Earlier this month, Lawrence Livermore National Laboratory (LLNL) announced to the world that they had achieved a record 1.3 MJ yield from a fusion experiment at their National Ignition Facility (NIF). Yet what does this mean, exactly? As their press release notes, the main advancement of these results will go towards the US’s nuclear weapons arsenal.
This pertains specifically to the US’s nuclear fusion weapons, which LLNL along with Los Alamos National Laboratory (LANL) and other facilities are involved in the research and maintenance of. This traces back to the NIF’s roots in the 1990s, when the stockpile stewardship program was set up as an alternative to nuclear weapons testing. Much of this research involves examining how today’s nuclear weapons degrade over time, and ways to modernize the existing arsenal.
In light of this, one may wonder what the impact of these experimental findings from the NIF are beyond merely ensuring that the principle of MAD remains intact. To answer that question, we have to take a look at inertial confinement fusion (ICF), which is the technology at the core of the NIF’s experiments.
Everything Is Better With Lasers
ICF is one of the two main branches of fusion research, the other being magnetic confinement fusion (MCF) which includes today’s tokamaks and stellarators. Much like with the initial optimism in MCF and the crushing disappointment when Z-pinch fusion turned out to be unworkable, ICF has had its own share of disappointments. Although it was initially regarded to be a practical way to produce power from fusion, it soon turned out that the power requirements to initiate (ignite) fusion were much higher than estimated, and far less easy to achieve.
MCF found a second life in the form of tokamak and stellarator research, which were more complicated, but promised solving the Z-pinch issues, in particular the plasma instabilities. ICF got a fresh start with the invention of powerful lasers, which might be powerful enough to heat fuel and initiate fusion. This process involves subjecting a sphere of fuel uniformly either directly to laser energy (direct drive), or indirectly (indirect drive).
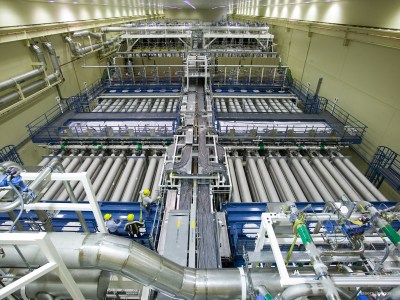
LLNL has been involved in ICF since the 1950s, but only in the 1970s with the advent of more powerful lasers could the first high-power experiments take place. These included the Shiva laser in 1978 and the Nova laser starting in 1984. Both of these laser systems failed to achieve ignition and only got middling results, mostly due to variations in the laser beam’s irradiation.
Despite a funding crunch for fusion research in the 1980s, the findings from these experiments ultimately helped LLNL to establish the NIF, which can be regarded as the successor to the Shiva and Nova laser programs. Through its primary objective of research for the US’s nuclear stewardship program it was ensured of funding, even though construction of the NIF would take significantly longer than originally planned.
Similar to the NIF is the Z Pulsed Power Facility (also known as the Z machine) at the Sandia National Laboratories in New Mexico. This facility uses the Z-pinch principle, making it an MCF system. Despite Z-pinch-based MCF having been discarded as an avenue for power production, it is nevertheless useful for research purposes, making it an important part of the same nuclear stewardship program.
Putting Things Into Perspective
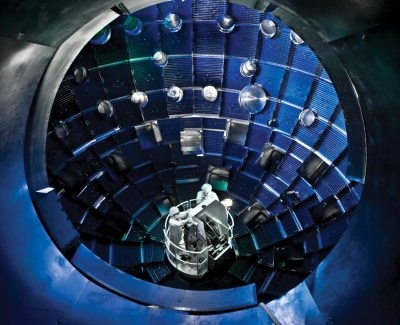
Summarized, the stages in ICF to achieve fusion involve heating up the surface of the fusion target, causing the resulting plasma envelope to expand and thus compressing the fuel. This compression increases the temperature and density of the fuel to the point where it ignites, meaning that the alpha particles produced from fusion are captured inside the fuel and contribute to heating it up.
This in turn causes more fusion events inside the fuel, triggering a chain reaction which ideally fuses all of the fuel, thus releasing all of the potential energy.
