Telsa are one of the world’s biggest purchasers of batteries through their partnerships with manufacturers like Panasonic, LG and CATL. Their endless hunger for more cells is unlikely to be satiated anytime soon, as demand for electric cars and power storage continues to rise.
As announced at their Battery Day keynote, Tesla has been working hard on a broad spectrum of projects to take battery technology to the next level in order to reach their goal of 3 TWh annual production by 2030. One of the most interesting aspects of this was the announcement of Tesla’s new tabless 4680 battery, which will be manufactured by the company itself. Let’s take a look at what makes the 4680 so exciting, and why going tabless is such a big deal.
Roll Up, Roll Up
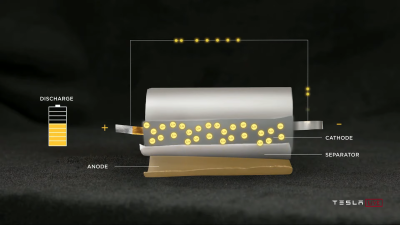
Tesla are somewhat unique among electric car makers in that they’ve resolutely stuck to using cylindrical cells in their battery packs, where other manufacturers have largely used prismatic designs. Beginning with the venerable 18650 popular with laptop manufacturers and flashlight builders, Tesla later moved on to using larger 21700 batteries, with the bigger form factor meaning each cell had greater capacity. To construct these cells, long, thin sheets of anode and cathode material are laid on top of each other with a seperator material in between, and then rolled up into a “jellyroll” to fit inside the cylindrical body. The anode and cathode each have a small tab, generally at the center of the rolled up sheets, which pass power to the terminals on the outer case of the battery.
These small tabs hold back cylindrical cells in a multitude of ways. They act as a bottleneck for current flowing in and out of the cell, as despite the huge area of the anode and cathode, all current flowing in and out of the battery must go through a pair of tabs just a few millimeters wide. Electrons from the outer areas of the jellyroll must travel a significant distance to reach the cell terminal, with an electrical path length up to 250 mm in 21700 cells. This greater path length means more resistance, with a corresponding effect on thermal performance. Additionally, the tabs frustrate efforts to effectively produce anode and cathode sheets at speed, with production machinery having to stop and start repeatedly to deal with the protruding features.
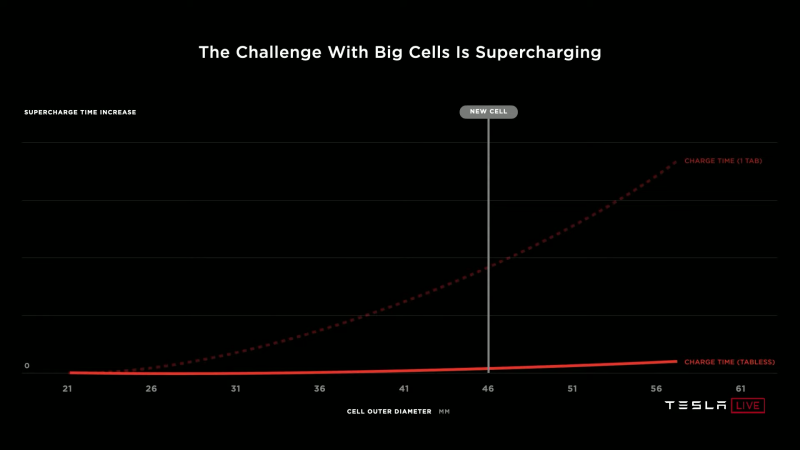
Tesla had previously made gains in performance when shifting from 18650 cells to the larger 21700 design, but efforts to further increase cell size hit a brick wall. While larger cells can store more energy and deliver cost savings in production, thermal issues meant that charge times and discharge rates would be negatively impacted. Larger cells meant longer path lengths, with the higher resistance meaning less power output per cell and slower charging. Even with Tesla’s fast charge technology, many still consider electric cars to charge too slowly, so this was one tradeoff that wasn’t worth making.
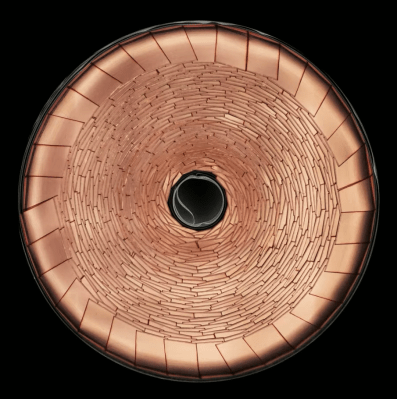
Enter “tabless” batteries. Rather than having a small battery tab attached to the anode and cathode respectively, the entire anode and cathode foils are laser-patterned and processed to have what are essentially many tiny tabs all along their length. Replaces the step of manually attaching separate tabs later in the manufacturing process.
When the anode, cathode and separator are all rolled up together, these many smaller tabs flatten out to form a “shingled spiral”, creating a much larger contact area between the active battery material and the casing. This means that the path length for electrons to travel is much reduced; Tesla quotes as much as a 5 times reduction compared to previous designs. This is due to the fact that electrons can now move directly towards the battery terminal, rather then having to take a more roundabout path to the center of the sheet first to reach the single-tab connection.
The end result is the 4680 cell, named for its 46 mm diameter and 80 mm length. This is a departure from the five-digit nomenclature, but nobody at Tesla could figure out why 18650 cells have the trailing zero, so the company eliminated it in the new cell’s designation. The new cells are claimed to contain 5 times the energy earlier designs due to their larger size. Even better, Tesla claim they can deliver up to 6 times the power, due to the reduced electrical path length of tabless construction enabling better thermal performance. Estimates are that the switch to 4680 cells in Tesla’s automotive packs could result in a range gain of as much as 16% — an impressive number given the automaker’s already impressive numbers in this area. For example, the upcoming Model S Plaid claims to have a range of 520 miles using the 4680 cells.
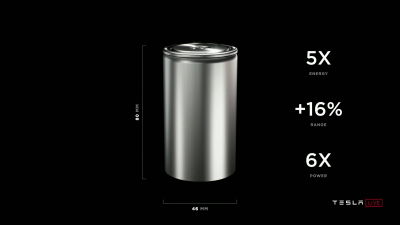
The new design brings production benefits, too. By laser-patterning the anode and cathode for direct connection, rather than attaching separate tabs, the materials can be handled through continuous roll processing, not dissimilar from techniques in paper production. This promises huge gains in production speed, with machinery able to move at continuous high speeds rather then having to continually accelerate and decelerate for tab attachment to each anode and cathode sheet. Tesla’s goals to produce more batteries to meet demand are not practically achievable with current production techniques, so improvements in processing and production speed are key to solving this problem. This will also lead to reductions in cost, which is a major part of the company’s effort to produce a more accessible electric vehicle at the $25,000 price point.
