Many years ago, I read an article about the new hotness: lithium batteries. The author opened with what he no doubt thought was a clever pop culture reference by saying that the mere mention of lithium would “strike fear in the hearts of Klingons.” It was a weak reference to the fictional “dilithium crystals” of Star Trek fame, and even then I found it a bit cheesy, but I guess he had to lead with something.
Decades later, a deeper understanding of the lore makes it clear that a Klingon’s only fear is death with dishonor, but there is a species here on earth that lives in dread of lithium: CEOs of electric vehicle manufacturing concerns. For them, it’s not the presence of lithium that strikes fear, but the relative absence of it; while it’s the 25th most abundant element in the Earth’s crust, and gigatons are dissolved into the oceans of the world, lithium is very reactive and thus tends to be diffuse, making it difficult to obtain concentrated in the quantities their businesses depend on.
As the electric vehicle and renewable energy markets continue to grow, the need for lithium to manufacture batteries will grow with it, potentially to the point where demand outstrips the mining industry’s production capability. To understand how that imbalance may be possible, we’ll take a look at how lithium is currently mined, as well as examine some new mining techniques that may help fill the coming lithium gap.
A Rocky Start
Although lithium has been known and well-characterized by chemists since the early 1800s, it was only in the middle of the previous century that commercial uses for lithium compounds were identified. The aircraft industry’s demand for stable lubricants resulted in the development of greases made from lithium soaps, and the need for high-performance but lightweight metals led the aluminum industry to employ lithium to improve the Hall-Héroult smelting process. Around the same time, doctors discovered that lithium salts can treat patients with bipolar disorder.
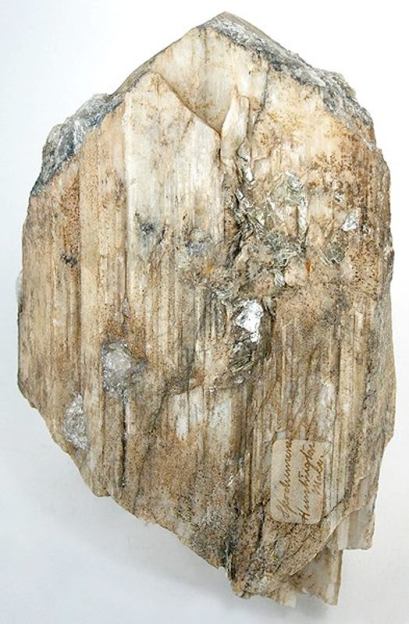
Even with the additional demand of the nascent nuclear industry starting in the 1940s, pretty much all the lithium needed could be supplied from small hard-rock mining operations that exploited deposits of rocks containing large crystals of lithium minerals, like spodumene, petalite, and lepidolite. These three minerals remain in high demand to this day for the production of lithium hydroxide, one of the two main lithium compounds used by industry.
The production of lithium from hard rock mines has a lot in common with other mining and refining methods we’ve discussed in this series. Ore-bearing rocks are blasted out of open-pit mines, scooped up by gigantic loaders, and trucked to a refining plant. There, the rock is reduced in size by a series of crushers and mills until it becomes a fine powder. Water is added to the powder to create a slurry known as pulp, which also contains surfactants and dispersants that make the lithium-containing minerals hydrophobic. In a shallow tank with air pumped through from the bottom, the light lithium forms a froth that floats to the top while the heavier rock particles sink.
After the lithium froth is skimmed off the flotation tank, the extra liquid is filtered off to create a concentrated but impure lithium powder that needs to be refined. The refining process depends a lot on the source minerals and desired end product, but for concentrated spodumene ore, lithium is typically leached out using a combination of sulfuric acid and sodium hydroxide. While this is a direct route with high yields, the acids and bases involved can make it environmentally problematic. Other acid-free leaching processes have been developed as a result, which is said to be the kind of process Tesla is using in their new lithium hydroxide plant being built next to their Texas Gigafactory.
Down in the Brine Mine
As mentioned before, seawater contains something like 230 billion tonnes of lithium, dissolved mainly as lithium salts. While this constitutes the bulk of the lithium on the planet, it’s far too diffuse — a mere 25 micromolar — to serve as a viable commercial source without vast expenditures of energy to extract and concentrate it. But seawater isn’t the only brine that contains lithium, and extracting the valuable metal from underground brines has become the main production method since the 1990s.
By far the biggest lithium-bearing brines are found in the “Lithium Triangle” of South America. Occupying parts of Chile, Bolivia, and Argentina, the area is home to large salt flats or salars, areas where ancient lakes or ponds evaporated, leaving behind salts and other precipitated minerals. These salt flats have built up over millions of years, leaving rich layers of minerals beneath their surfaces. And as we’ll see, the flat terrain and harsh arid conditions on the surface also play a part in the mining process.
