Although every electrical grid begins with the production of electricity, there are times when storing this power in some form instead of using it immediately is highly convenient. Today’s battery-powered gadgets are an obvious example of such time-shifting, but energy storage plays a major role on the grid itself, too, whether in electrochemical, mechanical or in some other form.
Utility-level energy storage is essential for not only stabilizing the grid, but also to time-shift excess energy and provide a way to deal with sudden spikes in demand (peak-shaving) plus demand drops by absorbing the excess energy. The health of the grid can essentially be regarded as a function of its alternating current (AC) frequency, with strong deviations potentially leading to a collapse of the grid.
Naturally, such energy storage is not free, and the benefits of adding it to the grid have to be considered against the expense, as well as potential alternatives. With the rapid increase of highly volatile electrical generators on the grid in the form of non-dispatchable variable renewable energy, e.g. wind turbines and PV solar, there has been a push to store more excess power rather than curtailing it, in addition to using energy storage for general grid health.
Types Of Storage
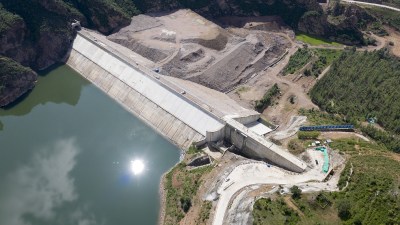
As mentioned, the biggest threat to grid stability comes in the form of loss of grid frequency, as this indicates a situation where connected generators and other inputs are no longer able to synchronize. The result of this is generally a cascading failure blackout which requires a slow and painful restart of the system to recover from. For this reason it is essential that on-grid storage is available that can respond to spikes and drops in demand so that supply and demand can be constantly adjusted.
These increases and drops in demand are highly dynamic, often requiring a response from an energy storage system within a matter of milliseconds, while other demand changes are more gradual and spaced out over the course of minutes to hours. For the fastest possible response, flywheel and battery storage are highly suitable, while pumped hydroelectricity storage (PHS) and compressed air energy storage (CAES) are suitable for more gradual ramp-up of energy absorption and release over longer periods of time.
When it comes to these grid stabilization systems, the actual cost of the energy is of secondary importance, as their primary function is to prevent the grid from collapsing. In that regard such storage systems are an integral part of the grid. This is different from the way that ‘grid storage’ has entered the public vernacular, in the sense of time-shifting large amounts of energy produced by renewable energy sources such as wind turbines and PV solar panels.
At its most extreme, a national grid is envisioned that uses nothing but variable renewable energy as well as hydroelectricity, while storing excess energy in grid storage to allow for time-shifting and release over the span of weeks to months as supply fluctuates. Naturally, in this scenario where dispatchable generators like thermal plants are replaced with mostly non-dispatchable sources and grid storage, a number of factors are essential. First is the system cost, followed by the operating cost, as these determine the price tag added to any energy released by these storage solutions.
To scale energy storage to the required levels for this scenario, technologies that can match PHS and CAES in terms of system and operating cost are required. For decades, reflow flow batteries have been developed as a possible solution.
Redox Flow Batteries
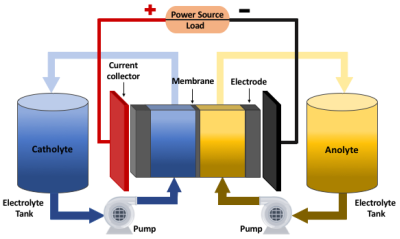
A redox (from reduction-oxidation) flow battery – or RFB – is a type of electrochemical battery that uses a liquid anode and/or liquid cathode electrolyte. In most implementations, the fluids for for the cathode and anode are separated by a membrane which allows for charges to be exchanged. Theoretically this kind of system would allow for a very large energy storage capacity along with most of the benefits of non-flow batteries such as fast charge and discharge response times.
Unfortunately, despite decades of research, even the most promising type of flow battery, vanadium-vanadium, has very low specific energy of ~20 Wh/kg of electrolyte where old-school lead-acid cells manage 25-50 Wh/kg and lithium-ion batteries over 200 Wh/kg. This means that the electrolyte tanks have to be quite large to match the energy capacity of e.g. a Li-ion or lead-acid based battery storage system. The density limit here is set by the ability to dissolve the redox compounds into the solvent, which for all-vanadium flow batteries is generally sulfuric acid (H2SO4).
These and other issues with flow batteries in general were covered by Clemente et al. (2020). Among the issues covered are also longevity, particularly with the membrane material, but also with the electrolyte itself due to unwanted reactions with hydrogen and oxygen. As flow batteries rely on electrolyte flow, the flow characteristics of the electrolyte, as well as the energy spent on pumping this electrolyte around and getting the optimal flow rate add engineering complications to what at first glance would seem to be a straightforward system.
Another such complication concerns the difficulty with determining state of charge. Since the voltage on the cell’s electrodes does not correspond to how much of the redox couple species has been converted to the charge/discharge state. With vanadium flow batteries, one option here is to estimate using the color of the electrolyte, as the redox process changes the physical properties of the vanadium species. For full automatic charge control, these are but many of the engineering questions.
As noted by Xu et al. (2018), the system efficiency of a flow battery is >60%, with ~82% possible with improvements in all-vanadium batteries. An unfortunate aspect of an flow batteries are the parasitic losses due to the electric pumps, in addition to ohmic losses and flow resistance losses. As the redox reaction is exothermic, some energy will inevitably be lost during operation.
Fully charging a flow battery implies having all of the redox couple species undergo the desired redox operation. Because this involves interactions at the membrane surface separating the two electrolyte flows, the likelihood of this decreases with the charge state as fewer redox couples will interact. Increased electrolyte flow compensates for this to some extent, but this likely increase the parasitic losses, and makes simply scaling up the system by increasing the tank size unattractive.
When compared to the much higher round-trip efficiency and lower complexity of e.g. Li-ion batteries (>90%), it should come as little surprise that most large-scale grid-level battery solutions in commercial operation today employ such Li-ion cells.
The Cost Picture
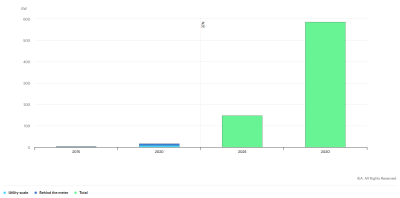
In the current applications of grid-storage, not an enormous amount of capacity is needed. With a few megawatts of strategically placed flywheel- and battery-based systems enough capacity should be available to respond to shifts in demand with some limited time-shifting.