These new cells, with their greater energy density and high power output, would appeal to a huge market of hackers and makers. However, the continual theme throughout Tesla’s keynote was that they simply can’t source enough batteries to meet their needs as it is. We suspect that it will be several years before tabless batteries hit the open market, as Tesla keeps its entire supply for its own internal use. Other manufacturers will likely scramble to develop similar technology due to the gains on offer, but this will take time, and in the meantime, individuals wanting the best cylindrical cells will have to wait for new Teslas to show up in their local wrecking yards.
The tabless technology announcement was just one piece of Tesla’s Battery Day announcements. Work is ongoing to make gains in other areas, such as anode and cathode chemistry, and production techniques, in order to meet the company’s lofty goals of increased battery production to meet worldwide demand. If electric transportation and Powerwall grid storage are to truly change the world, projects like these will have to pay off — otherwise we simply won’t have the batteries to put in the cars!
Thanks for the plot with no y axis. :(
It’s a relative comparison, so that doesn’t matter.
https://imgur.com/JWdA2TL
Right. Labels don’t matter at all :)
Sure there is, it’s just not labeled!
B^)
There is an y axis. It is labeled “Supercharge time increase” and has one tick (“0”) on it. And some very faint horizontal lines that hint that (but do not confirm) we are looking at a linear and not a logarithmic scale…
The wider “tab” should greatly help with cooling the cells as well, faster charging?
Yes the wider tab (tabless ;) will help with heat in two ways… firstly there will be less heat generated via resistance in the jelly roll as the electrons only need to traverse ~40mm to the tabbed end rather than ~600mm on the larger roll format given a centre tapped jelly roll. Secondly the copper bottom and presumably similar aluminium top conductors will act as a rapid heat pipe out of the battery; aluminium and copper also having excellent thermal conductivity. The thermals will be so great that the efficiency of the battery pack will be improved while the cooling requirements will be significantly reduced.
From what I understand, the cells shouldn’t get as hot during charge and discharge due to the lower resistance (since the electrons don’t have to travel as far). Heat is a limiting factor of charging and discharging (ignition is bad) so it will decrease the time required for both while retaining a similar thermal output.
The change in current flow direction seems almost too obvious. Was there a seperate innovation which enabled this construction method, or is it just a case of nobody trying it before?
Dave has explained everything in detail as well :-) https://www.youtube.com/watch?v=hbPKE62aM0U
thanks! Q
Has Tesla looked into Kilowatt labs series supercapisator that charges in seconds?
The funny thing with having a “wide tab” like this is that it honestly is nothing new at all, and has existed on the market for a rather long time.
And this is true for both capacitors and batteries alike.
Though, there is honestly little reason to go with a continuous tab like this, going from the traditional single tab to one at either end more than doubles the cells performance, without making a headache out of folding the tabs into a package. But having more than two is of course still advantageous.
But I am curious to if a nearly continues one is actually advantageous, or if it only add more copper bulk at either end than what it adds in performance. Diminishing returns is after all a thing. And if it only adds weight, and lowers density, then it isn’t really a worth while thing to “waste” copper on…
Ie, in the end, how often should one have tabs, and how wide should they be for optimal perfomrance?
Though, to be fair, electric cars should make more use of super capacitors regardless, due to their much lower charge/discharge losses, and far higher power density. But capacitors obviously shouldn’t outright replace batteries, just complement them for acceleration and re-gen braking.
I’ve wondered whether super-capacitors might have been good as a “cache” between the batteries and the engines for both spiky regenerative-braking / acceleration. But given the performance of this new cell design you get all the benefits of the cache with a highly simplified cell / pack structure.
The copper plate created by the tabs on the bottom and the aluminium plate created on the top act as a heat pipe as well is a very low resistance cell. Cooling will be significantly reduced / simplified.
That “accelerating” tab design in their patent is what made this work where others had failed. They don’t have the tabs cut at liner spacing rather the tab spacing curves upwards (when graphed) to create “flat” tab-ends as opposed to the convex bump you’d have otherwise.
Oh so much magic… :)
The problem with capacitors is though their very steep discharge curve compared to batteries.
So it will practically have to be a separate bank, with a bidirectional DC-DC converter in-between.
Though, doesn’t stop us from adding some regular 450 Vdc aluminium electrolytic caps to smoothen things out a bit.
And yes, the increased amount of tabs on the battery itself would help keeping it cool.
But so would a super capacitor bank as well. (Since with such a bank, the battery never really needs to see any huge power draws. (Charging is though still an “issue”, but fast charging is bad for batteries due to more than just temperature related things.))
Just wait until someone manages to make capacitors in the GF range.
I don’t think I’d like to sit atop that.
But batteries already do everything that is needed in terms of surge current, due to how large our batteries are. We don’t have motors that can handle 2000A anyway. With motors and batteries already perfectly matched to get sub-3 second 0-60 times (with the newest most expensive cars now sub-2), I think supercapacitors for cars are just a waste. Maybe for Formula 1e racing or something, but not for the average consumer.
In other words, although what you say is true, it’s unnecessary for consumer vehicles, and the small (tiny?) benefit isn’t worth the complexity or cost.
Until you look at the manufacturing costs of batteries.
The environmental impact of that production.
And the increased weight of the vehicles, that contribute to an increased road wear.
Now, some companies doesn’t care about such details.
Koenigsegg for an example discharge their batteries up to 60C, a rather stupendous thing to do as far as discharge efficiency is concerned. They if anyone should have used capacitors. But they didn’t….
Now, capacitors could give many kA of output currents, and easily hundreds of kW of power.
But that is as you say, “unneeded”, and I agree.
But to get any meaningful amount of power out of a battery, it needs to get rather huge.
And that discharge will also be fairly inefficient.
Batteries have good efficiency at low discharge rates. Going above 2-2.5 C for most lithium based batteries is like climbing mount Everest. One do get plenty of power, but plenty more is burned as heat in said battery. And that is literally our range getting turned into said heat.
A small capacitor bank can keep those peak power draws down at much more reasonable levels, ensuring that we aren’t wasting range for acceleration.
While also being able to reduce overall weight of the vehicle, not to mention price.
A bidirectional DC-DC converter isn’t all to hard to develop nor build, and if it is bottlenecking our capacitor bank is largely a non issue. Since our capacitor bank needs about the same amount of energy as our vehicle would have when traveling at “typical” highway speeds at max rated weight.
