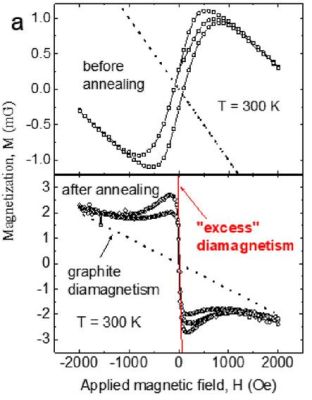
Little has to be said about why superconducting materials are so tantalizing, or what the benefits of an ambient pressure, room temperature material with superconducting properties would be. The main problem here is not so much the ‘room temperature’ part, as metallic hydrogen is already capable of this feat, if at pressures far too high for reasonable use. Now a recent research article in Advanced Quantum Technologies by Yakov Kopelevich and colleagues provides evidence that superconducting properties can be found in cleaved highly oriented pyrolytic graphite (HOPG). The fact that this feat was reported as having been measured at ambient pressure and room temperature makes this quite noteworthy.
What is claimed is that the difference from plain HOPG is the presence of parallel linear defects that result from the cleaving process, a defect line in which the authors speculate that the strain gradient fluctuations result in the formation of superconducting islands, linked by the Josephson effect into Josephson junctions. In the article, resistance and magnetization measurements on the sample are described, which provide results that provide evidence for the presence of these junctions that would link superconducting islands on the cleaved HOPG sample together.
As with any such claim, it is of course essential that it is independently reproduced, which we are likely to see the results of before long. An interesting part of the claim made is that this type of superconductivity in linear defects of stacked materials could apply more universally, beyond just graphite. Assuming this research data is reproduced successfully, the next step would likely be to find ways to turn this effect into practical applications over the coming years and decades.
Wait until April 1’st with publication, just in case 😸
Entertain a bore please, what are the actual uses of an ambient conditions superconductor that is too expensive to use for power transmission lines? Novel magnetic circuits?
Cheaper MRI scanners for one thing – hopefully.
Could mean cheaper or even portable NMRI machines
It’s tricky. Many superconductors lose their superonductivity when passing large currents. . If you want to make superconducting magnets for your MRI machine, for instance, that matters. And so far, the large current superconductors all require very low temps.
This doesn’t look like it’s going to be a power conductor. Which is _not_ to say that no-resistance for small-signal circuits at room temperature wouldn’t be revolutionary. Just a different problem solved.
I’d suspect room temperature superconductors would be useful for all sorts of electromagnetic sensors and signal cabling but the obvious use for them would be to separate audiophools from their money, can you imagine how much they’d pay for a ‘superconducting interconnect’?
Well, there’s taking existing normal things and making them better by removing the losses you normally assume are unavoidable. Antennas that are much smaller than the wavelength you use them with are normally very inefficient. But if there was no resistive loss, then you could actually use small antennas. Or arrays of them, if you don’t need to take up less space but you could benefit from high directionality or beamforming. Or you could have antennas for more frequencies. I imagine you can also put smaller quantities of the stuff into various parts of the analog signal processing chain, not just the actual antenna.
All sorts of things with signals, really, could be done differently if you didn’t have to worry about imperfect conductivity and there was something with perfect diamagnetism, even if the allowable power density was too low to afford enough of the stuff for so-called “power” applications. There’s various electrical and magnetic things that only make sense to do if you have superconductors to use – I see something about a London moment, where a spinning superconductor makes a really great gyroscope. It’s hard to think of some of them, because I generally look more for applications of things we do have instead of things we don’t.
They can be used for RF filers. Theoretically a communication satellite could support receiving more channels at once. Letting you support things like ring service and other low bandwidth protocols for many sat phones at once (10’s of millions maybe?)
For transmission lines anyway, superconductors aren’t that useful unless they can handle pretty high current densities. A superconductor ceases superconducting f the amps per square millimetre gets too high, due to magnetic effects, so even with zero resistance, the wires may ned to be pretty thick, at which point why not just use a normal conductor thick enough to have an overall low enough resistance per unit length despite the higher resistivity of the metal material itself.
“as metallic hydrogen is already capable of this feat”… LOL.
We are not doing that again.
Yes, we are. Again and again and again and… that’s science.
…and expecting the same results. That’s madness.
First, it’s expecting different results, not the same. And second, good thing the Wright Brothers were mad then. Please do share the percent of scientific breakthroughs that succeed on the very first try.
Funny you should say this, because I’m betting you’re reference the LK99 fiasco: but this isn’t the first time highly-oriented pyrolitic graphite (HOPG) has been claimed to be a room-temp superconductor either!
I think I first saw it mentioned back in 2011:
https://www.nature.com/articles/nature.2012.11443
but there have been other claims periodically ever since:
https://arxiv.org/abs/1301.4395
This article *actually* came out 2 years ago and has likely been cycling through peer review.
Hmm.. room temp 25C = 375K so 300K = -75C NO nice temp for a living room ;)
You might want to run those figures again.