For long-duration (~4 hours) storage, CAES and PHS are highly preferred where the environment allows for them to be used. A common use of such long-duration storage systems involves storing the energy produced by thermal power plants in times of low demand. Thermal plants often have optimal operating levels which makes it cost-effective to time-shift excess power for later discharge.
With more non-dispatchable sources being added to the grid storage capacity has to be increased for time-shifting like never seen before, along with the entire electricity market business model. Whereas thermal plants and hydroelectrical plants sell the electricity they produce on the capacity market using multi-year contracts, variable renewable energy installations sell the produced electricity on the energy market, which means the power they produce is opportunistic and unpredictable. Essentially electricity is sold to the grid when it’s available, regardless of whether it is needed at that point in time or not.
What this means is that for successful grid integration, these systems require significant amounts of spinning reserve to compensate for drops in supply, and storage of excess power as detailed in e.g. the Net Zero scenario projection by the IEA, which sees the current 10 GW of utility-level storage (in addition to 7 GW behind the meter) increase to 600 GW by 2030.
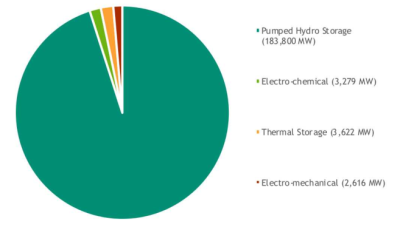
In a 2013 analysis by Purdue University’s State Utility Forecasting Group, the projected cost for a number of storage solutions were compared. Noted as an essential point are the system costs over time, which in the case of both PHS and CAES are quite minimal, since these systems tend to require relatively little maintenance while lasting many charge/discharge cycles. At the time Li-ion batteries were not a big player in the energy storage market.
In a more recent analysis by Sandia National Laboratories from 2018, we can see that the overwhelming majority of grid-storage is PHS (>183 GW). Of battery storage in the US in that year, Li-ion already made up 78% of installed battery storage, a number which has grown by a number of GW since. For example with Vistra’s 1.2 GWh (300 MW) Li-ion facility at Moss Landing, Monterey County, California, and its subsequent Phase II extension. Although both installations are currently offline, having caught fire, showing an inherent risk with battery storage.
According to the US Energy Information Agency (EIA), US power usage will reach about 4,000 TWh in 2023, which gives some indication of the challenge if a significant part of the world’s electricity needs are to be met from utility-level grid storage. Taking the Vistra Moss Landing storage as example, to cover 1 TWh (0.025% of the US demand), 625 of these 1.6 GWh facilities would be required, sufficient to cover the nation’s demands for a brief while. This lack of large-scale, long-duration storage is also noted in the Sandia National Laboratories report.
The Environmental Impact
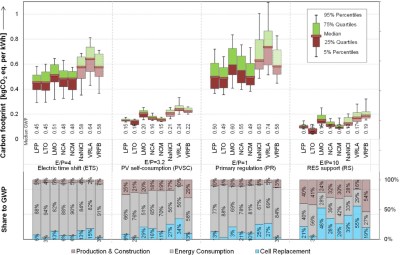
It seems clear that at least for now Li-ion battery systems are taking the spoils with grid storage since both PHS and CAES cannot be reasonably expanded on account of their limitations with regard to geology and similar. In addition to the higher costs of Li-ion, an important consideration becomes the environmental cost of these batteries, as the manufacturing of batteries is energy intensive and produces significant amounts of greenhouse gases (Baumann et al., 2016).
With the addition of such battery systems, the effective carbon emissions of variable renewable electricity can reach levels of around 100 gCO2e/kWh when lifetime emissions are taken into account. When considering the economical cost and environmental impact of carbon emissions, this would thus seem to be rather self-defeating from an environmental point-of-view, not to mention costly.
Using dispatchable hydroelectricity, coal, gas and uranium the conversion from gravity potential, carbon or fissile matter into electricity is performed on-demand. In comparison using mostly intermittent sources for powering a nation’s grid would seem to be an unsolved problem as this requires storing electricity in enormous amounts. Although a reasonable solution may be found in the future, at least at this point in time there is no mature, scalable technology that can perform this function in a way that could be considered economical.
[Heading image: Bath County Pumped Storage Station (Credit: CHA)]
>Although a reasonable solution may be found in the future
Power-to-X. Synthetic petrochemicals, basically. The only way to economically store huge amounts of energy and produce all the stuff that we’re using petroleum for anyways.
Another way of seeing power-to-X as a concept is to store energy in the products you’re making anyways. You simply make them when you have the power, and don’t when you don’t, then consume at your own leisure.
It doesn’t even have to be esoteric catalytic processes. Heat and cold storage are good examples. Freeze a cubic meter of water and you have stored 437 MJ or 121 kWh of energy for later.
I always liked aluminum for that, it’s been called “crystallized electricity”, it’s stable to store and a large proportion comes back out if you “burn” it in a fuel cell.
Only problem is, aluminum production doesn’t lend itself to intermittent use because the molten electrolysis cells can’t run cold – it takes days to get them back up if the power goes out, and using them at a low duty cycle wastes large amounts of energy just to keep them from freezing up solid.
That’s why it’s mostly done in places like Norway where there’s a continuous supply of cheap hydroelectricity.
Indeed, thermal storage is dirt cheap and works for a very large fraction of residential energy use – HVAC and hot water.
Then there’s automated load management for residential loads that can easily be rescheduled. What’s left over is mostly lights and home electronics, a small fraction of the total.
Or, see Drake Landing solar community in Canada. They consistently get 90-100% of heating from solar power collected in the summer. It’s kinda like distributed/district heat system where you pipe hot water around.
>automated load management for residential loads that can easily be rescheduled
I don’t like that, because it mostly represents deferred demand, and it tends to come back as large demand spikes when people eventually have to turn on their devices, else their fridges melt of they can’t wash their clothes. The more you push it into the future, the more it comes back as one big wave.
If there is any load management, it should be on the consumer side, not on the supply side.
