We here on Earth live at the bottom of an ocean of nitrogen. Nearly 80% of every breath we take is nitrogen, and the element is a vital component of the building blocks of life. Nitrogen is critical to the backbone of proteins that form the scaffold that life hangs on and that catalyze the myriad reactions in our cells, and the information needed to build these biopolymers is encoded in nucleic acids, themselves nitrogen-rich molecules.
And yet, in its abundant gaseous form, nitrogen remains directly unavailable to higher life forms, unusably inert and unreactive. We must steal our vital supply of nitrogen from the few species that have learned the biochemical trick of turning atmospheric nitrogen into more reactive compounds like ammonia. Or at least until relatively recently, when a couple of particularly clever members of our species found a way to pull nitrogen from the air using a combination of chemistry and engineering now known as the Haber-Bosch process.
Haber-Bosch has been wildly successful, and thanks to the crops fertilized with its nitrogenous output, is directly responsible for growing the population from a billion people in 1900 to almost eight billion people today. Fully 50% of the nitrogen in your body right now probably came from a Haber-Bosch reactor somewhere, so we all quite literally depend on it for our lives. As miraculous as Haber-Bosch is, though, it’s not without its problems, particularly in this age of dwindling supplies of the fossil fuels needed to run it. Here, we’ll take a deep dive into Haber-Bosch, and we’ll also take a look at ways to potentially decarbonize our nitrogen fixation industry in the future.
Easy to Find, Hard to Use
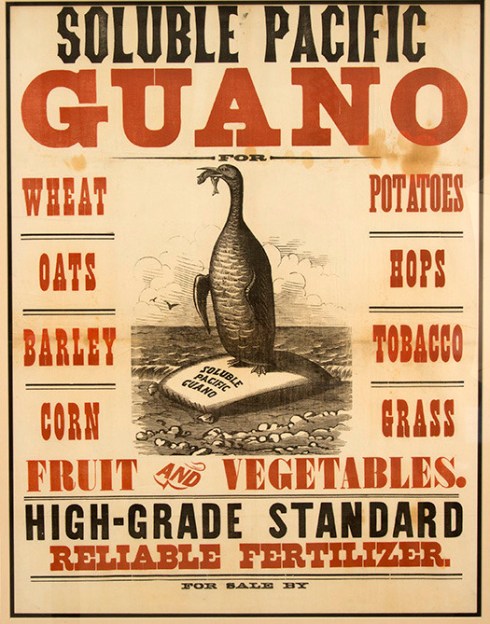
The heart of the nitrogen problem, and the reason why the production of ammonia is both necessary and so energy-intensive, stems from the nature of the element itself, specifically its tendency to bond strongly with others of its kind. Nitrogen has three unpaired electrons available for bonding, and the triple bond that results in the diatomic nitrogen that makes up most of our atmosphere is very difficult to break.
These triple bonds are what make gaseous nitrogen so inert, but it also creates a problem for the organisms that need elemental nitrogen to survive. Nature has found a number of hacks to that problem, through nitrogen-fixing processes, which use enzymes as catalysts to convert diatomic nitrogen into ammonia or other nitrogenous compounds.
Nitrogen-fixing microorganisms make nitrogen bioavailable up and down the food chain, and for most of human history, natural processes were the only method of obtaining the nitrogen necessary for the fertilization of crops. Mining of deposits of nitrogenous compounds, such as saltpeter (potassium nitrate) or in the form of guano from bat and bird droppings, was once the primary source of nitrates for agriculture and industry.
But such deposits are relatively rare and finite in extent, leading to a problem both in terms of feeding a rapidly expanding world population and providing them with the products needed for an increased standard of living. This led chemists to search for methods of turning the vast reserves of atmospheric nitrogen into usable ammonia, starting at the end of the 19th century. While there were several successful contenders, German chemist Fritz Haber’s laboratory demonstration of making ammonia from air became the de facto process; once it was scaled up and industrialized by chemist and engineer Carl Bosch, the Haber-Bosch process was born.