In the case of the NIF, a 500 TW (pulse) laser is used, delivering all of the energy to the target within a few picoseconds. Due to the intense stresses on the laser system the NIF is limited to a few hundred firings per year. The fuel is generally not directly irradiated by the laser, but is contained in a Hohlraum, which is a specially shaped hollow object that, when the laser beams enter the cavity (not hitting the fuel pellet) causes it to emit a specific wavelength of radiation. In the case of the NIF this Hohlraum is designed to produce X-rays. This Hohlraum can have two entrance holes, or more, as detailed for e.g. a three-axis hohlraum design by Longyu Kuang et al.
These X-rays heat up the fuel pellet, starting the fusion process. The advantage of using a Hohlraum is that it makes an even heating of the fuel pellet much more straightforward. Even so, the energy requirements to heat up the fuel pellet with ICF are immense. This is detailed in the following diagram from the Wikipedia entry on the NIF:
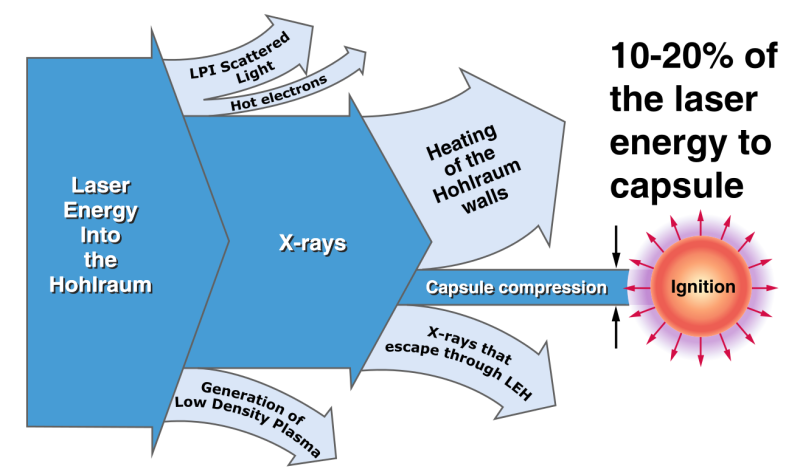
It takes roughly 422 MJ of energy to power the laser system (total system input), of which only a percentage is converted into the laser beam energy that ultimately makes it to the Hohlraum. Of this energy, most contributes to the generating of X-rays within the Hohlraum, only a fraction of which ultimately contributes to the compression of the fuel pellet.
When one considers that it took 422 MJ in order to get a return of approximately 1.3 MJ out of the fuel target, it probably does not require an extensive explanation why this approach to fusion is unlikely to ever reach the point of breakeven, i.e. a Q of 1. The notable aspect here is rather that this amount of released energy was 70% of the input laser energy, meaning that ignition was almost achieved.
No More LIFE
Between 2008 and 2013 LLNL did in fact work on the Laser Inertial Fusion Energy (LIFE) effort which sought to turn the lessons from NIF into a nuclear fusion power plant, using solid-state semiconductor lasers and mass-produced fuel. Yet by the end of the project’s life it was clear that a power plant using ICF was eminently unrealistic, especially in the light of ICF ignition not having been achieved yet.
When one compares the monumental challenges that ICF faces in competing with MCF approaches, with the JET and HL-2M tokamaks as well as the Wendelstein-7X stellarator showing highly promising results in terms of plasma stability and heating, not to mention a Q factor that is tantalizingly close to 1, it should be clear that ICF is not a player in the future power plant game, barring some amazing breakthrough.
What ICF projects like the NIF do however contribute to is increasing our understanding of physics, not just nuclear physics, but also those related to exceedingly powerful laser systems and energy physics in general. That by itself makes this almost-ignition event at the NIF something to cheer at.
[Heading image: Preamplifier at the National Ignition Facility by Lawrence Livermore National Laboratory CC-BY-SA 3.0]
Fusion research seems to be nothing but a works programs\ for turbo nerds. Year after year we here “in five more yearsL
this turns out not to be the case – it has been ‘in TEN more years’ pretty much since I can remember ie the 60’s..