25C is 298K. 300K is 27C or 81F. Agreed, not a nice temperature for a living room. Unless there’s a power outage in January…
It’s kinda hilarious that 300K became de facto “room temperature” in most of physics, but that’s what rounding does for you when human livability is confined to maybe something like ~30 temperature units and climate control is only about 10.
It semi-sorta matters for radio frequency stuff because the noise you see *is* from absolute temperature: it’s kinda hilarious to see people bounce between 290, 293, 298, 300 K because they can’t figure out whether to round in Celsius or Kelvin and how many digits to round to and *none* of them (63, 68, 77, 81 F) are actually the most common room temperature (72 F, or 295K).
room temperature to one significant digit. That it is within 10% of room temperature should be considered a bonus. fwiw, 297K is about ideal to me, but I keep my winter thermostat set to 293K.
The issue is that for radio frequency stuff, “room temperature” is used as a reference when quoting noise figure. And they give those values to *plenty* of significant digits, but a lot of times they don’t even *tell you* what their definition of room temp (and therefore noise figure) is.
Now, you might say “hey, they say measurements are at Ta=25 C, so a 3 dB noise figure is relative to noise at 25 C.” Maaaybe? Except wait, now you see other plots with noise figure versus temperature, so surely they don’t mean with reference to *that* temperature?
And then you go and look through documentation from the manufacturer about calculating noise figure, and… huh, 290K one place, 300K another place… It’s a full-on mess.
(for reference, the ‘official’ – as in, IEEE – definition of noise figure uses 290 K as the reference temp)
definitely run it again… 0K is -273C so 300K is more like 27C, which is 4C higher than my current room temperature with the central heating on.
Apart from the calculation error, the other thing that’s related to temperature is that the temperature you get a superconductor to work at really doesn’t matter until it’s high enough that it changes how you can use it.
If you can only use it around liquid nitrogen temperatures, you need to buy or produce liquid nitrogen to use it. If you go far enough above that, your cryocooler might only need to be enough for say liquid methane temperatures. If you can get one to work at the temperature of dry ice, you can use it temporarily with dry ice and permanently with a fancy enough freezer. If you can get it to work around negative 40 degrees, you can use it with a pretty normal freezer. If you can get it to work at above the freezing point of water, you can use ice or any freezer. If you can get it closer to room temperature, you may only need air conditioning to use it, or air conditioning combined with for instance a peltier cooler or a really tiny refrigerator. If you can get it to work at greater than indoor temperatures, you can not only use it indoors, but use it even inside a device that may be warmer than the ambient indoor temperature. Or maybe outdoors, depending on the weather. If you can get it to work at a warm enough temperature, you can start integrating it into various electronics even despite the waste heat they produce, with just a regular heatsink.
“the temperature you get a superconductor to work at really doesn’t matter”
This isn’t really true. If you look at superconductors on a phase diagram of magnetic field/temperature, as you get lower and lower below the critical temperature, the critical *field* rises, and then typically saturates.
Obviously the saturation value of that critical field (the critical field at absolute zero) matters, but all else being equal, higher critical T means that for a fixed temperature you get a higher critical field. Even if you *did* get a “room temperature superconductor” there’s a very good chance you’d still want to work with it at very cold temperatures, just because it works better.
(you might note in the diagram above that the high-field case shows an *increasing* critical current with temperature, which is anomalous and explained in the paper as indicating that the behavior is occurring through an array of superconducting regions – e.g. Josephson junctions).
Here’s the part you cut out “until it’s high enough that it changes how you can use it. ”
My point is that if one reaches 297K and one reaches 298K, but the 297K has much better properties in other respects, then the extra degree doesn’t matter. But if you find one whose critical temperature is 350K, even if it’s worse than the other two in all ways except temperature, it’s still worthwhile because of the higher threshold opening up new opportunities to use it.
Even a pretty minimal performance cap is still usable for at least something, whereas requiring cooling that you can’t provide is a dealbreaker. I’ll agree with you that we’d want to provide cooling almost anytime we could, but we’re not putting LN2 in cell phones.
With so much excitement around superconductors, I’m surprised we don’t have everyday applications of just regular low temperature ones.
It’s not like making stuff cold is all that hard, I don’t see why consumer grade cryocoolers couldn’t exist, or even peltier effects devices that just cool a micron sized junction with vacuum insulation.
Not as cold as these are, unless you’ve seen better – the superconductors I know of that can be warmer than dry ice are only the ones that operate at high pressure. And dry ice is already below what people get with peltier chips, though there’s been DIY cryocooling articles on this site that show ways to get into that temperature range.
On the paper, the “superconducting” effect collapse above 0.1mA. I don’t think it’s going to be that useful, unless you stack hundred of thousand of such “conductor” (and even then, the accumulated magnetic field might still collapse if above 500 Oe).
So it’s a nice material for studying but it’s clearly not useful in practical life.
I mean, there’s other things in daily life than power transfer. If you can drastically improve how well a cell phone’s wireless receiver works, that sounds useful in practical life to me. Or if you can make really good sensors, like gyroscopes or something, and stick them inside gadgets, that’d be nice too.