This is where the smart grid could come into play. Imagine if all your most important appliances were equipped with smart grid tech, and everyone was given a “critical power energy budget” of some low value like 1000 kWh. You could say “I live in a cold climate and it’s most important to run my furnace” so everything else would get shut off. An apartment owner could say “I have 32 units, so I’ll budget 32000 kWh to the HVAC systems. Or you could decide “I value my freezer more than my comfort” so your freezer gets the budget. When the next major grid event strikes, the alert goes out and customers immediately shed all but their most important loads.
I know such a thing would be almost impossible to implement politically, but if people could automate their cooperation it could save everyone from these costly, ugly grid collapses.
>everyone was given a “critical power energy budget”
In other words, a centrally controlled energy ration. What would go wrong with that…
Perhaps there should be more emphasis on turning loads *on* rather than off for demand management. Any load where using more now means using less later can be applied. For example, thermal stores can be “recharged”, such as by setting water heaters to maximum safe temperatures or by setting dedicated freezers to the coldest setting.
> more emphasis on turning loads *on* rather than off
That actually does go better with both solar and wind power, because they both have a tendency to produce a lot of power over short periods of time: a couple hours around mid-day (except when it’s cloudy), or 1-2 days in an average week for the wind turbines. They both have a high peak-to-average ratio which is the problem that requires a large amount of storage capacity.
To best utilize them, you have to be able to sink down a lot of power fast, and then use it gradually over time.
We prefer to call it carbon monofilament hydrogen storage. ;-)
Much more politically palatable. Can’t say you’re making synthetic gasoline.
“It seems clear that at least for now Li-ion battery systems are taking the spoils with grid storage since both PHS and CAES cannot be reasonably expanded on account of their limitations with regard to geology and similar.”
This doesn’t seem particularly clear to me. The pie chart just above shows PHS to be dominant, and while it can’t be installed everywhere, there are plenty of places it can be. The paper linked at the end of the paragraph is an overview of battery chemistries compared to one another, not why it’s taking the spoils relative to electromechanical storage.
Is there more context for this statement?
PHS is dominant because it’s already built, decades ago, in places that were/are cost effective or just massively subsidized regardless.
Having new facilities is difficult because of public opposition, cost, but also a point that the article does not mention which is that all artificial dams release methane due to decomposition of organic matter that gets covered in water.
Fair enough! I’m just uncomfortable with the idea of using exponentially more batteries while we still don’t have a battery recycling industry worth mentioning. It feels like other options should be actively explored where it makes sense, and we shouldn’t just take battery storage as the new de facto king.
We won’t be using “exponentially more batteries” without first having a battery recycling industry. It just won’t be possible.
Efficient and economically viable battery recycling technology is going to come. That’s an absolute given. It may take years, but it will eventually come. Batteries in storage solutions built now will last many, many years before they need recycling. Why wait for the recycling technology to be around before we start ramping up battery production when we won’t need the recycling technology for maybe a decade after we start ramping up battery production?
Because it might not come when and how you planned it. If you try to run before you can walk, you may succeed on the first few steps, but you have to learn pretty fast or you’ll end up on your face.
On the other point, there won’t be ramping up battery production without recycling them for materials, because the supply can’t keep up.
CAES is partly still in development and most installations of it aim for power density instead of longer term storage.
In short, a lot of people willing to invest in compressed air wants to see it regulate the grid hour to hour, something batteries are great at. The main advantages of CAES over batteries is that a pressure tank is relatively cheap for the amount of energy stored, and the self discharge is marginal.
CAES is better at day/night cycles if one has a 1:10 power to capacity ratio. At a 1:15 one can theoretically start to average out day to day variations.
However, CASE has the downsides of:
1. It needs a large installation to be economical, mainly due to the maintenance required for the mechanical parts involved.
2. It is generally seen as inefficient, old fashioned, and “not cool enough” to be worth while investing in. (Give it lasers and a crypto currency and people might flock over it though…)
3. For near isothermal operation we generally need a lot more capacity than power output. Thankfully it is the power out/in bit that is the main bulk cost from the maintenance perspective, since a pressure vessel is relatively low maintenance. (and for everyone saying “why not adiabatic?” but this requires much more expensive pressure vessels due to requiring very good insulation to not loose the heat, at this point batteries are a better alternative…)
I’d argue CAES doesn’t need to be large to be economical, at small scales It is basically just a shop air compressor which are rather over engineered and so mass produced any parts that do end up being needed will be pretty cheap.
I would suggest its probably more economical at that sort of small scale than most battery systems as all the charging circuitry and inverter will fail eventually and the battery itself does have a finite lifespan, all those rather highly complex bits of electronic engineering far more expensive than the simple mechanical wear parts any machinist here could make for themselves if they really wanted to…
Being Large helps CAES in making efficient isothermal operation for a given output easy or gives the potential for much more massive bursts of power on demand the smaller system can’t manage.
Please calculate how much energy a shop compressor will hold, and contrast that to the price and service life of one.
How much it holds is entirely down to the pressure tank, which can be any size you like and really doesn’t cost much to make bigger – just string a few extra standard tanks together, or make a large scale one, which isn’t particularly hard or expensive when you stay to low pressures. The moving parts that can wear out from use are the pump element not the tank, and that normal shop compressor pump is massively mass produced (not to mention that same pump will ship with tanks of a variety of sizes too – my compressor for instance has the 100L tank, but the same pump is available off the shelf with various sizes up to 200L bigger than that you get the next size up pump)…
As for service life the half decent ones seem to keep going forever, I’ve seen ones older than I am that still work and have had lots of use – I assume less effectively than they used to as no amount of proper oiling and maintenance can negate all wear, but still they last a very very very long time if you look after them at all, and the most common point of failure does seem to be be the pressure tank being left full of water not the pump…
1 m^3 compressed air at 160 Bar holds about 22 kWh of energy, not accounting for discharge losses. So a fair bit higher energy density than a lead acid battery installation. (since the battery installation typically needs room for air to keep it cool, service access and cable routing.)
But that is at 160 bar, not an unrealistic pressure for a large scale installation, but most shop compressors only gets to 8-15 bar typically. Though, the main thing going against shop compressors is their laughable efficiency since they are built primarily for a high flow rate.
‘Laughable efficiency’ is rather unfair, at least on some of them, though its certainly true no shop compressor goes in for maximum efficiency over airflow volume.