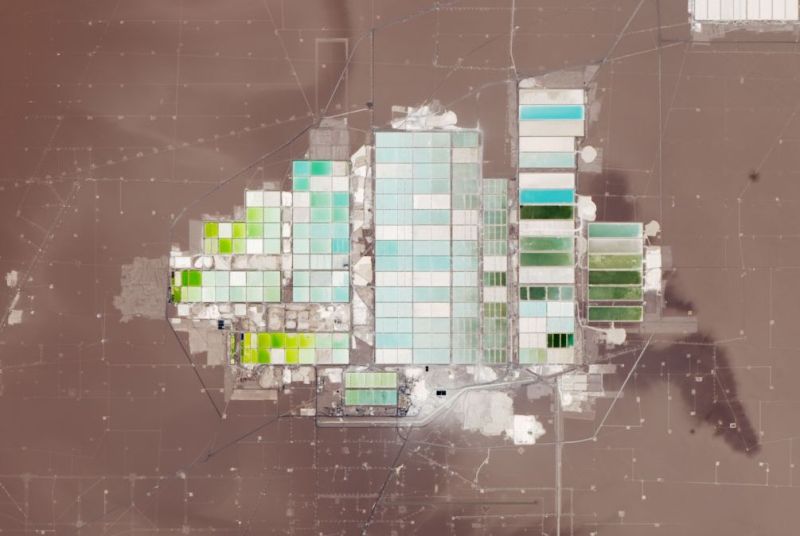
Mining lithium brine is quite unlike any of the other methods of mining we’ve covered before, and couldn’t be simpler. Instead of digging up rocks and painstakingly isolating the material of interest, brine mining consists of injecting water down into salt deposits through deep boreholes. The water dissolves the salt deposits, creating a rich brine that can be pumped up to the surface. The brine is pumped into shallow ponds and is left in the sun to evaporate.
When most of the water in a pond has evaporated — up to two years later — the now concentrated brine is harvested. The concentrate contains a variety of elements in addition to lithium, including sodium, magnesium, phosphates, and boron. The concentrate can either be further processed on-site, or as is becoming increasingly common, shipped via pipelines to ports for transport to lithium processing plants abroad.
On the face of it, the evaporation method for lithium brine mining seems like a winner. It’s super simple, it’s powered almost exclusively by the sun, and it’s devoid of some of the impacts that a large open-pit mining operation can have. But there are still huge problems with evaporation concentration. First off, it requires vast amounts of water to create the brines in the first place, and because evaporation ponds are only practical in places where it doesn’t rain much, water is already in short supply. The water used for brine mining is also lost to the atmosphere, coming back to the surface somewhere far from the evaporation ponds. Plus, the evaporation ponds occupy unbelievably large amounts of land — some pond complexes cover an area the size of Manhattan — which makes it difficult to scale up operations. And the amount of time it takes the sun to do its work is a problem in terms of production flexibility.
A Better Way
To make the most of brine mining while mitigating its shortcomings, direct lithium extraction methods are becoming increasingly popular. In DLE, brine is pumped from underground sources, but instead of concentrating the brine by open evaporation, lithium is removed from the brine using a number of chemical and physical methods. One method is ion-exchange adsorption, where the brine is mixed with an absorbent material that preferentially binds lithium compounds over the other compounds in the brine. One class of sorbents used in DLE is known as layered double hydroxides (LDH), materials with a layered structure that allows lithium chloride in the brine to fit between the layers while excluding the potassium, magnesium, and other salts. The brine is returned to the ground, while the high-purity lithium chloride is washed off the sorbent.
Other DLE methods include membrane-separation technologies like reverse osmosis, where the brine is pumped at high pressure through membranes with pores that retain the lithium salts, or by solvent extraction, where organic solvents are used to extract the lithium. The common theme with DLE methods, though, is the fact that they are closed-loop processes — the water used to create the brine is returned to the underground formations containing the lithium. DLE plants also take up a fraction of the physical space that even a single evaporation pond would take, and they don’t rely on extreme environments like salars to work.
Best of Both Worlds
As attractive as DLE technology is, at the scale needed to be commercially viable, DLE plants still require a fair amount of energy to run. But in some places, a quirk of geology has left ample lithium deposits near a source of abundant renewable energy. In the Imperial Valley of California lies the Salton Sea, an inland saline lake that lies atop a series of active geological faults, including the famous San Andreas fault. The area is perfect for geothermal electricity production, with eleven plants currently producing 2,250 MW. Some of these geothermal plants are co-located with DLE plants, which pump up hot, lithium-rich brines that are purified using the geothermal energy produced on-site. Environmentally speaking, such plants are about as low-impact as lithium production can be, with the geothermal DLE plant being built by Australian company Controlled Thermal Resources predicted to produce 68,000 tonnes of battery-grade lithium by 2027.