And there’s absolutely nothing wrong with it. Think of all the side effects of this research. Yes, we won’t ever have a practical fusion reactor. But we’ll develop a lot of very useful technologies while toying with it. You know, like pretty much any other fundamental science research.
“Fusion research seems to be nothing but a works programs\ for turbo nerds. ”
Pssst:
“the main advancement of these results will go towards the ** US’s nuclear weapons arsenal. **”
This actually isn’t fusion *power* research. It’s fusion *ignition* research, and, uh, we’ve been achieving fusion ignition for a *long time* now. Just… not in the way you’re thinking of.
For comparison, the energy for each shot is equivalent to the fully charged energy in a 117 kWh electric car battery, which is just a little larger than the 80-100 kWh that Teslas typically have—except of course that it’s all used up in picoseconds, not hours. The cost of each shot is just 15$ , though :)
I’m guessing the cost of those fuel pellets is a little more than 15$ !
You are correct. However in the LIFE and other IFE programs the mass production of targets has been studied. I don’t remember the costs, but given the development of a mass production process cost would go way down.
It cost at least a million dollars; took 6 months to make, out of diamond and gold. To shoot off 10 a second, each target would have to cost about a quarter.
It is good to persevere despite setbacks. The rewards of the nif achievrment could well mean a better and safer and climate stable earth. WELDONE NIF
I don’t understand where things would go even if they even do manage to break even on liberated energy from fusion, someone swapping a pellet every round just to eek a few joules out? Or is this only for research
Maybe they could feed it in, along with a hohlrahm, ala welding rod. Where as it just starts to fuse, and they slowly slide in next length of hohlram and fuel pellet in a continuous feed system.as each begins to fuse etc.
A lot of the people working on fusion think that for inertial confinement to be practical for power generation they’ll have to do away with the hohlraum and directly illuminate the target. NIF is really just a stepping stone to that.
An indirect drive could be a power generating stepping stone in itself though. The article notes that about 400MJ went into the current 1.3MJ record. That 400MJ is from a facility built with no eye on reducing wall power consumption and 15 year old laser tech. Just swapping the laser pump from flash lamps to diodes could knock that down by 10x (400MJ to 40MJ), it’s like swapping an incandescent bulb for a an LED. The real question is whether the exponential gains in yield seen with this last record will continue enough to get from ~1MJ to 10’s or even low 100’s of MJ. Seeing as ignition is an exponential regime where fusion helps to beget more fusion, it’s not implausible (though still yet to be proven).
Electricity was just a laboratory curiosity with no practical applications at one point, too. What’s “only for research” today can have practical applications tomorrow.
NIF is primarily for research, but there are proposed designs for ways for commercializing inertial confinement fusion. Mostly via automating the injection of pellets. This is a *big* step towards that, though ICF is still kind of considered the dark horse for practical fusion power with the ITER design being more favored overall.
Feeding for real life usage is only half the story. How do you capture the output *efficiently* of high energy within a short duration? And most importantly what is the type of energy you are receiving and how to transform it into a usable form efficiently?
Casually I look at the pictures under fusion, I only see sub-atomic particles and gamma radiations as output. This following is from the wiki page that the picture of article is based on.
>In 2018 a significant improvement in output was announced, the result of continuing improvements in controlling compression asymmetry. A shot with an output of 1.9×1016 neutrons resulting in 0.054 MJ of fusion energy released by 1.5 MJ laser pulse
Um. This, uh, isn’t about fusion power.
Think about it. They’ve got a small core of fusible material. They’re delivering energy to it such that it collapses and begins to fuse and hoping to reach a temperature where the fusion reaction becomes runaway and burns the whole thing.
This process should sound a *whole lot* like another one – just initiated via a different mechanism, and, with, uh, much more destructive results.
Well, it may be more destructive, but igniting it without a nuclear fision weapon, would allow the “war winners” to use the “conquered” terrains without waiting some thousand years for the radiocative contamination…
So to be clear, studying ignition and laser-induced fusion/fission isn’t about a new way to make a thermonuclear device: it’s about testing how existing ones age and checking any replacement components without actually, y’know, blowing stuff up. Because of that whole “it’d be a treaty violation now” thing.