Under Pressure
The simple chemistry of the Haber-Bosch process belies its complexity, especially when undertaken at industrial scales. The overall reaction makes it seem quite straightforward — a little nitrogen, a little hydrogen, and you’ve got ammonia:
But the problem lies in the aforementioned triple bond in the N2 molecule, as well as in that double-headed arrow in the equation. That means the reaction can go both ways, and depending on reaction conditions such as pressure and temperature, it’s actually more likely to run in reverse, with ammonia decomposing back into nitrogen and hydrogen. Driving the reaction toward the production of ammonia is the trick, as is providing the energy needed to break down diatomic nitrogen in the atmosphere. The other trick is providing enough hydrogen, an element that is not particularly abundant in our atmosphere.
To achieve all of these goals, the Haber-Bosch process relies on heat and pressure — a lot of each. The process starts with the production of hydrogen by steam reformation of natural gas, or methane:
Steam reformation takes place as a continuous process, where natural gas and superheated steam are pumped into a reaction chamber containing nickel catalyst. The output of the first reformer process is further reacted to remove the carbon monoxide and unreacted methane and scrubbed of any sulfur-containing compounds and carbon dioxide, until nothing remains but nitrogen and hydrogen.
The two feed gasses are then pumped into a heavy-walled reaction chamber in a ratio of three hydrogen molecules to each nitrogen molecule. The reactor vessel must be extremely sturdy because the optimum conditions to drive the reaction to completion are a temperature of 450 °C and a pressure 300 times atmospheric. The key to the reaction is the catalyst inside the reactor, most of which are based on powdered iron. The catalyst allows the nitrogen and hydrogen to bind into ammonia, which is removed by condensing it into a liquid state.
The handy thing about Haber-Bosch is what Bosch brought to the table: scalability. Ammonia plants can be massive, and are often co-located with other chemical plants that use ammonia as feedstock for their processes. About 80% of the ammonia produced by the Haber-Bosch process is destined for agricultural uses, either applied directly to the soil as a liquid, or in the manufacture of pelletized fertilizer. Ammonia is also an ingredient in hundreds of other products, from explosives to textiles to dyes, to the tune of over 230 million tonnes produced worldwide in 2018.
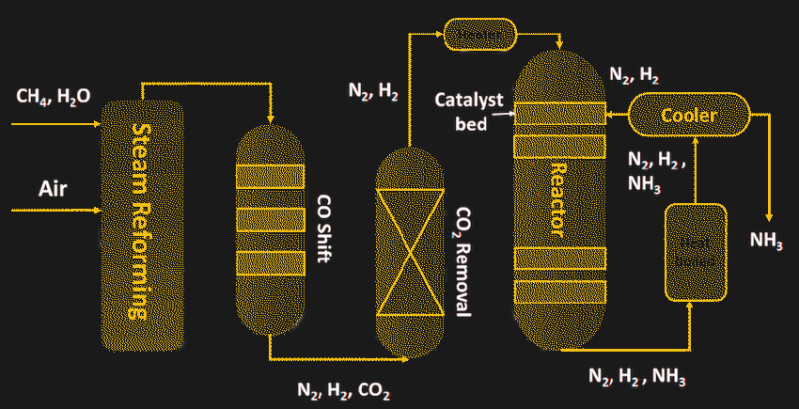
Cleaner and Greener?
Between the use of methane as both feedstock and fuel, Haber-Bosch is a very dirty process from an environmental standpoint. Worldwide, Haber-Bosch consumes almost 5% of natural gas production, and is responsible for about 2% of the total world energy supply. Then there’s the CO2 the process produces; while a lot of it is captured and sold off as a useful byproduct, ammonia production produced something like 450 million tonnes of CO2 in 2010, or about 1% of the total global emissions. Add in the fact that something like 50% of the food production is absolutely dependent on ammonia, and you’ve got a ripe target for decarbonization.