Also, in terms of output current, our capacitors would most logically be in series, so they would likely only provide a few hundred A or low kA at most, though at likely 200-500 volts. (And this isn’t all that unreasonable, the Tesla Model S for an example has a total of 615 kW of motors, running at only 400 volts. So that is 1537.5 A, not account for drive train losses, so peak current is higher.)
And that car uses typical 18650 cells.
This is configured as 74 cells in parallel, 6 of those sets in series to form a group.
And 16 of those groups in series to form the complete battery pack.
This means each cell needs about 4.17 volts each for our total of 400 volts. (Though, that 400 volt will drop as the batteries discharge. Requiring even more current to get our 615 kW power output.)
But it also means that our 1.5 kA is only shared between 74 cells. Or 20 amps per cell when fully charged.
If we are generous and say that these 18650 cells have 3 Ah of capacity, then they are discharged at 7C, quite a bit above 2-2.5C where discharge efficiency is already crawling up the wall….
Now, most people do not put the pedal to the metal, but that 7C is for a fully charged battery.
But lets say that we have a more modest 3.5 volts left in each cell.
Only use 2C (6 amps) from them. Then we get 150 kW of power.
If our drive train is magic and is 100% efficient, then it will take us 11.6 seconds to go from 0-100 km/h. (0-62.5 mph)
And few people are that easy on the pedal when driving a Tesla…
In the end.
Batteries are wonderful for energy storage, but providing power is something they are lack luster at doing efficiently.
>”With motors and batteries already perfectly matched to get sub-3 second 0-60 times ”
Well, “perfectly”. Tesla “ludicurous mode” only works when the battery is full above a certain charge threshold, because they’re banging against the discharge current limit of the cells. They got into a lawsuit in Norway for false advertising about the acceleration because a Model S will drop power when the battery isn’t fully charged.
If the fast charge/discharge inefficiencies are inherent to the chemistry, then the heating is inevitable and limits the charge/discharge. If conductor losses are the primary cause of heating in fast charge/discharge, Tesla’s improvement makes heating 1/6th the problem it was up to now, and the use of supercapacitors provides no improvement at increased cost and complexity.
To support a model 3 (lowest end) 210+kW full throttle output for 5 sec, we need almost 300 Wh supercapacitor (>20kg), and it deliver 90% efficiency. Compared to running the built in battery at 4C for 5 sec, also give around 90% discharge efficiency in single tab battery. When battery itself is large enough, adding a super cap don’t worth the effort if the “peak” current is within reasonable consumer car specs.
Supercaps are a useful add to a battery pack, they have a much lower ESR and ESL than a battery. However, their energy density is much, much lower than a battery. The power density of a supercap is high because of the low equivalent series resistance, and so is very helpful to cover those short term bursts of current. For example, the biggest supercap cell from Maxwell, on the DigiKey website, is 2.85V and 3400F. Pretty Big. But, putting that in battery units, it is a 1.4Ah battery. It’s bigger than two D cells, 60mm D x 140mm L. Not a great battery. But it can deliver a burst of 2000A without damage.
But batteries already do everything that is needed in terms of surge current, due to how large our batteries are. We don’t have motors that can handle 2000A anyway. With motors and batteries already perfectly matched to get sub-3 second 0-60 times (with the newest most expensive cars now sub-2), I think supercapacitors for cars are just a waste. Maybe for Formula 1e racing or something, but not for the average consumer.
Yes. Super capacitors are a bit mediocre when it comes to energy density.
Not a technology that will replace batteries in most applications.
Though, they do compliment batteries very well due to their high power density.
Thereby reducing peak current draw from our battery bank, since the main power needed for acceleration would come from our capacitor bank.
Same for re-gen breaking, there we can stuff the energy back into our capacitor bank. And due to the exceptionally low charge/discharge losses, then we can get a bit more range.
Just removing the acceleration related load from our battery bank will likely increase our range by itself. After all, battery chemistry itself isn’t all that impressive when it comes to its ESR compared to capacitors. (Adding on more tabs only reduces conductive losses, it doesn’t do much for our chemistry related losses.)
But I have also seen super capacitors that aim for energy density tend to be a bit less impressive in terms of power density. Since they have fewer tabs, and more tabs takes more space. (And more tabs also increases costs, and energy/dollar is also a thing.)
I have looked a bit at a bunch of capacitors and found energy densities around 35 kJ/kg with decent power density (about 4-8 kW/kg) as well, so a 2 ton car moving at 100 km/h would have the same kinetic energy as we could store in about 44 kg of capacitors. (or about 175-350 kW of power delivery. (240-480 hp)) And our batteries can still supply a bit of power as well, though preferably within the region where it retains good discharge efficiency.
But obviously we would have a bit larger capacitor bank, so that we never discharge it down to low enough voltages were it becomes hard to deal with. (A 2.5 V 500F cap for an example stores only 250 J for the first volt, while the next 1.5 volts stores 1312 joules (for 1562 joules total), so discharging down past 1 volt is mostly giving us negligible more energy, but at an increased DC-DC complexity cost. (Where exactly we put that line is though a different question, and 2.7 V or 2.8 V capacitors is also an option on the market.))
Supercaps are also pretty bad when it comes to self-discharge, crash safety, fire safety…
Any capacitor that stores significant amounts of energy in an electrostatic tension is basically a bomb. You break the insulator, it goes bang instantly.
Yes, capacitors does have a fair bit of self discharge, compared to batteries.
But it is still slow enough to not be particularly noticeable over many hours.
In terms of crash and fire safety, they do indeed pose a threat, but so does batteries.
The main reason batteries tend to not explode when impaled is due to contact resistance between the shrapnel and the conductive sheets within. Typically leading to self fusing around the intrusion. (After all, the intrusion isn’t going to make all that good contact with the sheets, unlike the tabs on the battery that has a significantly larger contact area.) Same is true for capacitors.
In regards to fire, batteries aren’t really all that safe either….
Not to mention that both battery banks and capacitor banks can have protective casings around them, that protects against physical harm, and also against some fire as well.
Though, even regular fuel tanks on normal vehicles have their own risks during a crash.
Sure, but over days and months – if you’re keeping any amount of energy in the supercapacitor then you’re losing a bunch. When the car is parked, the capacitors drain empty, so if there’s a kilowatt-hour in there, you lose a kilowatt-hour…
As for puncture safety, capacitors are fundamentally different in the sense that the discharge is nearly instantaneous, and it is not limited by chemical reaction rates because it’s electrostatic. A very large very high capacity capacitor is a touch-and-go situation. A small failure may fuse, a larger failure will vaporize the material in a flash and explode the capacitor entirely. Batteries don’t do that (yet).