Ultimately though when its storing the spikes of excess renewable it don’t actually matter much how good the throughput efficiency is as it would have been wasted otherwise, though more efficient is obviously better. What matters more is the cost to build and maintain the system, so using a less efficient compressor that is durable enough to work for decade after decade can make sense, especially at the smaller scale where the cost of building and maintaining vs value of energy stored isn’t comparable to the massive systems where dropping 1% efficiency equates to many mW maybe even more – which would have massive value.
>the pressure tank, which can be any size you like
Not quite any size.
>and really doesn’t cost much to make bigger
The house and property to keep it in does, plus the safety factor of keeping several kilowatt-hours of energy in air pressure. At some point you have to start bullet-proofing the neighborhood…
Energy stored as pressure isn’t inherently any more dangerous than energy stored as combustible fuels, which are often pressurized as well!
So yes you need some safety in the execution, but the same is true of the natural gas piped around, the gas cylinders folks not on the gas grid need, the corrosive battery off gassing and fire potential – storing large volumes of energy always has risks, and always should be properly managed. Storing energy as air pressure isn’t anything new there, I’d suggest its actually much safer than any other energy storage method – its not going to start a fire anywhere near as easily, any failure of containment is almost certainly just a tiny harmless airleak, and serious failures are mostly just going to throw a little debris that likely hurts nobody (odds of hitting somebody in such a failure are damn nearly zero), and even a full on pipebomb level failure only throws shrapnel, really dangerous but of no further danger in and of itself after its happened, where all combustible fuel problems will likely set everything else remotely burnable on fire, so nice toxic smoke clouds from burning plastics to go with carbon dioxide, monoxide and just the fire itself…
While I do agree building space has a cost its not all that significant when even a tiny increase in size in any direction gets you huge volume increases – buildings are big… Also you can fit pressure tank of any convenient size into a large array to make them fit an existing building – which won’t necessarily be any problem cost wise over one or two really giant tanks – bigger tank needs to be vastly stronger to hold the same pressure with safety margin – much harder to make.
Also (assuming the other comment will get past the moderation at some point) its got to be a bigger tank within sane limits for compressor size – no point in a tank capable of storing 6 years worth of your household use but that will take 3 years to pressurize enough to use at all… So really you are talking for shop compressor less than 1000L of volume needed I would suggest, certainly no more than 5000L – just take too long to fill. And that sort of volume is easy enough to loose in most buildings (sure it doesn’t hold huge amounts of energy at that size, but its still more than enough to be very useful, and can actually be recharged in a viable time from such a compressor – we were talking small scale afterall)
3 foot diameter mild steel tank, 160 atmospheres. Wall thickness 3.25 inches. Tank weight about 2 tons. No safety margin but also not in fatigue stress, so adjust according to risk tolerance.
Wall thickness scales linearly with tank size. You don’t change the steel to volume ratio by going bigger. Surface area on order of size squared. Thickness or order of size.
22kWh isn’t that much energy, until it’s released all at once. Regular shop compressors are known to cut people in half when tanks fail. At 10 atmospheres.
Use the compressor tank drain folks. The ass you save might be your own.
Actually HaHa you most definitely do end up changing the steel to volume ratio – as at smaller scales you have way more wall thickness than the pressure itself requires, to make it strong enough for protection from external dings – so dropping that penny doesn’t pop the tank like a soap bubble, and at giant scale the wall thickness required for that little over pressure safety is more than enough to protect you from errant little knocks like our hypothetical penny but you end up needing a great deal of additional structure to distribute the weight of the steel walls of the tank – it might not be required to hold the pressure but it is still required… Somewhere in the middle therefor you should find the lowest material cost to volume.
Also the cost to make a giant vs small tank is not just materials – a fire extinguisher sized tank for instance is trivial to mass produce and ship, where the giant 2 ton monster you describe is very hard to ship, and getting any bigger than that you end up having to assemble it on site, with each component still being stupendously heavy and so very hard to ship…
I do agree entirely on using the tank drain though.
>Energy stored as pressure isn’t inherently any more dangerous than energy stored as combustible fuels, which are often pressurized as well!
Yes it is, when we’re talking about very large tanks of air pressurized to hundreds of bars.
If things go wrong, in one case you’re most likely to get a modest puddle of something like diesel oil and a mess to clean up. In the other case, fragments of metal and stone flying around at the speed of sound.
>1 m^3 compressed air at 160 Bar holds about 22 kWh of energy
Which is comparable to two gallons of diesel oil, which can be safely held in a jerry can with no extra provisions.
Oops, two liters of diesel oil – not two gallons. Sorry, I’ve been drinking today :)
>If things go wrong, in one case you’re most likely to get a modest puddle of something like diesel oil and a mess to clean up. In the other case, fragments of metal and stone flying around at the speed of sound.
Seriously Dude take the best case vs the worst case
Almost all CAES failures, like any air system won’t explosively let go, they will simply leak first, letting out harmless air… Just like most gas supply problems are just minor leaks and don’t blow up the house they happen in, it leaks, gets noticed and then fixed, but every now and then that house and quite likely all the nearby properties are damaged by the giant gas explosion…
So CAN it go very wrong absolutely!..
But the same is true of everything – even of hard to ignite fuels like Diesel, if that stuff is ignited it is then very hard to put out both it and the resulting fires – you have to be there with the right type of extinguishing equipment really early – as its got way way more than enough heat output and thermal mass ignite damn nearly anything.
As for lighter more volatile fuels, well they will find it a bit harder to ignite surfaces they are burning on top of, but its perfectly possible for a ‘nice’ fuel air explosion, they will still be able to ignite stuff, and are far more likely to be ignited accidentally, and that is not to mention often being massively thinner liquids or gaseous they will just flow under doors, around corners burning merrily a very long way to find something they can ignite…
One limitation is how many GWh of battery storage can be produced in the coming years. Tesla produced just 4GWh of grid storage batteries in 2021. That would run a country like South Africa for less than ten minutes. Even with ambitious ramping up of production we will still see major bottlenecks on the supply side.
>to cover 1 TWh (0.025% of the US demand), 625 of these 1.6 GWh facilities would be required
Or, if you’re clever, just build 2 and use them every day instead of once per year, since we’re talking about yearly electricity demand.
Since we’re talking about yearly demand, you need capacity that is used just once per year – to do things like carry excess solar power from summer to the dead of winter.
