At its core, the concept of vehicle-grid integration (VGI) – also called Vehicle To Grid (V2G) – seems a simple one. Instead of a unidirectional charger for battery-electric vehicles (BEVs), a bidirectional charger would be used. This way, whenever the BEV is connected to such a charger, power could be withdrawn from the car’s battery for use on the local electrical grid whenever there’s demand.
Many of the complications with VGI have already been discussed, including the increased wear that this puts on a BEV’s battery, the need for an inherently mobile machine to be plugged into a charger, and the risk of needing one’s BEV and finding its battery to be nearly depleted. Here the cheerful marketing from Nissan and that from commercial initiatives such as Vehicle to Grid Britain makes it sound like it’s a no-brainer once those pesky details can be worked out.
In parallel with the world of glossy marketing leaflets, researchers have been investigating VGI as a potential option for grid-level energy storage. These studies produce a far less optimistic picture that puts the entire concept of VGI into question.
The Demand Vs Supply Puzzle
In most VGI scenarios it is assumed that there are independent stakeholders, with the participation of the utility companies, VGI service providers, electric vehicle owners and possibly the government. When studying this scenario from a South Korean perspective, Moon et al. (2021) came to the conclusion that the economics of VGI are unlikely to work out without government subsidies. The essential problem is that with the overhead and logistics involved, it is exceedingly hard for any of the stakeholders to run a significant profit, if any at all.
What is fascinating about Moon et al.’s analysis is the description of the Korean energy market, which features both public utility companies as well as two tiers of demand response (DR). Korea’s Type I DR applies mostly to larger industrial electricity users which receive incentives to reduce their load when asked to. This is a required response, with fines if the demand is not reduced when asked. Type I DR contracts have a maximum number of hours and number of calls.
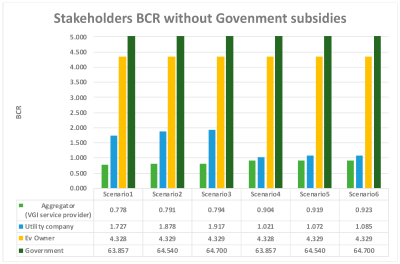
Type II DR is a demand-side bidding program involving interested parties submitting load reduction offers. Here too, penalties apply if the requested demand is not reduced as offered. Parties can participate in both Type I and Type II DR, but Type I takes precedence over the latter. In many ways this DR system can be considered to be a kind of negative load-following, as well as peak-shaving system. The main difference is that instead of the utilities adding more supply to the system, demand is time-shifted or reduced.
In Moon et al.’s analysis, when considering VGI in the context of this system, it is exceedingly hard for the stakeholders to break even. Increased participation in the VGI scheme through a pooled VGI service provider improves matters, but a guarantee of profit is impossible to give for the VGI service provider without government subsidies. Perhaps ironically, there’s also an increased chance of profitability when the cars involved are used less often as cars. This notion has some led to consider an alternate take on VGI, using the swappable battery systems which are already in use in some places.
Herding Batteries
Although it is very much true that cars spend a large part of the time standing around, even in the case of BEVs, this is unlikely to always be in a location where they are continuously plugged into a charger, let alone a VGI-style bidirectional type charger. Many people will have their BEV out on the driveway, or parked alongside the road. But if you pull the battery out of the car when parked, you end up with something like a battery swapping station (BSS) that also provides grid-level storage services, as proposed by Zeng et al. (2020).
The convenient aspect of such a swapping-based system is that each BSS can maintain a pool of batteries, using the majority of these batteries fully for grid-balancing, while keeping a number of fully charged batteries ready for insertion into an EV. The mostly discharged battery from this EV is then added to the pool.
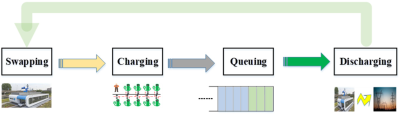
While avoiding the issues with BEV usage patterns, including their essentially random connecting to and disconnecting from the grid, the obvious issue with a BSS-centric system is that very few electric vehicles feature a swappable battery. To this day, it is a feature that is most commonly seen with e.g. taxis in countries like Japan and China.