What you need to understand is that a if the separator breaks down in a chemical battery, the electrical contact is provided by whatever is piercing the separator, if it’s still present. In an electrostatic capacitor, the different charge carriers are physically attracted towards each other through the breach and the entire thing will drain like popping a party balloon.
That’s why storing large amounts of energy in electrostatic capacitors is a stupid idea; as an energy storing medium it’s like building a gigantic watch-spring out of ultra-high tensile strength steel and hoping it won’t snap..
When turning off the car, it should preferably move the energy stored within it into our battery bank. It could wait with doing this in case we suddenly decide to start the car within the next little while.
But typically, the discharge of capacitors isn’t remotely “fast”, I have left a 500F “ebay special” super cap at 2.5 volts for over a month without loosing more than 0.1 volts on it. So self discharge is not something I would worry too much about. (And it is a super cap, it gives out far too much power to be a battery. And have way too linear charge characteristics under constant current charging for it to be a battery.)
Also, the capacitor bank wouldn’t need to be all that huge to be fair.
Since we don’t need it to store more than the equivalent kinetic energy of the car when fully loaded and traveling at a “reasonable” speed. Like 100-130 km/h (typical highway speeds world wide is around that figure).
For a 2 ton car, that would only equate to 2.6 MJ of energy, or about .72% of a kWh. (2 tons moving at 130 km/h.)
So a 1 kWh capacitor bank would only be needed for rather extreme scenarios, especially considering that some of the energy used during acceleration can still be provided by our battery bank. (Same story for re-gen breaking. The capacitor bank just handles the majority of the power.)
In terms of safety though.
Considering the very thin conductive layers making up the capacitor, it would make fairly poor contact with any intrusions. This is the main reason lithium batteries at a lot of times doesn’t care about having a nail or two hammered into them. The sheets around the intrusion self fuse at the intrusion, by simply vaporizing away.
Though, that is for a nail. I haven’t seen any tests with a large knife edge being forced through the device. Since that can potentially lead to less current density in the sheets due to a larger contact area, something that could make the device fail a bit more spectacularly.
Have a look at http://www.kilowattlabs.com and especially the youtube videos on the safety aspects. They are also quoting 70 Wh.kg energy density. I have seen several of these in operation in residential applications and they compare well against the Tesla Wall, except in ergonomics :-). But they will outlast the Tesla and retain full performance…
>it would make fairly poor contact with any intrusions
Again, the separator in a capacitor doesn’t do the same thing. In a li-ion battery, the separator conducts electricity. In a capacitor, it specifically does not conduct electrically and isolates the two halves of the cell.
Breaking the insulator, the hole itself leaks charge, which causes the capacitor to self-discharge quickly. The electrostatic discharge is not limited by the rate of the chemical reaction like in a battery – all the charge just rushes towards the hole in the insulator. If the energy density of the capacitor is high, a plasma arc forms that empties the capacitor in a flash and vaporizes the materials, causing an explosion.
Someone once said, “The best part is no part”. I bet someone who said that would have thought about these points in really great detail. Maybe even made analogies to the rocket equation while considering cost, part weight and power density. Can’t imagine someone like Elon would have heard of that though…. ;-)
Part count reduction is indeed a thing that can decrease system complexity and reduce price.
Though, since individual components seldom behave/perform ideally for one’s application, one needs to find complimentary components that together achieve a better job than any of the two does alone.
Batteries for an example dislike putting out tons of power for a given capacity, but they do have rather desent energy density.
While capacitors have lack luster energy density. But they on the other hand doesn’t mind putting out literal kW for every Kg with minimal losses.
So the two tends to compliment each other rather nicely for when we want short but very high power pulses. Like accelerating a car.
Downside is that they have wildly different discharge characteristics, so one or both needs to be having some DC-DC converter. (And for a car, that DC-DC also needs to handle re-gen breaking, but that can be a second DC-DC, but a bidirectional one isn’t actually all that hard to design and build. (Especially if one has synchronous rectification, then one practically has a bidirectional one, but only lack a suitable controller.))
The biggest issue is the power produced by regenerative braking. It does not matter how fast a vehicle can achieve 60mph as most decelerate roughly at same pace on road legal speeds. Super cap buffers could sort this out. The issue is even more acute when adopting electric drive to trucks. Electric engines in trains solve this by feeding the power back to the conduits.
Luckily enough.
Capacitors also do not mind sucking in power just as easily as they can give it out.
Unlike batteries that sweat if one charge them much faster than 3C.
Even if they “happily” discharge at 7C.
Also, the capacitors have much lower charge/discharge losses compared to batteries.
With that, re-gen breaking can have an increased efficiency, and be more viable in practice since it can increase the range of our EV in city traffic where starting and stopping tends to be rather common, instead of just burning it resistively, be it through resistors or friction.
I don’t understand why capacitors matter here?
Regeneration inefficiency isn’t in storing the power, it’s in generating the power. Converting the mechanical motion to AC through the motor, then rectifying it to DC is where the inefficiency is.
Right now, vehicles tend to regenerate at about 1/3 to 1/6 the maximum draw rate. The Bolt has 70kW regen and 160kW maximum draw. In the case of Teslas, they regenerate (77kW) at about 1/4 of supercharging rates (250kW).
In other words, it’s easy to store the power in the battery – that’s not where the limitation or inefficiency is – switching to a capacitor won’t change this.
If regeneration was 10x the power, then yes, you’d have a point. Since both the draw and storage capacity scale with battery size, this won’t change for larger vehicles either.
Musk talked about how the line needs to stop to deal with the tabs, a shingled design like this avoids that, and so it lets the line move much faster as it doesn’t have to deal with acceleration limits.
In addition, the continuous tab will make much better thermal connectivity, which will also help the cells not overheat.
Supercapacitors can actually replace batteries totally in EV’s, once they improve the energy density further, which is expected within a few years – possibly even three years. It is already used in forklifts and buses; however in buses they are too small for good range and are charged for a few seconds at every stop. I am actually using a supercapacitor AA battery in my computer mouse, and it works faultlessly and charges quickly. They offer more than a million cycles without degradation and have none of the safety issues of lithium batteries, while being able to work at high temperatures without cooling. At the same time supercapacitor batteries offer an RTE of 99% DC-DC.