One way to knock Haber-Bosch off the ammonia pedestal is to leverage electrolytic processes. In the simplest case, electrolysis could be used to create the hydrogen feedstock from water rather than methane. While natural gas would still likely be needed to generate the pressures and temperatures needed for ammonia synthesis, this would at least eliminate methane as a feedstock. And if the electrolytic cells could be powered by renewable sources like wind or solar, such a hybrid approach could go a long way to cleaning up Haber-Bosch.
But some researchers are looking at a completely electrolytic process that will make ammonia production much greener than even the hybrid approach. In a recent paper, a team from Monash University in Australia details an electrolytic process that uses chemistry similar to that in lithium batteries to make ammonia in a completely different way, one that potentially eliminates most of the dirtier aspects of Haber-Bosch.
The process uses a lithium-containing electrolyte in a small electrochemical cell; when current is applied to the cell, atmospheric nitrogen dissolved in the electrolyte combines with lithium to make lithium nitride (Li3N) at the cathode of the cell. Lithium nitride looks a lot like ammonia, with the three lithium atoms standing in for the three hydrogens, and sort of acts like a scaffolding upon which to build ammonia. All that remains is to replace the lithium atoms with hydrogen — a feat easier said than done.
The secret to the process lies in a class of chemicals called phosphonium, which are positively charged molecules with phosphorus at the center. The phosphonium salt used by the Monash team proved to be effective at carrying protons from the anode of the cell to the lithium nitride, which readily accepted the donation. But they also found that the phosphonium molecule could go through the process again, picking up a proton at the anodes and delivering it to the lithium nitride at the cathode. In this way, all three lithium atoms in the lithium nitride are replaced with hydrogen, resulting in ammonia produced at room temperature without methane as a feedstock. The Monash process appears promising. In a 20-hour test under lab conditions, a small cell produced 53 nanomoles of ammonia per second for every square centimeter of electrode surface, and did it with an electrical efficiency of 69%.
If the method can prove out, it has a lot of advantages over Haber-Bosch. Chief among these is the lack of high temperatures and pressures, and the fact that the whole thing could potentially run on nothing but renewable electricity. There’s also the possibility that this could be the key to smaller, distributed ammonia production; rather than relying on a relatively few centralized industrial plants, ammonia production could potentially be miniaturized and brought closer to the point of use.
There are plenty of hurdles to overcome with the Monash process, of course. Relying on lithium electrolytes in a world where EVs and other battery-powered devices are already stretching the limits of lithium extraction seems tenuous, and the fact that lithium mining is heavily dependent on fossil fuels, at least for the time being, tarnishes the green potential of electrolytic ammonia as well. Still, it’s an exciting development and one that just may keep the world fed and fueled in a cleaner, greener way.
https://www.nature.com/articles/s41565-020-00809-9?proof=t%29.
Mechanochemistry for ammonia synthesis under mild conditions
isn’t this the best ammonia synthesis alternative ?
Haber/Bosch is energy intensive, something like half a million joules per mole of product, and requires enormous factories to achieve efficiency of scale. People have been looking into replacing it for at least the past 50 years, to no effect.
(I have a project over on .IO that makes one attempt at a replacement, and have been looking into a 2nd attempt for the past 5 years or so. It’s a really hard problem.)
I skimmed the paper you cited, and:
“The energy consumption needed to produce one tonne of ammonia is 4.5×1012 J, which is competitive in comparison with the Haber–Bosch process at the laboratory scale (Supplementary Table 1)34. However, it is substantially higher than the state-of-the-art Haber–Bosch process at the industrial scale.”
The “substantially higher” means that it is even *more* energy intensive than HB, so it’s unlikely to be a replacement without further development.
That being said, higher energy requirement isn’t by itself a problem for a replacement. As noted, much of the cost of Ammonia is due to the cost of the factory and the shipping. Hypothetically, a small production facility, in a farming town say, might be useful.
Also, there’s lots of “stranded energy” sources around the country: wind and solar energy that goes to waste because there’s no consumers at the time of generation. If you have a system that can be quickly spun up to take the extra energy and use it to produce something useful, such as Ammonia, then that energy wouldn’t go to waste. It sounds like the mechanical process might satisfy those conditions.