Lithium batteries would also be massively cost-inefficient and extremely energy-inefficient to build for that purpose since you would only use them for 15-20 cycles before the end of their calendar lives. The cost to manufacture a generic lithium battery is about 200 full cycles worth, so your energy efficiency in storage would be less than 10%. Doing just about anything else would make more sense.
The same goes for all the rest, though. Imagine building a massive pumped hydro dam and only ever using it maybe a hundred times over so many years. That’s why the “battery” to store the energy for seasonal and yearly variations must itself be extremely cheap. It doesn’t need to be very efficient, as long as it costs almost nothing to have.
Power-to-X technologies fill that point by the fact that the product you are making and consuming is the battery. If for example you’re making synthetic methane from air and water, the gas grid will happily hold it for six months at virtually no additional expense: it’s already built to do exactly that. Even if the energy you get back from it is just about a third of what you put in, it’s still 3-4x better than any imaginable lithium battery.
Yes, seasonal storage really needs to be cheap as hell. And why I personally don’t think seasonal storage is “the right move”.
Wind at least where I live would though be 2 cycles per year since it mainly blows during spring and autumn, but still…
Day to night variations is likely the best option, effectively cutting out the low efficiency generation facilities from the grid since with enough storage one won’t need “peak power” from generators. Week to week storage is also likely quite viable, since some weeks are better than others.
But just running factories when there is most power available for them is also a wise move. Have seen some ideas thrown about using a algae bioreactor, since one can then turn the algae into what effectively is crude oil. And the rate at which one can expand a pond of algae is quite impressive, so scaling it up along with increasing power availability isn’t hard. Or just run any other carbon capture to hydrocarbon process.
But using power when power is most available is a good solution to not need as much storage in the grid.
I for one try to avoid using extra power when I know there is a lack of it on the grid.
One has to avoid taking any advice to the extreme: running factories intermittently is neither cheap nor efficient, and it places additional demands on the supply chains to stockpile materials and products.
Some fields of work can operate like this, others can’t.
Which is why factories bid into the power pool at their own curtail price(s). Just like a generator.
The curtail price is more of a soft limit, and the factories will run to cut losses even above that – if they can predict the price to come down soon. When the power prices fluctuate too much, many factories start to leave the grid and begin to generate their own power because it becomes a smaller penalty than dealing with stop-and-go operation.
This happened in Germany, where lots of factories started buying natural gas and running their own generators to deal with price volatility. In the official figures, the electric grid got “cleaner”, because lots of people who couldn’t use intermittent renewable energy bypassed the grid entirely.
Dude:
The curtail price is a pretty hard limit once the users bid into the pool. Operational details are rolled into the price, along with awareness of the pools dispatch duration (hourly, 15 minutes etc).
Curtail price is assumed to include all their costs, I mean ‘they’ bid it. (I made some good bucks helping them model the system with real-time initial conditions.)
Most industrial uses won’t generate their own power unless they also use the waste heat. Even then they will sometimes curtail their own operations and sell power, if it gets valuable enough.
Devil is in the details. e.g. Aluminum producers will set two prices. First shuts down production, but doesn’t let the system solidify. Second is much higher and covers the cost of restarting.
>using power when power is most available is a good solution
One has to be careful not to use that argument to support energy rationing. The implication being that there would be an authority to decide how much and what energy is available to whom, at what prices, or who makes it, with what technology etc. It would be a gigantic problem in terms of basic corruption and politics: a crony capitalism based on energy.
Make energy pricing more responsive to actual demand and supply. People, and automated systems, would then be incentivized to buffer the system.
That is a solution, not necessarily something everyone likes.
We already have “market price” power contracts that adjust according to the spot prices. Only problem is, when the price goes up wildly, people have no options to shut down. Every year it’s the same. Just before Christmas the power companies start calling you and cajoling you to switch over to “cheap” market priced contracts with only “six cent power prices” – yeah, at night when I’m not using any. At some point in the future when the supply variation becomes bad enough, they will stop selling regular fixed price contracts and push all of the problem onto the consumers, who can deal with it no better.
Last year we had super high power prices for a while (28 c/kWh average, 120 c/kWh peak), and families with “cheap” market priced contracts were suddenly facing 1000 EUR power bills. Some households were turning down heat, hot water and stopped cooking at home for a month. Can these people also afford the batteries to bridge a week or a month of shortfall?
In CA during the initial power pool mess, we were paying PG&E market for gas and regulated price for electricity.
So it made financial sense to turn off your gas heater and get by on electric space heat for the duration of the first power pool winter. PG&E was already broke and had it coming anyhow.
I’ve always thought ‘perverse economic incentives’ would be a good porn movie title.
I was the guy who handled the power trading value at risk (VAR) report grand total overflow bug six months previously.
Curse the SEC and my cowardly ass for still having to work. I’d be out of prison by now, drinking Champaign out of fitness models cervical caps!
So. Nuclear. Ez. Looks cheaper than the batteries by themselves..
Can we just get started already and stop trying to solve a problem that’s already been solved.
Campaign slogan can be.. “Got Uranium?”
1) great if and when we develop cheap safe reactors.
2) Uranium is a nonrenewable fuel. Can we develop reactors that run on renewable fuel?
I hear thorium mentioned the most often when this comes up. A glance at the wiki page shows a bevy of potential benefits, though I’m sure it has its share of detractors. There seem to be a few research reactors running in China.
1. Gen III and later reactors are already safe enough. SMR and others will be safer still.
2. Having a thousand years of supply is just as good, especially if the alternative hardly works at all.
Nuclear reactors by definition don’t run on renewable fuel, but we have literally hundreds of years of fuel available. They also produce many gigawatts of power without any CO2 released at all. At that point grid storage is just redundant.
As for safety, it’s a problem that was solved with improved reactor designs which *themselves* have been around for decades now.
For reference Fukishima was built in ’71. Chernobyl was built in 1970-77.
Nuclear is easy. Remediate the NIMBYS, not so easy.
People like gambling. Start a federal lottery for townships where the “winner” gets a nuclear reactor and all applicants get a new school or civic center or football stadium or casino.
Or give them discounts on energy!
I think some cities near nuclear power stations get free district heating or other perks.