As the article pointed out, being able to do ignition studies is basically a side effect of having a big giant laser used for actual weapons studies. It’d be nuts to actually *build* a laser like that for this purpose, but, hey, since we’ve *got* it…
Meanwhile, there are millions, if not billions of tons of non weapons grade radioactive material that could be used to power nuclear plants and provide, literally, free energy to every one.
You know that most energy is in fact “free”?
You don’t really pay petrol, it’s in the ground, just need to extract it, you don’t pay a petrol god with virgins or toddlers.
You on the other hand pay whoever have power on the land and on the extraction chain.
So it will be the same with your non weapon grade radioactive material.
Or you can install some solar panel on your roof and have free energy from the sun god.
That’s fair. So let me elaborate on that.
That is not what I mean. What I mean it is that is is so readily available that and there is so much of it that other than the cost of building the plants and operating it, and it’s efficiency, whatever the cost per KW, it would be so cheap, that it would be free compared to petrol, the damage it does the environment, etc. And it would be so cheap that we could probably do give it away for free to places so poor that they still live without it today.
Doesn’t fusion actually cause global warming by directly warming the planet with energy previously locked up? As does a conventional nuclear power plant? That energy then wanders off, unlike CO2 that hangs around, of course.
“Meanwhile, there are millions, if not billions of tons of non weapons grade radioactive material ”
Meanwhile, this process is *really* about studying those small bits of remaining *weapons* grade stuff. It’s ignition research, not power research.
1.3 MJ – that’s about 1-seconds worth of fairly mediocre racing car engine.
Assuming 35% efficiency of combustion, that’s 610 HP. Not too shabby. Beat’s my 186 HP.
So, to power the 24 Heures du Mans, that would take 86,400 NIF reactors strapped to the car. As at present, pretty sure it takes more than a day to reset and go. Probably easier to move the racetrack around the car.
Nonsense aside, this is still quite a milestone for NIF and an interesting stepping stone on the way to fusion power. The future’s vast, we’re bound to get there at some point.
People are genuinely upset that a 2 millimetre pellet does have “””only””” the same energy as 37 millilitres of gasoline?
I have seen everything on the internet now.
“I have seen everything on the internet now.”
How much time would that take and then how long in therapy?
Or 31 times the energy density of sunlight per M2
Uh no, thats actually 31 times Langley.
I started taking off my solar panels, free energy is close.
NIF never meant much, it was always to get data for the right thermodynamic equation of state relevant to trigger a H-bomb. (necessary to validate computer models).
Most likely kind of ‘ICF’, even though it also kind of isn’t, it’s really ‘electrostatic confinement fusion’, is Bussard’s Polywell.
You should just watch https://www.youtube.com/watch?v=FhL5VO2NStU and decide for yourself.
That video has been around since 2006, and it seems the ‘right’ kind of people haven’t followed up on it yet.
Latest paper about it misused the beta equation – On one page, they stated in words correctly that beta was the pressure balance between the injected electrons and the magnetic field, yet, on the same page, they have an equation that uses the mass of the ions. Which is only relevant to tokamaks.
Even though the magnetic fields of the polywell are only there to make the electrons avoid the grid – nothing else, really, except that it turns out if you drive it not too hard, and not too soft, there’s a nice ‘wiffle ball’ effect that makes it far better at trapping electrons, which turns out to do the trick.
And obviously, getting the field strength calculation wrong is like mistaking a ping pong ball with a golf ball.
Imagine taking a golf club to a ping-pong match, that’s what getting that wrong is like.
But in that case, the ping pong ball is only 17 times lighter than you expect. Comparing just one proton to an electron, and that ratio is 1836.15267343. Get it wrong, and guess what – the magnetic field *doesn’t* help trap electrons, just as Bussard shows in that video.
It also should be clear that for this thing to work, the background pressure has to be in the ultra-high vaccum range (at least to start, before any fuel is allowed in). Not the ‘plasma pressure’ range that tokamaks use. Finally, the ‘triple product’ doesn’t really matter at all – this thing is a high flow, isotrophic auto-recycling (of failed collision and misses) particle accelerator. Voltage depth of the well == available energy, so temperature doesn’t matter as much (although it does affect loss-balance).