The last point is the kicker – as renewables grow we will see more periods when there’s effectively a surplus of energy with no-one to buy it, processes that are energy intensive but can ramp up quickly can take full advantage of this… hell, even slower processes that can run overnight when the wind is blowing are still a pretty good bet.
Agreed. I am hoping the day will come where a home with solar or wind can be truly off-grid. To do so means having not just daily storage (aka a battery), but seasonal storage where surplus energy when the battery is full is stored for use months later when sunshine is less prevalent.
Ammonia has some distinct advantages for this in that aqueous ammonia is fairly energy-dense, easily stored in tanks, and potentially usable in either fuel cells or an ICE. NH3 is also carbon-neutral in that it has no C in its molecular structure that would be released on use. All it lacks is a small-scale earth-friendly production method.
With both daily and seasonal energy reserves, a home could finally be independent from the local electric grid. I see NH3 as the best path to this vision. If the summer months did not produce enough NH3 to last the winter, one could have more trucked in for emergency coverage. If winter ends and there is still some left, it could fertilize the spring planting or be sold for profit.
“While natural gas would still likely be needed to generate the pressures and temperatures needed for ammonia synthesis”
No.
Additionally, the ammonia forming reaction is exothermic. At a lower temperature the reaction yield is higher but it happens more slowly and the process produces heat.
Haber was instrumental in convincing Germany to use chemical warfare in the first world war and he drove his wife to suicide.
> Haber was instrumental in convincing Germany to use chemical warfare in the first world war and he
> drove his wife to suicide.
The French were the first to use gas in warfare, under the direction of Lavoisier. Haber was the first to use *poisonous* gas, and gets all of the blame despite the fact that the French did it first. Winners write the history.
Despite the poison gas thing, he also invented his ammonia process was one of two breakthroughs which allowed us to quadruple agricultural production worldwide. (The other one being pesticides.)
He received the Nobel prize for an invention that continues to feed most of the world.
It was also a different time, and he lived in a different culture.
We can admire his accomplishments while simultaneously disagreeing with his politics.
The difference between the use of non-lethal tear gas and poison gas is extremely noteworthy. It’s not particularly an example of the winners writing the story. Especially as it was already prohibited by treaty, the treaty that Germany then broke.
The first world war wasn’t really about politics (the opposing sides didn’t disagree about anything in particular beyond not letting the other side ‘win’).
Given elemental nitrogen and hydrogen, perhaps. But you can ignore the vastly greater endothermic demands of cracking N2 into elemental nitrogen.
If the Monash process pans out, it would be revolutionary. The high pressures and temperatures required by Haber-Bosch are difficult to achieve with electricity, which is the stereotypical green energy source. H-B uses natgas, not only as a feedstock for hydrogen, but as a simple and effective heat source for driving the process.
Doing H-B from electricity would require expensive and maintenance-prone multi-stage heating and compression machinery.
Without Monash, we will be limited to a billion or so people, after the natgas runs out.
What about using nuclear reactors to get heat and pressure?
Sure.. let’s start messing with HO explosives and the nitrogen bond inside the confines of a nuclear reactor. What could go wrong?
Snark aside, I don’t think that’s wise. Especially if a workable method is evolving that does not require such high temperatures and pressures.
Dude that is just an ignorant comment
Not correct. In synthesis, there is no such thing as elemental nitrogen or hydrogen. Interstellar space is another story.
The reaction as described above (3 H2 + N2 -> 2 NH3) is exothermic at room temperature. At high temperature the negative forward entropy pushes the reaction backwards. Why do we heat then? To get useful reaction kinetics. Why do we pressure it? To compensate for the heating.
This has always been the best first year chemistry illustration of enthalpy, entropy, temperature, pressure and energy of activation and their interactions.
“All” you need a better catalyst that would allow to run the reaction fast at room temperature. As my prof concluded back then: “If you ever want to be a billionaire chemist, this is the only discovery you ever need to make.” Yes, the problem IS a hard one.
