen, whereas it’s not clear any of the other things you listed can.
A research roadmap? Impressive…yawn…
These projects are only created to keep some people off the street, otherwise they may get bad ideas. As long as I live, I hear and read about it, but haven’t seen no steady and usable fusion at all. I will be dead when a drop of water will come out of it.
Maybe not off the streets but fusion reactors do generate pensions and kickbacks for politicians.