With the demand for lithium set to soar, the ability to extract what we can from the limited sources we have available using the lowest amount of energy possible is becoming a challenge indeed. Geothermal DLE seems like a good start, but the number of places in the world with both the correct geochemistry and the tectonics to support such operations is limited. It’s going to take some clever engineering to get at the rest of the lithium that’s available, at least with the technology and energy resources we currently have.
[Banner photo by PABLO COZZAGLIO/AFP via Getty Images]
Or we could hurry up and build the nuclear plants we should’ve built in the 70’s, so we don’t need batteries for things that shouldn’t need batteries… like energy production.
I really don’t get why anyone would want to exclusively rely on energy sources that only work half the time at best… when we can buy nuclear plants that work 100% of the time and costs less than the batteries for the alternative would.
TL;DR: Stop reinventing the wheel – this problem was solved half a century ago with nuclear.
I don’t think 100% nuclear is a very practical solution, but I do think nuclear should be 100% of baseline load-it takes quite a long time to cold start one or adjust power output up or down;
adjusting power of reactor up or down or load following is fast enough as in minutes not hours.
Even if we had fusion we would still need some way of storing energy for things not directly attached to the grid (like vehicles, mobile devices etc).
Not sure why nuclear got mentioned, but I’m a hackaday reader so by law i’m requreid to have an opinion on it.
Nuclears biggest problem is people perception of it, its is essential a public relations issue and no amount of engineering is going to solve that. Changing the intrenched opinions of a large group of people is probably one of the most difficult task there is, which is why I cringe when people say Nuclear is the solution…it has a very big problem which i havn’t seen anyone propose a real solution to.
I’m with D here. Talking about how the energy is generated is unrelated. Lithium is mostly used in energy storage.
Except for, as Dan mentions in the intro, dilithium.
Factor in the costs of retreating , storing used fuel and decommissioning old power plant, then the whole nuclear industry is not profitable anymore.
https://en.wikipedia.org/wiki/Nuclear_decommissioning
Put them in the middle of nowhere, where no one will ever live. Middle of the Sahara/Amazon jungle/Death Valey/etc, may be exaggerated examples, but you get the point. Build them underground and no one sees them, and there so no need to get rid of them either. Store the used up fuel under, on the side of the plant. No one is around, no need to decommission them until more of them are making enough of a profit and we have no other things to worry about climate wise to then worry about dismantling them, if at all necessary.
Build them so that we can get rid of emissions and worry about dismantling them after the world is no longer worried about extinction. I think that’s a fair price to pay.
Nuclear? Seriously? Ask the people of Chernobyl and Fukushima what they think about nuclear. Or no, ask the French, who are now battling with electricity shortages because they have dramatic corrosion issues with quite a few of their reactors. Or wait, ask the Brits who slightly underestimated the cost for the Hinkley Point reactor. Or ask the coming 10.000 generations who will have to deal with the radioactive waste that was left by our generation of stupidity…
Actually us Brits aren’t paying for Hinckley Point, that would be the French and the Chinese.
Also we’ve fixed the price of the electricity generated, initially thought high, but now the gas price has shot up, it might be a bit of a baaargin.
All we need now is for EDF to fix the design flaw and finish it.
French Electric Network is higly stable, they export electricity to neighbour countries, have one of the cleanest ratio of CO2/KWh in the world, and Electricity cost in France is very competitive. So, yes, ask the French!
it won´t stay competitive very long, since many power plants will have to be retired in the next 10-20, the decommissioning costs will be absolutely *dramatically high*.
All the nuclear disasters have been with reactors of designs stupendously old, and often who’s primary function wasn’t power generation anyway…
Also the radioactive waste really isn’t an issue – take lots of highly radioactive naturally occurring stuff, refine it a bit and throw it in a reactor where all the radiation is nice and contained, safer than when it was just kicking around in the world… And at the end have lots of less radioactive stuff – with a brief period, assuming you don’t make use of ‘spent’ fuel from the first reactor to power the next, of more radioactive spent fuel – as all the newly created short half life products decay, but guess what that happens while its stuck in a cooling pond deep enough you could swim in it and not receive a meaningful does of radiation from it, and because they are short lived won’t take 10.000 generations, won’t even take one to go from being super dangerous radioactive spent fuel bits to less radioactive than the starting fuel spent fuelrods, that will still be stuffed in a hole safely out the way.
Yes some of the French reactors are offline, but that same things happens in all power generation facilities -the maintenance checks lead to “oh shit boss x is wearing out”, which leads to the shutdown for repair/upgrade – just because there is radioactive material involved doesn’t suddenly make proper if unscheduled maintenance new or scarier – what would happen to you downstream if the flaw in your hyrdopower station wasn’t fixed? Or to those living nearby if the fuel stocks for your fossil fuel plant started leaking, burning, perhaps blowing up… Anything that is supposed to handle such stupendous energy is bloody terrifying if you think about it that way, but ultimately with the right engineering and maintenance perfectly safe.