Pressure is cheap, heat is expensive. Monash requires (at least formally) metallic Li, which means electricity, which is comparatively very expensive to produce. We’re not there yet, but any progress opens up new options.
My cat has figured out how to make ammonia, and it uses no electricity or fossil fuels at all…
Side bar: your cat makes urea, which bacteria break down into ammonia. Your cat makes urea because we need a good way to get excess nitrogen out of the body, as its byproducts are toxic (particularly ammonia.) The only source we have for getting nitrogen is the proteins we eat.
Birds deal with this a little differently by making solid uric acid, which doesn’t require vast quantities of water (pee) to keep the nitrogen-containing crap dissolved. If you don’t keep it sufficiently dissolved it begins to crystallize out in places you don’t want it, causing gout. One way urea-based gout is treated is by reducing the amount of protein people eat.
But fundamentally, animals can’t make ammonia. Our bodies do surprisingly little nitrogen-based chemistry given that all proteins, which make up almost everything in our bodies, are themselves made of amino acids that rely on nitrogen bonds to link adjacent amino acids together. We do some nitrogen chemistry, but don’t generally change the oxidation state of the nitrogen. That’s where the H-B process and bacterial nitrogen fixation shine: they’re ways of reducing the energy of activation of those triple bonds down to the point where reactions start happening.
I think it’s kinda cool that nitrogen is pretty happy in both N2 and NH3 forms, where it’s accepting 3 electrons from other atoms, and in HNO3 forms, where it’s donating 5 electrons to other atoms. In a way it’s behaving like a semiconductor. But most of the cool chemistry happens with the atoms that are ambivalent about whether they donate or accept electrons and will go either way if they’re bored.
Second side bar: “as well as in that double-headed arrow in the equation.”
All chemical reactions have that double headed arrow: they’re all reversible. The reaction dynamics are defined by the relative energies in the two different states on either side of the arrow, and most of chemistry is figuring out how to make a set of reaction conditions that favors the product you want. Some reactions are really low-energy: H2O busting into HO- and H+ is spontaneous and at any given time about one in a million water molecules is disassociated into an acid and a base. Some reactions have a huge differential between reactants and products, like explosives, where the balance might be one molecule of explosive per solar system of molecules in the disassociated state. But it’s always bidirectional.
Haber-Bosch made us entirely dependant on a finite resource, and gave us the nightmare population collapse scenario which we’re now ALL facing. I hope you survive it.
> Haber-Bosch made us entirely dependant on a finite resource, and gave us the nightmare population
> collapse scenario which we’re now ALL facing. I hope you survive it.
Your statement is completely backward, in just about every way.
Firstly, terrestrial nitrogen sources (sources of bat guano and bird droppings) were the finite resource, and at the time we were using them up at an alarming rate.
Secondly, the nightmare population collapse would have happened if Haber had *not* invented his process – he’s credited with having averting that problem, which was calculated to have happened around 1950.
And finally, world population is not in any way a problem. For the last 20 years UN estimates held that world population would peak at around 2100 (at 9 billion) and then start going down, but these have been recently updated. The current estimate is that world population will peak at around 2050 and then start to decrease.
It appears that once poverty and a good standard of living are achieved, the population of a country starts going down. In fact, under-population is starting to become a problem for some countries such as Japan.
The US fertility rate is below 2, which means that our population would be going down except for immigration.
Population *used* to be a concern, but is no longer considered to be a problem we need to solve.
Happy Days!
Everybody please relax! Thorium is the new wonder material, that will save us from all our woes. Bill is building a nice new TerraPower reactor, so that pesky environment won’t be giving us any more grief. Everything is just fine!
Thank you for adding a bit of sense to all the hysteria.
“It appears that once poverty and a good standard of living are achieved”
You can do your part right away by moving to San Fierro!
Sorry – I think this reply is backward in just about every way.
The problem is people think what we have now is optimal and if we just maintain this status quo everything is OK.
