In my teenage years I worked for a couple of summers at a small amusement park as a ride operator. Looking back on it, the whole experience was a lot of fun, although with the minimum wage at $3.37 an hour and being subjected to the fickle New England weather that ranged from freezing rains to heat stroke-inducing tropical swelter, it didn’t seem like it at the time.
One of my assignments, and the one I remember most fondly, was running the bumper cars. Like everything else in the park, the ride was old and worn out, and maintenance was a daily chore. To keep the sheet steel floor of the track from rusting, every morning we had to brush on a coat of graphite “paint”. It was an impossibly messy job — get the least bit of the greasy silver-black goop on your hands, and it was there for the day. And for the first few runs of the day, before the stuff worked into the floor, the excited guests were as likely as not to get their shoes loaded up with the stuff, and since everyone invariably stepped on the seat of the car before sitting on it… well, let’s just say it was easy to spot who just rode the bumper cars from behind, especially with white shorts on.
The properties that made graphite great for bumper cars — slippery, electrically conductive, tenacious, and cheap — are properties that make it a fit with innumerable industrial processes. The stuff turns up everywhere, and it’s becoming increasingly important as the decarbonization of transportation picks up pace. Graphite is amazingly useful stuff and fairly common, but not all that easy to extract and purify. So let’s take a look at what it takes to mine and refine graphite.
Heat, Pressure, and Bugs
The story of graphite begins two billion years ago, when the oceans were teeming with cyanobacteria. Having just managed the evolutionary trick of figuring out photosynthesis, these organisms sopped up the nearly unlimited carbon dioxide from the Precambrian atmosphere, turning the upper layers of the ocean into a thick bacterial soup. When they died, mats of their carbon-rich remains piled up on the floors of the oceans, eventually becoming entombed under thick layers of sediment, where pressure and heat could begin to work their alchemy.
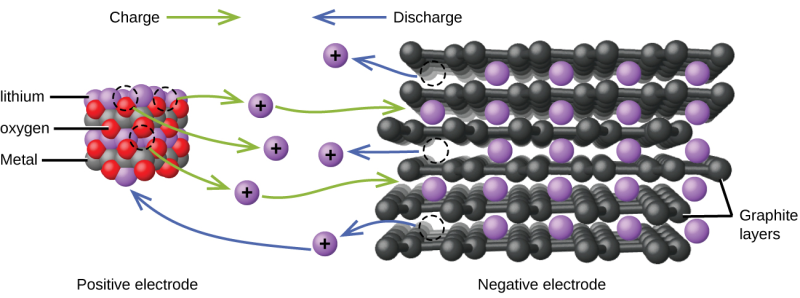
While all these dead bacteria are the ultimate source of graphite (not to mention every other carbon mineral, from coal to oil to diamonds), exactly what type of graphite is formed depends on geological circumstances. The most abundant form of graphite is flake graphite, which occurred when those dead cyanobacteria formed carbon-rich shales and limestones which were then thrust into high pressure and temperature conditions by tectonic activity. These metamorphic conditions produced rocks with crystals of graphite trapped inside them. On the other hand, amorphous graphite formed when the dead cyanobacteria formed coal deposits, which were then subsequently swallowed up into the Earth’s furnace. The heat and pressure cooked out everything but the carbon in the coal, leaving behind almost completely pure carbon.
No matter how graphite is formed, the nearly pure crystals of carbon give it unique chemical and physical properties. Graphite is a very soft mineral, often as soft as talc. It’s formed of multiple one-atom-thick layers of carbon stacked on top of each other, which leads to graphite’s fame as a lubricant. It was once thought that graphite’s lubricity came from fluids like air or water being trapped between these monoatomic layers, giving it some slip, or just from the fact that the individual sheets would shear away from each other. But current thinking is that graphite is slippery thanks to adjacent layers getting twisted relative to each other on an axis perpendicular to the layer lines; this has the effect of unlocking the nesting between layers, allowing them to slide over each other.
In addition to its lubricity, graphite has a vast number of useful properties, some of which have been exploited since antiquity. Neolithic cultures used natural graphite as a colorant for ceramics, and the mineral’s refractory properties — it doesn’t melt until over 3,600°C — have been put to use in high-temperature industrial processes for nearly 500 years, such as crucibles and molds for casting metals. Today, graphite has a bewildering range of applications, appearing in everything from pencil leads to electrodes for smelting aluminum. But the newest and perhaps biggest market for graphite is in manufacturing batteries for everything from cell phones to electric vehicles. Graphite makes up something like 95% of the anode in most lithium batteries, and it takes 50 to 100 kg of graphite to make the batteries for a single EV.