Well this is a perfectly stupid hot take. You completely ignored the fact that nuclear fission reactors are really just a stopgap until we get nuclear fusion reactors working. With fusion reactors, we’ll have all the energy we need to stabilize the radioactive isotopes that are left over. I give it 200 years tops.
More people get killed by solar every year than have ever been killed by nuclear power. You should know better by now
Citation needed.
Unless you’re referring to melanoma. Then, well, you’re probably right. We should probably just turn off the sun…
This is actually true, but it’s a result of workplace accidents during installation in all kinds of awkward places, namely rooftops/residential/etc. If you Google it you’ll find the citations and data easily.
Roughly about 200 people in the world had their health impacted by those episodes you mentioned. Would you care to compare that to the amount of people who get screwed by air pollution from coal/fossil fuels or possible lead poisoning from discarded solar panels per year?
Also, those were old tech. There are reactor designs today that makes reactor meltdown impossible, reactors that are fueled by those old reactors’ nuclear “waste” and small reactors that can be peppered all over the globe, making electric energy virtually free (when measured by kw/person alive in the world).
Having that much cheap and useful power would make a huge push for battery technologies.
It would make water desalination costs a thing of the past, therefore solving the so called water crisis.
People living in squalor all over the world for the lack of resources would be a thing of the past.
Sure, it could take 20 years to build one reactor. But consider the huge amount of emissions that can suddenly disappear when that goes online.
The energy consumption of a person’s entire life time can be supplied by nuclear fuel volume of about a can of soda. And that can go right back into the hole it was dug up from when done with.
But yeah, you go on drinking the Greenpeace Nuclear = Extinction flavored CoolAid.
If we are serious about reducing the effects that electricity generation and storage have on our planet, irrispective of generation or storage method, there will always be a negative impact on our planet. Why not look at the source of the problem and rather reduce consumption. We can begin by identifying non essential appliances and turn them off. Air conditioners are a good example and, there are many more. It might change the way engineers are thinking when designing megastructures in future. There are many more examples, AC is only one of them. Do we really need to boast with the tallest buildings, just think how much power that building is consuming. Don’t tell me that there is not enough ground availible to expand cities, if somewhere else we are destroying the earth. My own humble opinion as usual. Electric 0cars? Powered by renewable energy sources, yes! Powered by coal or nuclear sources, you must be crazy if yoy think it will not have a negative effect on the environment. All you do is moving the source of contamination to the place where the power is generated.
That is such a weak argument … I mean ask the of Boston what they think about the great molasses flood. You also assume that the technical problems of the past will not be solvable in the future. New York City used to be knee deep in horse manure. London had blackouts caused by coal smoke. LA had impossible levels of smog. All of these have been overcome by advances in technology.
None of the things you mentioned leave waste products that are dangerous for 10,000 years.
Every single problem you mentioned simply washed away and got diluted in the environment before settling or breaking down.
Every scrap of nuclear waste humanity has created is still around somewhere.
Do you have any idea what would happen if one of those long term storage sites got flooded or hit with some serious natural disaster? Perhaps a disaster that we couldn’t forsee when we created the site pre-climate Change?
Ask the people of Pripyat [Chernobyl] and they will give you an opinion based on experience obtained from a very outdated design being experimented on while live by people who didn’t really know what they were doing.
Ask the people of Fukushima and you get an opinion based on running a slightly less but still very outdated design on top of a fault zone in an area prone to tsunami.
If you get to ask the coming 10,000 generations anything ask how they will deal with our coal waste! Besides the planet warming CO2 there are all the heavy metals and yes, even radioactive ones that are released either into the air via the exhaust or into the ground and water via the ash. There is more radioactivity released during the lifetime of a coal burning plant than during the lifetime of a nuclear one.
But.. but… but… but… Nuclear! I’ve been told nuclear = bad.
What a gigantic effort to make a satellite image pixelated!
Thanks for killing mother earth, demolishing forests, melting ice caps.
There is nothing to fear.
As the demand grows, the supply also will.
It is just stupid to say the worldwide production is just as it is and all of it is used, every year. What’s the expectance? That someone will build a huge pile and just sit on it till there is a demand for it?
Telsa’s method is the first time I’ve heard of mechanochemistry i.e. literally hammering molecules together.
Why not cover the evaporation ponds with tents to condense the water vapor and reuse it? Everyone’s built a simple solar still in kid scouts, is there something that prevents the idea from working at a large scale?
How much does that material cost, and how many square kilometers of it are you going to need? What percentage of the sunlight is it going to be reflecting away, thus slowing the process down? How many tent-poles, pipes, maintenance technicians?