Even one billion people in 1900 was unsustainable. At 6 billion we have global warming, depletion of fresh water, lithium, copper shortages, species extinction, loss of river habitat etc etc. Most birds are now chickens to feed us! More people live in high rise cities, as they should because this puts less pressure on the environment, but is this really what we are striving for? – living like battery hens ourselves.
This is at 6 billion. At 9 billion it will be even worse. The status quo is not OK. The root cause of nearly all the crises we face is CURRENT overpopulation. Thus any population decline is fantastic (opinions of a certain religious leader not withstanding) . We don’t need to worry about under-population, at least for next few hundred years. Sadly it will take some time to reduce our population by several billion.
So …. are you going to do your bit to solve overpopulation? I expect not as it’s always other people that have to change. Your comment “… sadly it will take some time to reduce our population by several billion” is one of the most unkind and inhuman comments I have ever read on this site. I’m not in the habit of making ad hominem attacks on contributors but I find it difficult to phrase my disgust in any other way. These are people you wish to “reduce”; people with husbands and wives and lovers and children and aspirations and joy in life.
I live in rural France at the moment – it’s pretty much empty of people. If you live like a battery hen then I suggest that you move to somewhere which allows you to not do so. Check the population densities of almost anywhere in the world and choose a different lifestyle to enjoy the delights of life instead of moaning about how bad it is.
And, on another point, please, please could the writers here do a little research before making such incorrect comments about easily checkable facts. I refer of course to –
” …. particularly in this age of dwindling supplies of the fossil fuels needed to run it.”
The UK has proven reserves of at least 4 billion tons of coal and possible over 180 billions tons if we actually looked a bit harder. The rest of the world has about 130 years worth of proven reserves at 2015 levels of consumption and probably much more. We have a choice as always and I expect, if we approach the future with optimism instead of whinging at how bad it’s going to be, we will be humans and not puppets of stupidity.
Toodle pip
Most of the human population lives in a small fraction of the land area of Earth.
The population problem has two parts, numbers and resource usage rate. As subsistence farmers (up until the late middle ages)population is self limiting in the 1-2 billion range due to environmental carrying capacity. No problem (for the planet). Once you start using energy at higher rates(FF, but also intensive biomass and water/wind) the living standard goes up, innovation ramps up, and life expectancy goes up. The first two create a virtuous cycle and number one leads to higher birth rates, which in connection with number three leads to rapid population growth. That growth, coupled with the resource demand required to generate the improved lifestyles lead to environmental burden.
Our current set of crises has a similar dynamic. As lifestyles improve(even in Africa, most people are no longer subsistence farmers) life expectancy increases and fertility decreases, but fertility is a lagging indicator, and population lags behind that. It is true that the only ways known to purposefully decrease human population growth in a significant way are genocide and female prosperity. Unfortunately(or fortunately) the former is not currently under consideration, and the latter is only pursued erratically.
Increasing population with increasing resource demands has always lead to resource/waste problems, such as the horse manure problems in cities in the 1800’s and the whale oil shortages of the same era. Generally the solution to such problems has been the evolution of replacement technologies or the invention of mitigation technologies like wastewater treatment or vaccination. Generally, this has occurred at a rate that has allowed economic forces to drive the process with the resulting damages being borne mostly by poor people. Our current difficulty arises because some highly successful technologies (fossil fuels and fertilizers) have turned out to have environmental burdens which were cumulative and non-obvious. The success of these technologies also makes it difficult to change or move away from them due to political and logistic/economic considerations.
No one who has seriously studied the problem doubts that it is physically possible to support 9 billion people on the Earth with an acceptable lifestyle given our present to near term technology. The problems with doing so are mostly social/political. For example, the US alone grows enough protein and carbohydrates(corn and soy) to feed the world’s current population, if everyone were a vegetarian and food was distributed by need. Similarly, housing, communication, education, and energy are limited not by our economy’s ability to produce them but by it’s inability to distribute them to those who need them and it’s unwillingness to clean up after itself.