Silvery Bubbles
There are only a few places in the world where veins of pure graphite exist — Sri Lanka is the only commercially viable source of this type — and those graphites tend to be reserved for specialty uses. So, as with most commercially valuable minerals, mining and refining graphite is a matter of finding deposits with enough of the stuff to justify the effort and expense involved in liberating it from the rocks it’s embedded within. Graphite mines are usually open-cast mines, with all the usual equipment and processes — blasting to free up vast quantities of ore quickly, huge shovels and haul trucks, and crushing plants to reduce the graphite-bearing rocks to manageable sizes.
After primary crushing, graphite ore is ground to a fine powder. To release the graphite from the surrounding rock, or gangue, the particle size must be smaller than the graphite flakes within the rock. A series of grinding processes with ball mills and rod mills accomplishes this. Depending on the rock type and graphite form, acids may sometimes be used to dissolve the gangue from around the graphite. In any case, graphite is almost universally separated from gangue by some sort of flotation process. Graphite is considerably less dense than the surrounding rock, making it easy to create a froth that lifts the graphite to the top of a flotation tank where it can be skimmed off, leaving the denser gangue to sink. Flotation methods have the added benefit of making it easy to move the graphite around to different processes in the plant by pumping it.
The graphite-rich slurry from flotation undergoes a series of steps to remove remaining impurities and further concentrate the graphite. These steps include filtration, centrifugation, and separation. A spiral separator is sometimes used; slurry runs down a spiral sluice with lighter particles and water tending to run along the outside edge while denser gangue particles bunch up along the inside of the sluice. When the graphite slurry reaches the desired purity it’s dried in a kiln, screened to a specific size, and bagged for shipment.
Global production of natural graphite in 2021 was over a billion tonnes. China produces almost 80% of that, with the rest coming mostly from Brazil, Turkey, and India. North America has only one operating graphite mine at this time, at the Lac des Iles mine in Quebec. The United States has no active graphite mining operations, although there have been mines in Montana and Alabama in the past.
Hot Coke
Natural ores aren’t the only source of graphite, though. Methods for producing synthetic graphite have been around since the 1890s, and while they produce extremely high-purity graphite, it comes at quite a price. Synthetic graphite is made by subjecting amorphous (non-crystalline) carbon to enormously high temperatures to rearrange the carbon atoms into the proper crystal structure. Choice of feedstock is critical if the process is to produce high-quality graphite; the carbon needs to have gone through a “mesophase” sometime in its thermal history where most of the work of producing and aligning the basic structural units of carbon crystals has already been done. The final heat treatment is really just an annealing process that indexes the monoatomic carbon sheets into their final layered form.
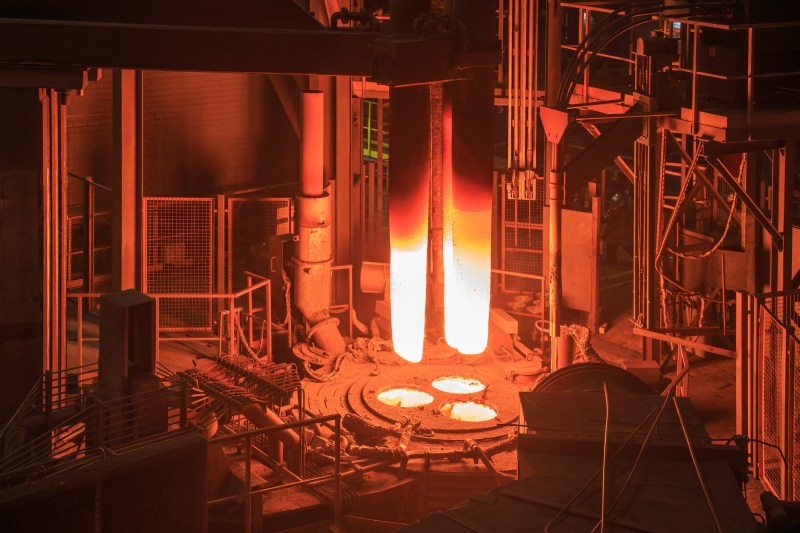
As it turns out, the perfect starting material is a byproduct of oil refining: petroleum coke, or pet coke. This carbon-rich material is basically the low-value stuff left over once all the higher distillates like diesel and gasoline have been boiled away. Pet coke is basically a solid mass of long-chain molecules, mostly carbon but with some volatile organics and a bit of water trapped inside, both of which need to be driven off by heating, or calcining, the coke in a horizontal rotary kiln. Graphitization takes place in an Acheson furnace, named after Edward Goodrich Acheson, who stumbled upon the synthetic graphite process while searching for a method to make synthetic carborundum.
An Acheson furnace is essentially an enormous carbon composition resistor: a chamber packed with calcined pet coke with graphite electrodes buried in each end. Graphitization starts when a massive current is passed through the graphite electrodes, heating the pet coke charge to 2,600°C or so. The process continues until graphitization is complete. This usually takes just a couple of days, but cool-down of the furnace and the graphite inside can take weeks. This process can be used to turn out raw synthetic graphite powder, but more commonly, it’s used to create graphitic parts like electrodes for metal refining. In that case, coal tar pitch is mixed in with the pet coke before firing, to act as a binder to give the graphite electrode more mechanical strength.