I suspect all the extra copper at the ends earns its keep. It lowers the overall electrical resistance significantly. The resistance reduction might seem minuscule until you consider the very high currents involved. Additionally, the extra copper decreases the thermal resistance a lot. So performance is improved in three ways. First, the heat produced during charging and discharging is reduced, because lower resistance = lower dissipation. Second, the heat that IS generated can get out of the cell more effectively because of the lower thermal resistance. Third, the lower heat actually RESULTS in lower resistance, because the resistance of (most) conductors increases with temperature. All of this means less wasted power, faster charge times, a few extra miles when the charge is getting low, and a significantly higher power density.
So when and where can we order them for our projects?
It would be awesome if these hit the open market! Would love a set for my rover.
Telsa are one of the world’s biggest purchasers of telecom equipment?
yeah, funny, the first word being a typo, good way to kick off…
I heard the trailing zero means cylindrical.
In retrospect, the CR2032 is 3.2mm thick, perhaps the convention was just to measure height in 1/10 of a mm.
Yes, I think that was exactly it. It’s really bizarre that they said that they couldn’t figure it out when it’s plainly obvious based on sub-10mm cells, and even a quick Google search…
https://en.wikipedia.org/wiki/Battery_nomenclature
Quote:
After October 1990, round cells are systematically identified with a number derived from their diameter and height.
/Quote
If Tesla (empoyees) can’t read wikipedia he’s maybe less smart then I thought.
For all those button cells using tenth of a mm resolution makes sense, but deviating from this standard just because the cell is a bit bigger does not, at least not to me.
Discussions about this are just more free advertisements for tesla. that may well be the biggest reason for doing it.
I’m also surprised this reaches hackaday. For some weird reason it seems a hype to post about this complete non issue about batteries, which has been used in foil capacitors and also in high discharge batteries for years and years. It’s likely that even paper wound capacitors of 80+ years old were made this way.
these are giant steps in battery technology, i love to read that here on hackaday
Meh. My take is it’s more of a manufacturing cost/time reduction than anything else. For example the claimed energy storage increase of 5x and power increase of 6x is not impressive when you realize the volume of the cell increased by 5.5x. Looks like energy storage density dropped, probably due to the loss of volume at the ends of the cell.
Also I’m not convinced of the supposed thermal improvements. Sure, it seems like it would conduct heat away more quickly, but if it doesn’t significantly change the amount of self-heating in the cell, then it doesn’t change the amount of heat you have to deal with. It still has to go somewhere, and getting the heat from inside the cell to the terminal is only a small part of that. Basically all it does is solve a problem created by increasing the size of the cell.
I’m not drinking the Tesla kool aid in other words. They have a great marketing team, but there’s no reason to think that they accomplished some kind of incredible feat that no one else was capable of.
What would be bigger step is to allow cooling using the center hole in the battery.
I know liquid an battery usually didn’t goes well together, but why not use a non conductive fluid (better than air, that is) to cool the cells from the ‘inside’.
When you stack the batteries in series, it will naturally creates a duct. so you can cool the cells from outside and inside ; it would be much better, no?
Grey – yes, it does change the self-heating within the cell – which is a factor of resistance. As described in the article and Tesla’s presentation, since the path the current has to travel is significantly less, there’s significantly less resistance, and significantly less heat generated.
@Telek
Understood. With a single tab design, current flows from the furthest layer all the way around to where the tab is connected. With the continuous tab design, current only has to flow from one side of the layer to the other. Less length of resistive material means less resistance. So fair enough, internal resistance is necessarily reduced.
But my point is that we haven’t seen any data that says how much it is reduced, especially as compared to the entire cell resistance. For a battery as big as the 4680 I guess there could be a few mOhm of conductor resistance, but I would be surprised if it was more than 10% of the dc resistance of the battery—in other words, only enough to offset the thermal issues caused by increasing the diameter. I’m speculating, but I wouldn’t mind seeing some real data.
And my larger point is that this is a manufacturing cost reduction achievement, not a battery technology achievement. How does it result in a battery pack that has 16% longer range? I’m pretty sure they just used the cost savings to make the pack bigger. They would have to make it about 28% larger to offset the reduced energy density with the new battery.
Notice the plot provided by Tesla labels the y axis as “supercharge time increase.” Whatever that means, it’s not real data—it’s marketing hype. Beware of any and all supposed “data” that doesn’t have units and measurement quantities are not all explained. It could be that for the 4650 the difference is 5 s vs 5 hours, or it could be 5 s vs 5 min. Who knows?
Generally agreed, yes. But we do have some numbers mentioned.
Tesla said that the current path shrinks from 250mm to 50mm – so, on average, one fifth the distance. Since all heat generated in the battery is proportional to this, that’s one fifth the heat. Furthermore, since the tabless design has better thermal conductivity throughout the cell, the overall ability to remove heat is better. So you have one fifth the heat, and it’s easier to remove. Since that’s an underlying restriction towards fast charging, I think we can expect a large improvement. But yes, it’s all marketing hype right now. Let’s see what it actually works out to be.
Note that, realistically, we don’t actually need that much faster supercharging. We’re already pretty close or at the sweet spot (180 miles in 15 minutes, IMHO, is the sweet spot). So all we need is a slight improvement and better longevity, which this will undoubtedly provide.
Telek, sorry for the delayed response, but I want to be thorough.
I am not a battery expert, but I don’t think you are correct in saying that all heat generated in the battery is proportional to the Joule heating in the copper foils. For one, the current also flows through the electrolyte, which will have a finite resistance, and there is probably also resistance from the electrolyte to the electrodes, which are lithium and graphite, to the copper anode and aluminum cathode. The chemical reactions that take place also affect the heat, but during charging this is endothermic (cooling) and not significant compared to the heat generated, so I will ignore it.
Typical copper foil used for the anode in an 18650 cell is around 6-10 microns and has approximately 18 layers (a little less than 0.5 mm layer thickness). We can easily calculate the conductor resistance for the copper foil:
– Assuming the foil is only 6 microns thick, using resistivity of copper 1.68e-8 Ohm-m, and assuming height around 60 mm (5 mm at least will go to the terminals etc.), the resistance of the foil per m length is 1.68e-8 Ohm-m / (0.000006 m * 0.060 m) = 46.67 mOhm/m.
– Assuming that the average layer length is half the circumference of the cell, we can estimate the length of the foil. Outer layer length is (18 mm * 3.14) / 2 = 28.26 mm. For 18 layers, that’s 509 mm. Note when you compare this to Tesla’s 250 mm length, they must be referring to the average length of current path, and they must also be comparing to a 2-tab or multi-tab design, because even accounting for inaccuracy in my estimation I think that’s too short for that diameter of battery.