Climate change is a serious problem mainly because the political/economic elite refused to act until recently and still refuses to take strong action. We are currently in a race to see if the public can drive the degree of change necessary to avoid a situation where our current, highly interconnected, technological level becomes unsustainable. If we do not, our population problem will solve itself in a particularly unpleasant manner. If we manage to avoid such a collapse our population will slowly decline over several hundred years. I regret to say I put the odds at 60/40 against.
Sorry if this seems like a long recitation of the obvious but this thread, like so many others on these topics, is in danger of wandering away from the actual situation into the realms of ideology.
Almost every human invention has increased human producitivity, and thereby increased the number of humans the earth can support. You might as well oppose farming in general. That drastically increased the number of humans vs hunting/gathering.
This is the way to the greening of Mars.
But yeah. Earth? As mentioned, it’s not at all clear that the lithium approach will help when all costs are included.
With a concentration of a couple of percent in an atmosphere 100 times thinner it may be tricky making enough to green Mars.
Going from one billion people to eight billion is not necessarily a good thing. But, we’re in luck. Global warming, pandemics, perpetual warfare and stupidity will likely solve the problem. Then we don’t need as much ammonia.
Of course, ammonia based fertilization of crops is the apex of nutrition-free food production and a major catalyst of the ‘true’ organic fertilization movement (utilizing decaying plants and animal waste to fertilize, which produces substantially higher vitamin and mineral content in our foods).
I do like capturing hydrogen in the form of ammonia as a means of storing renewable energy in a smaller, more cost effective scheme than electrochemical cell storage (ala Tesla).
Didn´t Tesla have a similar idea?
in his publication >The Problem Of Increasing Human Energy< , one point was to make the nitrogen of the air useable.
As a side note amonia is also used as a refrigerant. Most food production facilities are cooled using amonia. If you are near a food production facility you may notice an orange windsock on the roof. That sock is to make sure you are upwind if an accidental release occurs. These facilities also have multiple staging areas for workers to reloctae in an accident. Amonia it not only grows the food it cools it down as well.
Another possible alternative to the Haber process is the photo-catalysis of ammonia from atmospheric nitrogen and water using titanium dioxide as the catalyst, :
https://patents.google.com/patent/US4113590A/en
I designed two variants of a photo-catalytic fertilizer generator based of this reaction :
https://www.furaffinity.net/view/35992966/
Shouldn’t we be able to get our guano from all those 20 billion chickens?
Look up the Guano Islands Act, US went to war over that poop. There is an island in the west indies that is in contentions today.
The chicken poop is not sitting there. It is being used as fertilizer at the rate it is being made.
Starting with biomass carbon and energy from whatever politically correct source you have:
CaCO3(s) → CaO(s) + CO2(g)
CaO + 3 C → CaC2 + CO
CaC2 + N2 → CaCN2 + C
CaCN2 + 3 H2O → 2 NH3 + CaCO3
Repeat.
And don’t tell me that it is not efficient, the waste heat and flammable gases that cannot be used on preprocessing the inputs goes to steam turbine generators and the ash from the biomass is mixed with some of the ammonia to form solid salts that constitute a perfect fertiliser to return to the fields that provided the biomass therefore closing that loop too. We could have been doing that for at least a century except for a lack of knowledge and the economic pressures of industrialisation driven by nation states wanting to produce explosives. See how that works?
From the article: “If the [Monash] method can prove out, it has a lot of advantages over Haber-Bosch. Chief among these is the lack of high temperatures and pressures, and the fact that the whole thing could potentially run on nothing but renewable electricity.”
From a discussion on CaCN2 production: “Calcium cyanamide [CaCN2] is prepared from calcium carbide [CaC2]. The carbide powder is heated at about 1000 °C…” Whoops! Full stop. Looks like ammonia production via nitrolime also requires high heat, much like H-B does. Monash is poised to avoid that requirement entirely.
I’ll say it. When a production method produces significant waste heat and unspent flammable gases, it is not efficient. Devising ways to mitigate or use those waste products does not change that core fact.