Basically A Daft Idea
That VGI keeps receiving so much attention is rather astounding in light of the messaging from experts, such as J.B. Straubel did in 2016, back when he served as Tesla’s CTO. As covered back then by CleanTechnica, J.B. makes it clear that only ‘smart’ dynamic charging — charging mostly when demand wanes — makes sense. J.B. also shot down the notion that old BEV batteries can be reused for grid-level storage, noting the very different charge/discharge cycle requirements for a BEV battery versus that of a grid-level storage battery.
Dynamic charging should be easier to get BEV owners to sign up for. Charge rate is most important when traveling and stopping to recharge. When charging overnight, the only important aspect is whether the battery is full when getting into the car the next morning.
All of this seems to point back to the same issue: the conflict between trying to use the same battery for two diametrically opposite use cases. A BEV owner, when left to their own devices, has no interest in feeding power back into the grid, other than perhaps to their own home in the case of a power failure. This then leaves a financial incentive as the only reason for a BEV owner to participate in a V2G scheme.
Yet the most profitable way to benefit from a V2G scheme is to leave one’s car hooked up to a charger 24/7 and not use it as a car. At that point, however, you’re just buying the batteries. All of which serves to underline the reasons why V2G has such trouble making a solid business case, and instead keeps fumbling from trial to trial.
Smart Charging UPS-On-Wheels
When taking the available evidence into account, the case is easy to make that for utility and grid providers, the ‘smart charging’ and demand response aspects are both attractive and easy to justify. Much like with the large-scale DR schemes in South Korea, consumers might be enticed to let the utility regulate the charging speed of their BEV, especially for some compensation. For utilities, this could help with smoothing out the glut of demand that would occur when people arrive home after work, plug in their BEV charger, and turn on the airconditioning on full blast.
For BEV owners, the bidirectional charger makes some sense when it means that in the case of a power failure, the idling BEV in the garage could safely feed the power in its batteries into the house grid. This is a feature that is gradually becoming more advertised in advertisements for new BEVs. Unlike the uncertain prospect of selling BEV charging and discharging cycles to the local utility, using one’s BEV as a fancy alternative to an emergency power generator makes intuitive sense.
VGI may just not be the panacea for grid-level storage that some made it out to be. Although BEVs can perform simpler tasks like grid frequency stabilization relatively well since these tasks require a relatively small battery, it could be argued that installing a dedicated battery in the grid is more economical than hoping that enough people plug in their BEVs at the right times to keep the grid from desynchronizing and plunging part of the nation in darkness, not unlike what happened with the Northeast blackout of 2003.
With how complicated a national grid is to begin with it, it’s perhaps for the best that we do not seek to somehow integrate anything with a battery into it, especially when said battery can literally drive off at any moment.
The back and forth losses alone make it a daft idea. The losses incurred when charging the car batteries are rarely discussed, and when they are discussed they are dismissed as not relevant. Until those losses are calculated properly and the overall CO2 contribution they cause are fully understood, we shouldn’t be talking about battery energy storage. Gravity and water based storage is a better alternative.
Don’t forget Compressed Air stores too – but all of these ‘better’ alternatives are not really better, just better for some situation – and the ‘losses incurred when charging the car batteries’ are no different to the losses of any other energy transfer – batteries are often actually the highest in input power to output efficiency (but it is all situational).
But with losses in each direction, using vehicles as storage for peak demand is paying twice and putting more cycles on what is already a limited charge resource. Water, Gravity, and Compressed air might need a valve or pulley replaced, but that’s a far cry from replacing the actual cells that store the power.
Agreed the longevity and maintenance costs of whole thing is also something to account for, and there along with the geography required CAES is for me the current best option – scales well, can be put basically anywhere, can be very efficient but can also supply huge peak loads (at lower efficiency), so it is highly versatile and should be cheap to build and maintain.
But you are ‘paying twice’ no matter what type of energy store you use, as there are always losses in both directions – unless you are actually directly using the mechanical power of the wind turbine etc to pump the water uphill its still source to electric to grid to store and then store to electric to grid to demand – the store can be a BEV, a massive Grid battery, the pumped hydro it makes no odds you pay the conversion twice. So there all that matters is the in/out cycle efficiency, and batteries can be very much up there in that regard (though so can all of the others mentioned already and even flywheel energy storage – and which is best will be very situational).