Every person who claims to be an environmentalist and is not pro nuclear can just staple their mouth shut in my opinion. I’m beyond fed up with it. Solar and Wind will never provide the power we need when we need it and even if it could the environmental damage would be 100x that of nuclear plants.
The only argument against it is.
Oh but look at the 50 year old poorly designed soviet built nuclear power plant it obviously is a concern with modern day safety protocols.
Or oh look Japan had a more modern one and it failed after being hit by record breaking earthquakes and tsunamis. What do you mean just don’t build the power plant on fault lines that’s impossible.
If I could I would put a nuclear reactor in my back yard.
The real argument against nuclear is that we are running out of fuel. Yes, uranium is non-renewable, and the kind needed by the installed reactors (almost all of them) is hard to get indeed.
One day breeder reactors will solve this, but today is not this day.
Meanwhile in Southern California a close friends family died from not checking their new well and hitting a very potent uranium source. They sold the 20 acres of land 15 years ago. Now the land is being terraced and leveled for a housing tract…
The real argument against nuclear is Capitalism. The greed of even a single human can spell doom for thousands.
If the issue of dealing with spent fuel had been dealt with from the outset (and “I can’t see it no longer, so it must not exist anymore.” is *not* dealing with it) public opinion would not have turned. It is the old proverb: You reap what you sow.
It probably is not great to use The Simpsons as your authority on reactors and the energy business.
Good thing capitalism is ending fission power. Can’t make money at it, pretty much anywhere.
The real mess is when the government owns the power company, the nuke plant is a sunk cost and the ‘president’s’ nephew needs a good job in the control room, is promised ‘no math’.
The irony is, we’re sitting on top of literal mountains of nuclear fuel because it’s a waste product of rare earth elements production. Mining companies in east Asia are trying to stuff it away anywhere they can to avoid dealing with it.
In the end, there are thousands and thousands of years worth of uranium and thorium just laying around, and to not use it is frankly idiotic. Even if it runs out eventually, it is energy you can use; it’s like a lottery ticket you refuse to cash in on the excuse that you can’t repeat the performance – pure madness.
One of the rare occasions I agree with you entirely it really is idiotic to not use it, the only questions are how and where.
For me the best idea is mostly to use the smaller self contained reactors for much of it, its more expensive but more flexible in deployment and no ‘act of god’ level event can cause real harm as the things are easy to make incapable of meltdown and anything as stupid as say shelling a nuclear power station using them can only spread a very tiny amount of radioactive material as they only contain a pretty tiny amount – the danger posed even from deliberate attempts at harm is so minimal as to be lost among the many other dangers of living (no comfort to the poor sods who probably die horrible deaths or their families if such things ever come to pass, but life is like that)…
A well designed modern big reactor is very safe, more than safe enough IMO to use, at least in many places (avoid the really geologically unstable etc) but its not quite as safe and lacks the flexibility being a giant immovable object it takes a long time to build…
Not really, solar and wind is more than capable of producing in vast excess of what is actually needed, and without wrecking the joint to do so, assuming we actually at some point stop being so stupendously wastefully inefficient in how we use energy – insulate buildings properly, decent public transport etc and it helps if you do the sane things like put large amounts of solar into all new buildings, clad select highrise and industrial sites with it so there is no extra environmental cost…
Nuclear is a good idea really, but its not actually required to give everyone more than enough energy to live and work with modern lifestyles, it just means less energy storage and renewable sources need to be built in hurry.
Solar and wind can only be relied on a couple percentage points of nominal capacity, so no matter how much you build you will always have gaps – you just can’t do without either long transmission lines to other areas, or some sort of batteries.
Nothing wrong with long transmission lines – they already exist in most cases anyway…
As for batteries, well energy storage of some sort is going to be needed, though how much who knows. And storing as electric isn’t the only option, make houses efficient and have large thermal stores you can always bump up the temp in the thermal store, and in most cases the load can be turned off for hours even days with no loss of function, so the supply doesn’t actually need to be that perfectly matched to ‘instant’ demand…
> they already exist in most cases anyway…
Not long enough.
> have large thermal stores
That is “some sort of batteries”.
Dude throughout Europe almost every nations grid is connected to every other nation, in many cases in many more than one place – its pretty much as long a transmission line as you can possible desire covering basically all of Europe – so stupidly large areas even across oceans as the UK is connected too!
Technically, however Battery is a term that seem to have become entirely about chemical electrical storage these days…
Then there’s the ecological damage of solar panels.
They’re made from reject silicon wafers, and the silicon manufacturing industry is responsible for *the* most polluted regions on earth.
How many solar panels does it take again, to equal a modest couple-gigawatt nuclear plant?
Or ‘Go Thorium!’ It has a lot of potential and very little nuclear proliferation potential.
On the other hand, you can’t really throttle a nuclear plants in real-time. You still need topping load capacity.
Well, I mean, how real-time? Certainly you can throttle the overall nuclear heat generation by adding / pulling dampers, and I would imagine one could pretty easily throttle to a decent degree just via messing with the steam turbine process (spilling steam etc)
The new EPR:
“power level variation between 60% and 100% NP Return to 100 % NP possible at 5%/min during 80 % of the fuel cycle. Unusual load-follow : low power level between 25% and 60% NP Return to 100 % NP possible at 2.5%/min during 80 % of the fuel cycle”
It is rather fast. From 25% to 100% output in 30 minutes. Going the other way, as you said, is a matter of blowing steam, basically. Not literally though, but rather dumping heat into the coolant.
i agree that the newest (and future) nuclear technologies are “the way” for bulk power,
but; “when seconds count”… nuclear “is just minutes away”…
then-again, with the newest nuclear technologies, maybe we can design a second “emergency shutdown” mode(s) to cope with excess power, along with the existing obligatory load-shedding to deal with lack of power. but people around here expect a certain reliability from the electric supply.
personally, i always like to remember that a dam-as-a-storage can actually be constructed underground instead, by turning a river into a waterfall into an underground tank, then pumping it out to takeup excess energy. as confusing as that may be for the average joe, storing energy by getting rid of something sounds backwards.
The reason why “seconds count” is because there isn’t enough rolling reserve on the grid thanks to renewables, which have made the problem far worse than it ever was.
Every large gas turbine is also a gigantic flywheel, and it actually will respond in seconds to load variations. The rest of the plant just won’t.
Ask the U.S. Navy how its done.