– Putting that together, the total resistance over the length of the foil is 46.67 mOhm/m * 0.509 m = 23.8 mOhm.
– Assume the aluminum cathode foil has approximately the same resistance, and therefore the total conductor resistance for both is around 45-50 mOhm. Remember that’s with 6 micron foil, which for a power-optimized cell could be 10 microns or even thicker.
– Compare that to typical dc internal resistance of an 18650 battery in the range 80-100 mOhm, and also think about the fact that the internal resistance increases with age, which means there are other factors contributing to the internal resistance in addition to the resistance of the conductors.
Now that was for an 18650 cell which of course we have more plentiful information on. Think about this with the 4680 cell size: As you increase the diameter of the cell, the length of the anode gets longer, but the width of the electrolyte layer gets wider. So with a single tab design, you reduce the resistance contributed by the electrolyte etc. by the same proportion as the anode/cathode resistance increases.
In retrospect, my estimation that anode resistance should be <10% of the total internal resistance was low. It could be more like 40-50%. So cutting the anode resistance by 80% could reduce the total resistance by somewhere in the neighborhood of 40%, not insignificant—but not 80%. So I meet you in the middle somewhere.
Info on thermal characteristics during charge/discharge: https://directory.qmed.com/sites/default/files/Electrochem%20Li-ion%20Battery%20Temp%20Trends.pdf
Internal construction info: https://www.electricbike.com/inside-18650-cell/ Also note that in this article, there is a linked IEEE paper which provides some insight on effect of the number of tabs on performance, and suggests that current density improvements gained by adding tabs are more significant for large-format cells vs smaller ones, which was probably also a factor that pushed Tesla to use continuous tabs.
Pretty clever really, effectively charging each layer in parallel and discharging them in series. The voltage doubler of the battery world.
Not sure where you got that idea. It would be more accurate to say they charge & discharge in parallel, but it’s not like there are separate pieces here, it’s just single large foil strips rolled up together.
I think I miss read. Although saying that. It could be interesting to create a battery where parallel charging and series discharging [ and visa versa ] would be possible. No doubt smarter people than me are working on these sorts of things.
There’s no point (or very little point). Remember – it’s mostly about power, and power is equally applied/drawn from each cell regardless of arrangement. If anything, you want more serial because you want higher voltage and less current.
If you take a battery and you have 288 cells in a 96S3P configuration, for example, you get 400V with 96 series of 3 cells in parallel in each cell group. This is what the Chevy Bolt has, and most vehicles to date have standardized on 400V for good reason. Some are going to 800V.
Parallel charging changes nothing. If you could somehow magically switch it to 48S6P (more parallelization) then you get 200V, but at double the current (so now quadruple the losses). This is counterproductive.
Serial charging isn’t much better – sure, you could go 192S2P (if we had 50% more cells – another problem in itself, and more costly), but now you’re 800V – and higher voltage requires more insulation and isolation between transit lines and circuits. This is only used when the amount of power needed is too much for 400V, and mainly only due to charging concerns.
Remember – if you have a power of, let’s say, 72kW in a battery with 288 cells, that’s 250W per cell, regardless of the serial/parallel arrangement.
Friend of mine build an electric car out of Audi V8. This was really the part which hampered the usability of the car. He constructed the battery modules using the 18650 cells in series in order to achieve 48V nominal voltage. This hampered the max charge current as the series resistance grow and caused thermal issues. Now would it be possible to parallel charge the cells within the modules …
Reply to Jussi:
But that’s with a DIY setup, production vehicles are 400V or 800V, so that restriction doesn’t apply.
Ehhhhhh… as a home hacker I won’t be able to buy these so it’s not terribly interesting. For all the advancement in manufacturing of li ion cells I’ve personally seen the price pretty much remain stagnant the last 5 years for the avg. consumer buying 10s-100s of cells. Also BMS chips and charge circuits are still expensive as well. It’s almost as if the whole industry has stagnated.
It’s tempting to just start selling homespun BMS and li ion charge boards for different voltages but then there’s the liability component so maybe not lol.
I’m a little confused at the math on this. They claim 5x energy, 6x the power, and 16% more range. However, the new cells are 5.5x the volume of the old cells. So I don’t think the numbers line up – unless I’m missing something? Shouldn’t at least the range/energy numbers scale in tandem with the size? I understand that the energy density can increase (which is what gives us our 16% more range), but then why is the energy number *lower* than the 5.5x volume difference? I.e. it should be 5.5 * 1.16 = 6.4x energy. Similarly, with a significant reduction in heat buildup (the supercharging chart showed about 1/10th the resistance), shouldn’t the power number be significantly higher?
I immediately assumed the numbers were sucked out the thumbs of the marketing department.
If they could build Li-Ion with a 5x higher energy density than their competitors you’d hear from it via different channels.
I’m also not sure if i’d dare to come near such a battery.
The 4680 cells may have 5x the power of the old cells, but they are also much larger. You can’t fit as many in the same area. It is more efficient because of the reduced packaging materials and interconnects. Together with the advancements in battery chemistry, that gets you 16% more range.
Yes, but I already covered that. The cells are 5.5x the size as the old cells. So there should be AT LEAST 5.5x as much energy, otherwise it’s pointless – and that doesn’t jive with the 16% extra range, as I mentioned.
It seems the batteries have a lower energy density but this is offset by their lower ESR. This would translate to a lower heat production thus higher efficiency as well as a constraint reduction of the cooling system (also compounded by the lower thermal resistance of the new ‘tabs’) which could translate to lighter battery packs or less energy spent on cooling.
All in all I think it’s feasible to have a 16% increase in range; the energy is simply being spent more efficiently.
In order for that to happen, there would have to be far more than a 16% loss in current designs between the battery and the motor, if the density is less but this new system would have a 16% gain in efficiency. There’s closer to maybe a 2% loss in current designs. So that can’t be the reason why. There’s only about a 20% *total* efficiency loss between the wall and the wheels, and half of that is in the charging, and the bulk of the remainder is in the inverter and motor. Lithium ion is typically north of 99% efficient at discharging.
I think the missed part is they do not need as many cells in parallel as with the smaller cells, so same overall volume with less cells in parallel to get more current capability.
The power is different than the energy capacity, so that’s not what is at play here. With identical tech, a cell that’s twice as big can provide twice the amount of power and twice the amount of energy. So there’s something else at play.
fewer cells.
jibe
the cells can discharge faster with the tabless design.
for a given volume, less of the volume is taken up by the shell.
Very few things scale linearly when you do real-world engineering.