Given the expense of feedstocks and the enormous amount of energy required to produce it, synthetic graphite is about twice the cost of natural graphite. There are some applications where it makes sense, though; the aforementioned smelting electrodes are a good example, since they can be manufactured in their final shape. Synthetic graphite also tends to be better suited for use in composite materials like carbon fiber.
Whether it’s natural or synthetic, there’s no denying that getting graphite is a messy business — either we burn diesel to tear a lot of holes in the ground, or we keep refining oil so we can blast megawatts of electricity through the waste. And our need for graphite is only likely to increase, so here’s hoping that someone figures out a better way.
Great article–thanks much!
You’re welcome!
When “decarbonizing” requires huge amounts of carbon.
If only there were some reliable way to produce megawatts of energy without burning a bunch of oil oh wait we’ve had that for decades and decades and simply refuse to use it
I was going to say, they tiptoed around the fact that they’re using green energy to extract and process an entirely non-renewable resource, which isn’t very green.
Very interesting article though, I’d never really thought much about where graphite came from.
Yes, sort of, except we are able to make it synthetically, so it’s not entirely non renewable. However o hope there is another way than using petroleum byproducts. I bet that was used because there was a whole bunch of it for cheap though.
Graphite is a great moderator for reactors. You can use very lightly enriched uranium. Lots of rector designs use it in the core such as LFTR.
Sure, it’s great, except for that pesky positive void coefficient and the flammability.
There are many good ways to design a reactor. RBMK is not one of them. Most of graphite core reactors designs do not have a positive void coefficient.
There are also different grades and qualities of graphite. The Soviet Union didn’t use the best.
> While all these dead bacteria are the ultimate source of graphite
> (not to mention every other carbon mineral, from coal to oil to diamonds)
There is a growing amount of evidence that the oil we pump of the ground, especially the very deep stuff, probably does not originate in fossilized lifeforms. Best theory is carbonaceous chondrites, which are common in asteroids so can be found all over the solar system. Then heat, pressure, lather, rinse, repeat and non-fossil fossil fuels.
Coal, OTOH, is much more likely from life — especially those samples with leaf prints in them.
I don’t think it’s really a growing amount of evidence, isn’t that what’s been accepted for like 20 years?
I’ve loved the whole mining series. Thanks, Dan!
Thanks, they’re a blast to write!
Fun fact: when graphite was discovered in Cumbria (home of the famous Derwent pencil company), it was more expensive per kg than gold, and transported by armed guards.
This led to a black market in graphite – and those who stole graphite were spotted by their black-stained hands – giving us the term “black market”.
Very cool story, but the phrase doesn’t appear to have been in use before 1930
As I understand, it was valuable because it could be made into molds for perfectly round cannonballs, which of course are a huge military advantage.
— it doesn’t melt until over 3,600°C —
It doesn’t melt at all, it sublimates
Sublimes.
I was going to argue with you, but I checked and you’re correct. I learned something else new today.
What if we ‘green’ our blue hydrogen by turning the excess carbon (from the methane) into graphite?
If there are financial/tax-regime inducements then it could undercut mined graphite, which has the added benefit of cutting out the energy spent mining and refining it.
We just need to keep it out of the atmosphere for 100 years or so. What happens to all the used graphite in the end game, does it oxidise away (bad) or does it stay as microparticles (not so bad)?
we can do that with methane pyrolysis. The problem is that steam reformation works at much bigger scale and produces more hydrogen.
I machine graphite for high-power rocket nozzles. There is a reason that the rocketry community calls it “compressed filth” ;) Even with a vacuum to collect the dust I’ll end up blowing my nose black for a while. And being conductive the dust can short out a lathe motor in jig time… :(
Good luck with the graphitosis!
Graphite was noticed around Mount Monadnock in New Hampshire before 1792 and a financially unsuccessful mine was operated, probably from 1847 to 1850. There were other graphite mines in the 1840s in New Hampshire, notably at Mount Kearsarge.
Fun fact — my wife and I climbed both Kearsarge and Monadnock back in the 90s when we lived outside Boston. Anything to get away from the city. Walking up Watchusett Mountain in the spring was fun too, while the snow was still melting. Good times…
I love this series. Could you do mica some time? Is it also reformed from the ore like graphite or are big sheets carefully removed from the mine. Fun fact is that although it seems kind of flakey (thanks) it does an amazing job as a window in Geiger Muller tubes. You want the window to be as transparent to radiation as possible, which basically means being as few atoms thick as possible, but also robust in the field and able to sustain the partial vacuum within. I’ve fired up old Geiger tubes that are decades old and not yet found one that had leaked air in.