Or the U.S. Army…
>you can’t really throttle a nuclear plants in real-time.
Yes you can. It’s actually a design requirement for Gen III and later reactors. Sure, it costs more to operate them like that, but it’s still vastly cheaper than the alternative.
I am with you on that. Nuclear is the way forward until something better comes along (wind and solar aren’t it). But…the way it is going, we’ll be firing up a gas generator in our backyards to ‘supplement’ our non-wind, non solar days and weeks if certain people have there way. Going back to wood stoves and such for heat. Gotta love the mentality out there that push this stuff for a non-existent problem to begin with.
Agreed. Just Say No to all this battery nonsense and generate power on demand. A supernova did the energy storage for us a while back. Very good long-term storage. Modern reactors can use virtually waste-free decay cycles. The biggest road block is Hollywood and Luddites in university humanities departments. Maybe fusion will even be workable some day.
Just as well CO2 is not really an issue and fusion reactors are, according to TAE, viable and to be commercially available within a decade or two. https://www.youtube.com/watch?v=blUsX_f7Twk
they have been available in a decade or two since the 60’s…
Taking bets.
Which is first: Commercial fusion power goes online of full self driving Tesla (or any other) ships?
I’m taking fusion power, but give me odds and I’ll take your bets the other way.
Electrolysis to hydrogen to (optionally) Ammonia to combustion isn’t even discussed but billion dollar projects are in development based on this storage chain right now.
Another ammonia advocate; good to see. By my napkin scribbles, if a typical household could generate enough ammonia via solar to fill a 2000 gallon tank during the summer months, they would have stored about 5MWh, which is enough energy to cover the deficit of solar during the winter. The equipment needed for true independence from the grid: water distiller, H2 generator (via electrolysis), N2 purifier (from air), and ammonia generator (from H+N feeds), plus a fuel cell to consume it. The ammonia generator is the tough part, at least for now, but I’m hopeful, as the world really needs a source of truly green ammonia.
I can see it now, gleaming suburbs of houses, each with a solar array on the roof and… um… ten water heater sized tanks of pressurized ammonia in the garage.
Eh, people will probably maintain that carefully, right? What could possibly go wrong?
If this happens I am confident that the folks who control zoning will conclude that it is more efficient to store the ammonia in a few large tanks located in disadvantaged neighborhoods.
That’s a pretty big tank to store in the backyard of “a typical household”. Especially one of a pretty unpleasant chemical.
You could store it dry without pressure as a perchlorate.
Store it dry as Ammonium Perchlorate? Isn’t AP literally rocket fuel? That seems even worse than storing big vats of volatile ammonia.
Is there a way to be notified of new comments without leaving a comment? I don’t have anything to contribute at the moment but I’d like to follow the discussion.
Guess it’s moot now for this particular post anyway… ;)
Give the energy to my aunt… She’ll find a way to hoard it
Ha!
The easiest road to paradise is the road that’s already paved. Use superfluous energy to convert atmospheric CO2 and water vapor (*both* greenhouse gasses) into hydro-carbon chains. These can be stored cheaply in non-pressurized tanks and used to convert back to energy /or/ as raw material for creating plastics.
And for giggles, use the excess superfluous energy to recycle plastics back to small chained hydro-carbons.
I don’t get the aversion to bio-methane and synthetic methane, and it’s easily converted form, methanol, pulled from atmo with green input. All sorts of stupidity of “but it just lengthens our subsistence on hydrocarbons” “But it means burning stuff” “But it just enables the continuance of the old equipment/infrastructure”
For one, we ain’t never getting away from hydrocarbons, it’s been made a dirty word but the human body runs off them FFS. Secondly, it’s clean burning and locks carbon in a cycle, doesn’t liberate new carbon, and by large amount of use a large amount of carbon is continually pooled in storage, usefully. Thirdly yes, that’s the point, no billions of tons of CO2 dumped manufacturing flaky new tech that only sorta works, resulting in billions more tons to replace it when we get it right.
We do have economic problems with that though, natural fossil methane being super cheap, a waste product to oil really when it’s coming out of the ground, what costs a lot is schlepping it to where it’s useful, so you’ve gotta be cheaper than free, when the people who have currently conned you into paying for it, would actually pay you to take it if you’re attempting to eat their main course lunch, which is the oil. So yah, make laws about what you can and can’t do with fossil natural gas first, otherwise you’ll just get horrendously undercut and the whole thing falls apart because it’s too cheap and the right pockets don’t get lined.
With the progressive aristocratic left, any solution which does not require social engineering to work is a wrong solution ™ because solving the problem that way would mean there is no need for the aristocratic left to take over in facilitating and rationing the energy production. I.e. if it works without things like direct state subsidies, it’s a bad thing because the state has no control over it.
Basically, any solution which is good with the left needs to be dependent on the large-scale reorganization of the society in ways which demand strong central control, otherwise it doesn’t afford the opportunity to institute and maintain other social changes – namely anti- free market or “anti-capitalism” for the more naive supporters.
The easiest DIY energy storage is bagels, a bicycle generator, and human beings that like bagels and don’t mind pedaling.
Unfortunately it’s not going to be a great way to satisfy energy demand in order to run the dish washer or clothes dryer, but you could theoretically do those more efficiently by hand. You could charge a laptop or run some lights with the power of carbohydrates, and tone your calves at the same time.
The problem with that is most human food economically obtainable to the majority of average people is produced with significant CO2 output, and after you’ve burned off the 20 extra pounds you have stored unintentionally that hand power has to be fueled by extra food. Touching on that for human powered transport purposes… https://www.nature.com/articles/s41598-020-66170-y
But similar concerns apply, so unless you dig over your back yard, plant potatoes and obtain all your extra calories hyper-locally, you are probably just burning fossil fuel for CO2 out of sight and out of mind.
While true now its only true as long as transport remains so heavily fossil fuel powered, which is changing in many developed nations rather fast, and farmers keep farming like its the 70’s and the petrochemical and industrial chemical magic is on an endless tap, which isn’t changing as much or as fast as I’d like, but its hard to undo decades of what amounts to soil abuse while still growing crops sufficient to make a profit and feed everyone in the short term. Though if food waste were to be cut significantly…
The probability of a full meltdown goes up
every day.Most of the nuke power plants are now,
old worn out,dated technology ,just ticking away,
close enough to major population centers to sicken
and kill millions in a runaway meltdown event.