Bussard’s point was that he considered the science ‘done’. All that’s left is the ‘engineering problem’ of building one. And he points out, he has empirical data at different scales to show how it *really* scales, whereas tokamak’s don’t even have that.
It’d need to be a few m across, 99.999% of collisions do this) it doesn’t matter, because they ‘recycle’ their energy back into the electrodes as they head out again. Only to also fail to quite reach anywhere, causing them to repeat the trip back and forth, 100,000 or even millions of times, before they finally sucessfully fuse, retaining total charge, but getting enough extra energy to finally escape outwards.
The main reason ‘power feedback’ (aka ‘ignition’ aka ‘chain reaction’) doesn’t happen internal to the Polywell, is that the fusion-generated particles are so energetic, they are penetrating radiation in their own right as well. They’d penetrate solid matter, why would you think they’d penetrate a relatively massless plasma and yet get absorbed enough to dump much of their heat there? Doesn’t happen. Fusion is one and done – unless the plasma is, oh, I don’t know, the size of a small star?
What works in a H-bomb is that the speed of light itself keeps the heat from escaping. And in those, it’s just to get hard neutrons – 80% of the yield is from U238 shattered by those (it’s not fissile to ‘cold’ neutrons, but if you hit it *hard* enough…).
If a ball of hydrogen in space isn’t large enough to allow for fusion chain reaction, then it just stays a ball of gas. No reason all that ‘missing dark matter’ can’t be just said boring cold gas planets hurtling through the black. Why would you expect to see them through a telescope? I mean, they might cause light to diffract, but apart from that…
Anyway, someone (Tod H Rider) did a calculation of just how much X-ray loss power you should expect from any plasma hot and dense enough to ‘achieve fusion ignition’. And realised it would only be sustainable for balls with a sufficiently tiny surface-area to volume ratio… like, say, a star. Strange that. He promptly left the fusion field altogether.
This may also be why NIF and JET both can only claim ‘more power came out than we put in!”, for certain measures of ‘in’, and, if you include all the useless X-rays in what came ‘out’.
Fortunately the polywell does empirically show performance and size-scaling which indicates hope. This is again mostly because the colliding particles are not so thermally distributed, if you do things right (keep the voltage up, pressure down, electron excess population high enough and the magnetic field in balance against said population, etc).
Totally will thermalise, and quickly, if you let the pressure get too high because you’re insisting on it acting like a tokamak, though.
Good luck with that.
Also, doesn’t work well if you misdesign it such that the injected electrons can pass through the mag-grid, then make it to the outer walls before stopping, because you forget about the ‘free’ velocity you get from a hot cathode (only a small voltage of ‘self biasing’, but still good for velocities comparable to earth escape velocity…). You need those to stop, turn around, and get ‘dragged back in’, because they cost you energy to get free in the first place.
You only want them deliberately impacting specific terminals to cause secondary emission, terminals which are deliberately closer than the walls, and connected to the high voltage power supply. (Look for such stupidity as putting the initial electron guns *outside* the chamber, where *every single* electron is *guarenteed* to be able to reach the wall again after just one piddly pass at the mag-grid).
What’s super nice about the polywell, with it’s ‘adjustable voltage well depth’, is that it may fuse more difficult aneutronic fuels like p-B11, and that gets you mostly alpha particles, which are individually heavy (compared to neutrons at least), and carry +2 charge each. (and each have a few MeV of energy on them too). You need about 100kV of ‘well depth’ for that to begin to work, because fully-ionised boron has a charge of +5, so when it gets to the centre, it will have picked up ~500keV energy — just what it needs to maximise it’s odds of fusion, even though they are still spectacularly poor.
So you can ‘harness’ most of that energy almost directly by wrapping the polywell in yet another set of electrodes, these set up to decelerate and collect that energy electrically. Something you can’t do if most of the energy comes out in ultra-fast neutrons like what you have to accept from D-T and D-D fusion. (which are frankly horrible to deal with – like, standing behind a lead shield *gets you killed faster* because they like to generate very high energy X-rays when they crash into high-Z nuclei).