So this new electrolytic process depends not only on lithium, but also phosphorus? So far the lithium has had all the attention here, but hasn’t there recently been some buzz about phosphorus being kind of scarce, too?
Neat reading this. I was just yesterday thinking on the drive back from the other side of the State, that all the fields of corn seem like they can use a synergistic grown crop that’s a vine so concurrently sequestering nitrogen from the air and fixing into the ground whether or not with a probiotic to aid in moisture and nutrient retention, equilibrium. sourcing. This led me to wonder about the mass energy balance so to better understand the range of the systems potential metrics… like say for per acres worst case and best case scenerios.
Now, in regards to organic synthetic chemistry… I’ve not thought about process optimization in decades. Last leaving off with the wonders of the optimal molecular energy state energy sources to catalyze the reactions equilibrium and rates.
Another interesting topic I was thinking about yesterday and reminded of today was all the waste food somehow organized better for larger scale feeding or at least, sent to dedicated large scale mulch pits for sterilization (temp of the mulch pit so ideally no energy inputs required) for use in fertilizing.
Was thinking also, that seems almost that waste water treatment facilities can do this process more economically since the infrastructure already exists to transport and centralize the processing. The concern would be the enzymes or biotics used as well as filtration processes to clean the water and segregate the nutrient rich soil fertilizer/amendment stream.
The author wrote: “[W]e’ll also take a look at ways to potentially decarbonize our nitrogen fixation industry in the future.”
While reducing energy usage is commendable, the nitrogen fixation industry poses an equally serious problem: runoff into waterways and bodies of water. In 2009, a group of scientists from around the world identified “nine planetary boundaries” ( https://en.wikipedia.org/wiki/Planetary_boundaries ); exceeding one or more of these boundaries could be catastrophic. Climate change is just one of the nine boundaries.
“Biogeochemical flows” is another boundary which especially involves human-wrought changes to the natural/pre-industrial nitrogen and phosphorus cycles. In the Wikipedia article:
>>> Since the industrial revolution, the Earth’s nitrogen cycle has been disturbed even more than the carbon cycle. “Human activities now convert more nitrogen from the atmosphere into reactive forms than all of the Earth´s terrestrial processes combined. Much of this new reactive nitrogen pollutes waterways and coastal zones, is emitted back to the atmosphere in changed forms, or accumulates in the terrestrial biosphere.”[32] <<<
"Boundaries" for some of the boundaries have not been determined yet. A "boundary" is the low end of an understandably uncertain range; the range includes a "tipping point" at an unknown location — all of the planetary boundaries affect and are affected by the others and it is not known how they will interact. For example, the land-use planetary boundary (e.g., conversion to agriculture) affects climate change, the N/P cycles, and biodiversity loss … and probably a couple of others.
So we are not out of the woods yet. (No pun on plants intended!)
Those stats make it sound like a fairly small price to pay (in carbon) compared to the massive increase in food production that fertilizers have enabled. Don’t get me wrong I’m all for increasing efficiency where possible, but 2% world energy use is pretty small compared to what it gives us.
What is really cool is that ammonia can easily be used to create hydrogen. Hydrogen storage is a dangerous thing, especially on something that moves. Right now, there are more and more tug boats equipped with ammonia to hydrogen plants onboard, to create hydrogen. That way, you don’t need to store the dangerous hydrogen under super high pressure. It’s safer, it’s cleaner than diesel and it provides plenty of power. I hope to see cars equipped with this in the future. It would be a game changer. No more charging networks, no need to wait 30 minutes to fill up your car with electricity. Start to pump and you are done after 30 seconds, just like with benzene/petrol. It would solve all the big problems people have with electric cars.
I’m a Kansan, recently one my State’s Senators Dr. Quack Roger Marshall, but out a rant. In his rant, he stated ” At this rate, Europe will starve themselves before they die from pollution”. Ignoring That fertilizer production, depends on finite resources. My read of this article article and view the video, this “greening does as well.
Haber-Bosch was a milestone that became a millstone.