While the individual cells have 5x more energy, you can’t fit as many of them in a pack as you can 18650s, because they’re larger. Instead, the efficiency gains of having less individual batteries and lower resistance means that you can get 16% extra range.
As for why the cells are 5.5x bigger but only have 5x the energy, well, there’s likely some packaging considerations, different wall thicknesses, etc… nothing is perfect.
The zero at the end means cylindrical cell
So, what is at the end of a flat pack cell designation?
Tesla cars are still three times more expensive than they need to be to have mass market penetration under a traditional ownership and utilisation regime and no amount of battery tab engineering is going to help with that. The entire battery technology (amongst other things) needs to change to be genuinely competitive with conventional vehicles, even ones running more expensive synthetic fuels so that they are carbon neutral.
Three times? So a new electric vehicle should start at $12k?
They are targeting $25k within 5 years, and the average new car price in the US is $37k… so I think they’re actually well below where they need to be, especially considering that the operational costs are 1/4, and you can save between $1-2k/year of ownership. That isn’t even factoring in the less depreciation. Literally right now, you lose *less* money after 5 years by buying a Model 3 over almost any other vehicle, and that’s before the $10k in price drops that they are targeting.
>”So a new electric vehicle should start at $12k?”
Yes. That’s the entry level price of a new cheap gasoline vehicle, or a second hand car around 8-10 years of age. For example in Europe a Dacia (Renault) Logan starts around $10,400 (~£8,000), or if you want to go slightly more up-scale, you can have a Hyundai Accent starting from $15,925 in the US.
Most people buy sub $20k cars, either new or used. The second hand market accounts for over 70% of all cars sold. The illusion of the “mid-priced” car costing $35k or whatever is caused by only accounting for new cars, and including trucks and SUVs which cost more by default.
So yes, if you want mass-market penetration, you have to aim for $12k. Electric cars are about three times too expensive to have true mass appeal, especially since they have low second hand value because of dying batteries that are exceedingly expensive to replace. They’re going to the scrap-yard instead.
No, most people don’t buy sub $20k cars. Not by far.
Yes, the light car market has some 20-25k models, but that’s also less than a quarter of sales. In Dec 2019, 75% of new car sales were light truck / pickup / SUV. That’s where the average price is $40k+.
No, EVs aren’t 3x as expensive. Multiple reports all clearly show that owning an EV at this point, with today’s prices, have a breakeven point around 5-10 years, and after that is cheaper than an equivalent gas vehicle. By 2025 the up-front cost of an EV and a gas car will be equal for 80%+ of the vehicles sold (meaning breakeven point is 0 days), and at that point even for the cheap vehicles, it’ll still be a 5ish year breakeven point due to significantly less operational and maintenance cost. So spend $20k on a cheap gas car, or spend $25k on a much better EV and recoup that $5k within 5 years, after that you’re saving money.
Also, no, dying batteries are not exceedingly expensive to replace. Today’s batteries (other than the bizarre leaf) have active thermal management, and are expected to last 15-20 years. Even in 10 years, replacement costs will be somewhere around 5-10k *max*, and those batteries will still be useful for grid storage. Even if they aren’t, they are recyclable. They won’t be headed to the scrap-yard.
I understand that even as recent as 5 years ago what you said is true, but things have changed so rapidly, and the rate of change is increasing. We truly are at an inflection point in the industry.
Yes they do. If you want to talk about mass market, you are talking about the 75% of the market that can only afford used OR cheap economy cars with an MRSP well below $20k.
That’s the illusion of the statistics of car prices. New cars account for a minority of the actual market.
>Today’s batteries … expected to last 15-20 years.
Nope. There are some batteries with a -shelf- life up to 20 years (Lithium titanate), but these are not the same low cost high energy density chemistries as used in cars. For NMC cells it’s about 15 years, but companies like Tesla don’t use NMC in cars because of the high cost of cobalt.
The full shelf-life is also only achieved if you don’t actually use the battery (very low cycle count). Higher energy is a compromise with high shelf life and fire safety, and manufacturers optimize for more range at a lower cost rather than longevity (breaks earlier = more sales). The practical life of just about any EV battery on the market is only 10-12 years.
>the light car market has some 20-25k models, but that’s also less than a quarter of sales
That’s cherry picking by selecting a small slice of the distribution. Take cars between 0-25k and that’s closer to half the new cars sold.
People are also keeping their cars longer these days, since they can’t afford to switch. The average age of a vehicle has been climbing up steadily for years. Make do and mend, as they say.
>recoup that $5k within 5 years,
Except you lose at least $5k in the depreciation of the re-sales value, because nobody’s going to pay very much for your used EV with a failing battery.
And the maintenance costs being significantly lower is largely a myth. You’re still changing (battery coolant) fluids and brake parts, shocks, tires at a regular interval, and lose your warranty if you don’t follow the expensive scheduled checks. Plus, the spare parts are mad expensive since there isn’t an established third party parts market.
More precisely, the car market in the US is “bi-modal”. There’s people who buy cheap cars, and there’s people who buy expensive cars, some very expensive which drives up the mean price. In reality very few people actually buy the “average” priced car.
And again, EVs barely touch the second hand market because of the battery lifespan issue. A regular car has a technical lifespan around 20-25 years, at which point they start to come apart and the maintenance is no longer worth it. EVs reach the same point at half the age because a battery costs around a third of the new vehicle price – which is way more than the value of an average car at age 10-12 when the battery is due for replacement. It’s cheaper to scrap the EV and buy another used gasoline car.
I think your numbers are quite off. Most vehicles in not-south don’t have a 20-25 operational lifespan. It’s pretty rare to see a 1990’s vehicle in the North at this point due to the body falling apart, or repair costs being more than the value of the vehicle.
Whereas batteries in today’s cars (don’t look at the leaf) are easily in the 10-20 year lifespan, and due to the massively less complexity in an EV, the rest of the car will last just as long. We fully expect most modern EVs to have up to a 15-20 year lifespan on the original battery, and replacements will be in the 5-10k range after that. The used and refurbished market is already picking up there.
Yes, the market may be bi-modal, but relatively speaking very few people buy brand new cheap cars. Yes, the light car market has some 20-25k models, but that’s also less than a quarter of sales. In Dec 2019, 75% of new car sales were light truck / pickup / SUV. That’s where the average price is $45k+. So those who are actually buying new cars don’t really have to worry about skimping, and if they need to spend $5-10k more in 15 years for a battery replacement, that’s not going to be that big of a deal.
“I think your numbers are quite off. Most vehicles in not-south don’t have a 20-25 operational lifespan. It’s pretty rare to see a 1990’s vehicle in the North at this point due to the body falling apart, or repair costs being more than the value of the vehicle.”