Many have had operational lifetime ‘extensions”
The failure mode of any nuke power plant obliviates
any argument about “costs’,its mega death lottery
capatilism that makes lignite,asbestos,ddt, pcb’s
leaded gas and smoking tobacco look good.
Properly managed and built in the right locations even old Chernobyl type designs are entirely safe, you have to really bugger up the management or maintenance significantly for anything to really go wrong, and newer designs are about as safe as you can get…
Where by your same argument on potentially dangerous stuff close enough to population centers to sicken/kill a very very vast number of things nobody ever mentions or consider as dangerous and a few things rarely mentioned like all the cleaning chemical, pesticides, fertilizers, refineries, petrol stations, airports, dodgy knockoff Chinesium electronics are often massively more dangerous to many more people as they are such a distributed and deadly thing that even if each occurrence only harmed one person it will happen to vastly more people than are going to be adversely effected should anything happen at a nuclear power station, as there are so very few of those usually a meaningful distance from population centres (certainly much further than all the dangerous that are actually in the damn houses)…
IN SHORT LIFE IS INHERENTLY RISKY, but we are not all dead yet, or all going to die to any particular danger (even a nuclear war won’t manage to kill all of us, just a very depressingly large number with the survivors quite probably wishing they weren’t…).
What matters is understanding the risks and managing how likely they are – while most nuclear plants are now getting towards the end of their life they are being treated with more than enough respect, up to full on paranoia level of attention to detail, they will operate perfectly safely (at least outside of Ukraine where it is still possible to take such care, and such a pointlessly murderous war massively trumps the damage of a nuclear power station incident in danger of death)..
What old age? There are experimental reactors that have been running since the ’50s, I know of one from the ’70s down the road here in Reno.
Research reactors aren’t generally ‘experimental’.
They are used for experiments though.
Some for ‘tope production or doping semiconductors.
Interesting fact: Some research reactors only run on near weapons grade. Navy too. Fun for the kids.
>The failure mode of any nuke power plant obliviates any argument about…
False argument. You’re begging the question that every failure of a nuclear power plant is like Chernobyl or Fukushima, conveniently forgetting that Three Mile Island also happened, had a meltdown, and then nothing happened. The public launched into screaming hysteria over a tiny fart of radioactive xenon thanks to purposeful propaganda against nuclear power, and it continues even today.
It already happens, and rather than calling it ‘rationing’, it is presented as ‘economics’… why households use off-peak power for electric water heaters. Sure you can run your heater at any time you like, but if you do run it on-peak, you pay.
Thing is, off-peak power was entirely predictable because it’s a design feature of the power system. A design choice was made not to incorporate storage, because consumers could adjust, because it was cheaper for them.
With renewable energy, when the off-peak or on-peak will happen is entirely random. Even if you can predict it a week ahead, there’s usually very little you can do about it because you can’t have a “system” to deal with it. Someone just has to buy the batteries – and of course in the end, it’s the consumers who pay for it.
It already happens, and rather than calling it ‘rationing’, it is presented as ‘economics’… why households use off-peak power for electric water heaters. Sure you can run your heater at any time you like, but if you do run it on-peak, you pay.
One thing the article did not make clear is there are two storage issues, each with different requirements.
The 1st one is Grid stabilization – smoothing the grid fluctuations caused by millions of solar cells and wind turbines coming off and online
The 2nd one is long term storage – storing surplus generated electricity for periods when generation and usage don’t match. For example at nigh when solar is not there, but everyone is trying to charge there electric car
The 1st one requires a different solution because it requires fast reaction times. One of the way it is being solved is giant motor/turbines that are powered up during peaks and generated during lows.
> Unfortunately, despite decades of research, even the most promising type of flow battery, vanadium-vanadium,
> has very low specific energy of ~20 Wh/kg of electrolyte where old-school lead-acid cells manage 25-50 Wh/kg and
> lithium-ion batteries over 200 Wh/kg. This means that the electrolyte tanks have to be quite large to match the energy
> capacity of e.g. a Li-ion or lead-acid based battery storage system.
per kg is not a good metric for stationary energy storage. watt hours per litter would be a better measurement because lithium is never going to be beat on mass (atomic number 3) unless someone develops a helium (atomic number 2) or a hydrogen (atomic number 3) battery.
Per kg is a good proxy for material costs, which is more important than how much space it takes.
I don’t see how that follows. 1 kg of lithium costs far more than 1kg if sodium.
Flywheel storage is just as energy dense as lithium ions, can be charged\discarged arbitrarily quickly, the part that stores energy can be made from simple steel (dirt cheap), and dont need to be changed out every 10 years…
Ok, so what are the downsides? For instance, steel is cheap but has a large carbon footprint at the moment.
I don’t know all the arguments against flywheel storage, but the carbon footprint of the flywheel itself has to be one of the least significant ones. The flywheel isn’t consumable. It lasts forever. What fraction of forever does it have to run to negate its own carbon footprint? Furthermore, you could make or fill the flywheel with anything as long as its balanced. Rock, sand, crushed cars, nuclear waste, E-waste…. ;)
“Flywheel storage is just as energy dense as lithium ions”
Hunh? Reference? Sure, exotic small-scale laboratory ones can get to high energy densities, but the best available commercial-scale flywheels (e.g., Beacon Power’s) are delivering 22 Wh/kg, a tenth the energy density of lithium batteries.
I worked on the development of shipping container based, lithium ion grid storage batteries. Designed to discharge 1MW of power in 15 minutes. These were most useful for frequency regulation, like what a Natural Gas peaker plant does.
The problem in the United States 10 years ago when I left the industry was 2 fold: public utility commissions, and cheap natural gas. The public utility commission in most states is who decides what costs/improvements can be paid for by passing the cost on to the rate payer; public utility commissions had a challenging time determining how these could be added to the rate base since they don’t generate. Utilities wanted the battery storage to be able to command the same rate the peaker plants were getting on the electrical grid. Natural gas was also stupid cheap. It just didn’t make business sense back then.
Biden just mandated grid energy storage, coupled with the atrocities in Ukraine, we may get grid energy storage after all.
When your hope for an engineering solution is a senile politician’s mandate, you are screwed.
Are they planning on changing the laws of physics by act of congress?