It’s an exciting technology, mostly because with it you could get a single-stage aerospacecraft to orbit about 1000x cheaper than it takes with chemical fuel. And an interplanetary craft could get to mars in just a few weeks.
But it’s probably going to be like jet engines in terms of maintainance profiles. Think, needs regular TLC.
Probably things like LFTR’s can do energy production much more cheaply, and with far far less complexity, and nearly no development by comparison. But they are heavier.
And, unavoidably, fission means messy horrible neutrons making everything too close radioactive whereever they fall.
Funny how you never hear the tokamak guys talking about that little problem.
“No reason all that ‘missing dark matter’ can’t be just said boring cold gas planets hurtling through the black. Why would you expect to see them through a telescope?”
Nope. Very, very nope.
Back in the late 90s/early 2000s there were plenty of studies to try to figure out if the missing matter could be sub-stellar bodies like you’re suggesting. It’s not that hard to do lensing studies to figure out if it’s possible – the *number* of objects you need is so high that the statistics is easy. By the late 2000s (through the MACHO/EROS collaborations) it was very clear that the mass needed for the Galactic halo *cannot* be made up of substellar objects. Nowhere near enough.
But the *real* death knell came from studies of the cosmic microwave background (CMB) anisotropy. The CMB anisotropy studies tell you about how the Universe was interacting right at the point where protons and electrons combined into hydrogen, and the Universe became transparent.
No stars. No boring cold gas planets. None of that. Just primordial plasma, at totally tractable temperatures, around 3000K. Stuff we can measure in labs easy. And those measurements said that not only is the fraction of matter-like “stuff” (positive pressure material which clumps under gravity) still 30% (just like the dark matter estimates said) – the *vast majority* of that “stuff” does *not* couple to radiation.
In other words – the vast majority of the “matter like stuff” (clumps under gravity) does *not* produce bright hot spots of radiation when compressed. The ‘dark’ in ‘dark matter’ no longer means “doesn’t produce light.” It now means “doesn’t couple to radiation.” Big difference.
That’s a total deathblow for “boring cold gas planets” being dark matter. Just not possible. Has to be something other than normal baryonic matter. *Could* still be compact objects like black holes of just the right size, but definitely can’t be “boring cold gas planets.”
“And, unavoidably, fission means messy horrible neutrons making everything too close radioactive whereever they fall.
Funny how you never hear the tokamak guys talking about that little problem.”
If… you actually talk to one… you’ll find it’s one of the things they discuss most. It’s super important, but also workable.
UKAEA recently built a new shiny materials research facility to look into this kind of thing. It’s a huge part of any reactor design. Choosing materials that avoid getting activated for long periods, while staying structurally sound for long enough and putting up with thermal loads.
Neutrons aren’t horrible. Cakes are nice and they’re full of neutrons.
“That video has been around since 2006, and it seems the ‘right’ kind of people haven’t followed up on it yet.”
Research into polywell fusion devices were funded by the US Navy through the latter portion of the 2010s, but funding dried up after the last device failed to produce any fusion (signalling something was dramatically wrong with the simulations).
But, I mean, there are NIAC funded proposals still studying IEC designs, so I have no idea what you mean implying that it hasn’t been followed up on. And Sydney still has a functioning low-beta prototype, so the idea that there’s easy magic fusion that’s just not being looked into seems really weird. Sadly, there were several problems with Bussard’s understanding such that his “the science is complete” idea was unfortunately naive, as evidenced by the fact that *multiple* devices (WB8, MCVC-0) that ‘should have’ worked both failed completely in one way or another.
…When one considers that it took 422 MJ in order to get a return of approximately 1.3 MJ out of the fuel target, it probably does not require an extensive explanation why this approach to fusion is unlikely to ever reach the point of breakeven…”
…and an even less extensive explanation when one considers that the energy contained in one gallon of gasoline is approximately 130 MJ.
How about a Hackaday article on something we can all try in our sheds – Cold Fusion?