You might want to be less clear-cut here. If “North” here means “New England,” I can buy it. But definitely not middle-US states. It’s 2020. 20 years ago is 2000. There are *plenty* of model-year 2000 and earlier cars still on the road.
“or repair costs being more than the value of the vehicle.”
Why the hell would the repair costs being more than the value of the vehicle matter? The repair costs just have to be less than *buying a new car*.
Plenty being how many, exactly?
According to stats, the average age of a vehicle on the road in the US is 12 years, and ~25% are more than 16 years old. But less than 1% are more than 25 years. So, no, there are not a lot of vehicles older than 20 years old on the road *throughout* the US. I can’t find numbers specific for 20 years, but by the looks of it, probably 5%.
Repair costs being more than the value of the vehicle absolutely matters. Because why spend $5k to repair a vehicle worth $3k when I could just scrap it and buy another used vehicle for $3k?
But, more importantly, people are more likely to start buying a better or newer vehicle when their old one dies.
Having said that, I’m not sure why that’s part of the debate. Those who buy new cars (and that’s all that matters here) are, almost all of the time, spending at least $25k, and 75% of the time the average is $45k+. So this new price point target for a Tesla is at the *bottom end* of what new cars are selling for.
The cost savings over the course of owning the vehicle will be more than double the cost of replacing the battery, so that’s not a concern at all.
> But less than 1% are more than 25 years
Yep. Maintaining a car past 20-25 is rare because you essentially have to rebuild it to keep it. That’s what the statistics show. That’s the technical lifespan of a regular car: that’s when people stop maintaining them and just run them to the ground.
The technical lifespan comes from the same point as the replacement cost: when it costs less to buy another than keep the old one running, the old one gets scrapped for metal value. The average scrapping age of a car is actually quite a bit lower at 14-15 years, but that includes crashed vehicles and other accidents.
>In Dec 2019, 75% of new car sales were light truck / pickup / SUV.
Yeah, but guess what, EVs don’t compete in the truck, pickup, and SUV market because they get such a poor range in actual hauling duty. The Cybertruck is a joke.
Almost all the EVs sold on the market are sedans or hatchbacks. You’re making a false comparison adding $45k+ SUVs in the same lot when the people who would be weighing their choices between an EV and a regular car wouldn’t be buying them anyways.
Secondly, light and medium trucks, pickups, are bought as work vehicles and they see a high turnover because the companies and businesses keep them only for 4-5 years before they toss them onto the second hand market and buy another.
Ok, a few things to unpack here.
First, the original point was that people didn’t have enough money to buy EVs. Since 75%+ of vehicles are $45k+, that’s obviously not the case – and certainly not requiring EVs to be a third of the price that they currently are.
Second, of the 75%+ of vehicles sold that are trucks/SUVs – how many do you think *actually use* the full capabilities of that, beyond which an EV could handle? 5%? 10%? The vast, vast majority of people never or rarely use their big vehicles for towing or hauling. They just like the size and “security” and ability to pack their family with luggage in for a trip, or worse, they just like the look of it.
Thirdly, how often do people haul and need the entire range of their vehicle? We already have EVs today which can tow. As long as you can get around during the day and still make it home above empty (or to your workplace), then you have enough range. This preoccupation with range is bizarre and a relic of an older time.
Lastly you’re not paying attention to the market. By the end of next year, there will be several EV trucks on the market. The F150, Hummer, Cybertruck, and more. These will all have the range and towing capacity needed. And those will be competing in the ICE market segment that is overly inflated in price right now, and will easily be price competitive. By 2025 there will be more than 100 EV models on the road, at price parity, and several manufacturers will be EV only.
Yes, there are a very small number of heavy duty users who won’t be able to be satisfied until maybe 2025, but that’s going to be a tiny portion of the market segment.
If you can bag something like a 2009 Prius with under 120,000 miles for only $4,000 USD, then you’re considerably better off than buying new, and you get to spend a lot less on fuel.
“Three times? So a new electric vehicle should start at $12k?”
Street legal electric golf carts.
rather then having to take
rather then having to continually accelerate
Than is the word. You used the right word here
Rather than having a small battery tab
“These new cells, with their greater energy density and high power output, would appeal to a huge market of hackers and makers.”
I’m pretty sure this is incorrect.
The 4680 doesn’t have a higher energy density; the amount of energy it contains per volume is actually even less than the 21700 cell (it should have otherwise been an 5.4 increase).
It is simply more efficient due to its lower ESR and better thermal performance.
but then how is it 16% more range? I’m thinking that the marketing people didn’t really use exact numbers.
SMD caps have been doing this for decades. Did no one think of building batteries this way until now?!
Actually, there is lithium packs with high C ratings that have done this exact thing for years.
It is really nothing new.
But those have traditionally been flat packs, not round cells, but to be fair, it isn’t even new for round cells either…
There is nothing new going on here…
This method of extending the anode and cathode films alternately beyond the ends of the dielectric film, rolling or folding the films up, then affixing connections to the ends of the cylinder or folded stack is exactly how film capacitors have been manufactured for many years. The technique is called “Schoopage” after the Swiss Engineer Max Schoop who came up with the idea.[1]
Coincidentally, it turns out Big Clive recently released a YouTube video on this topic titled: “Will Tesla’s tabless cells ever use Schoopage?”[2]
1. Film Capacitor – Wikipedia, search for “schoopage” and “Max Schoop”.
https://en.wikipedia.org/wiki/Film_capacitor
2. Will Tesla’s tabless cells ever use Schoopage? By Big Clive.
https://www.youtube.com/watch?v=mYP1sIuOKGY
Tesla (which reminds me of “Edsel”) makes pretty impractical vehicles, so it’s nice to know that they’re contributing something to the world with their battery technology.
Well, Tesla has been in production more years than Edsel.
I’m not sure about the comparative production numbers…
> in the meantime, individuals wanting the best cylindrical cells will have to wait for new Teslas to show up in their local wrecking yards.
Good luck chiseling them out of their epoxy honeycomb!
We’re hackers…
We’ll do what is necessary!
“but nobody at Tesla could figure out why 18650 cells have the trailing zero”
Tesla engineers must be dumb.
The nomenclature is also seen for button cells, like the 1632, which are 16mm by 3.2mm. The height is measured in thenths, which apparently wasn’t needed for the radius.
But think of all the weight they’ll save by dropping the final zero! Just like Jeff the Cyclist in “Pearls Before Swine”: https://www.gocomics.com/pearlsbeforeswine/2012/09/03