The main challenge for CAES in terms of efficiency is maintaining isothermal conditions.
If you pipe the air through a body of water, the water has enough thermal mass that it keeps the temperature almost constant, which means you can take the PV = nRT and drop the T term out, which removes the hysteresis losses between charging and discharging the pressure reservoir.
Dude isn’t that the wrong way round? By cooling the air you’re removing energy. Surely it’s better to insulate the store and keep the gas hot? THen more energy will come back out when it’s released.
>Surely it’s better to insulate the store and keep the gas hot?
Using water as a thermal mass, you get the lost energy back because the gas cools on expansion by the same amount, so it draws the heat right back out of the water. The smaller the temperature difference between compression and expansion, the smaller the heat loss into the environment becomes.
It would cause a reduction in life span of the battery packs on the vehicles.
You’d basically be stealing from the car owners unless they were compensated in some manner never mind the increased pollution from having to replace them more often.
>just better for some situation
Such as for scaling up at lower marginal costs without overtaking the entire world’s supply of certain very expensive minerals.
You think of battery losses and in the same moment want compressed air storage? Don’t forget, that due to thermodynamics the efficiency is much lower. Compressed air is an (energetically) expensive energy storage or transport solution.
Charging efficiency is about 85% (it varies between 80% and 90%) and is quite well understood.
However, gravity/water based energy storage is a pretty terrible solution if you don’t already have a dam in place. It changes the local environment which means you need a four-year environmental study. On top of that, it can’t be installed just anywhere. It’s pretty great when it works but overall it has far too many restrictions to be widely used.
Lithium battery charge/discharge efficiency is much higher than water/gravity. You lose 10-15% in a battery cycle vs 30-50% in the others.
Lithium battery manufacturing costs between 3-10% of the lifetime capacity throughput (ESOEI 32:1 to 10:1) so use patterns greatly influences the overall system efficiency. The lower end of the estimate applies for batteries with a nominal endurance of about 6,000 cycles and the upper end at 2,000 cycles. Generally speaking it’s in the ballpark of 200 full charge cycles. Which is a huge amount of energy. It’s about the first 60,000 miles of a typical Tesla car.
This is a “hidden” cost that people leave out of their estimates on battery system efficiency because it’s hidden in the fossil-fueled manufacturing and supply chain. Of course if you beat the battery to death as soon as possible with maximum throughput, then your penalty is only that 3-10% loss, but if you spare your batteries then you’ll hit the calendar life limit before you hit the cycle life limit, and your efficiency goes down. Less actual throughput means the ESOEI goes down. It’s again a point of conflict with any V2G system: to make it efficient, it has to kill your battery.
Compressed air and pumped hydroelectricity have ESOEI numbers above 700:1 which means it costs negligible amount of energy to build them compared to the potential energy throughput.
For example, take the latest NMC cells rated at 3,500 cycles. This is the Tesla “million mile” battery. If you have a 100 kWh battery and you use 10% daily average for driving, and another 10% for V2G applications, that’s 0.2 cycles per day. The battery would have a cycle life of 48 years BUT it has a calendar life of about 12 – 15 years max, so you can only attain a third of the nominal throughput.
Back to the estimate: 200 cycles cost over 1,100 cycles is about 18% loss in manufacturing. Factor in 85% efficiency in use, and your system efficiency is 67% which isn’t actually better than CAES of hydro.
On a simple estimate, you should increase the V2G load on the battery to about 60% capacity per day to reach the maximum cycle numbers within the estimated calendar life, but in real life this wouldn’t work because the calendar life is also reduced by the cycle loading (and vice versa), so it’s a complex optimization task. In any case, you couldn’t use more than half your battery for yourself because it’s in daily use, so why pay for it?
On reason you might pay for the bigger battery that isn’t usually used for community is the simple convenience – you CAN use it when you want to – tell the car its got a long trip to do and it won’t do V2G, then you can do that extra long trip a smaller battery EV couldn’t do.
damn we need an edit button, or I need to stop trusting and pay more attention to the spulling checker….
community=commute!
>the simple convenience
Another reason to pay for a bigger battery is the ability to choose when you recharge it and when you use it. For example, the power prices here have been pegging up towards 50 cents a kWh thanks to wind power and Russia, so it would be nice if you could just not charge up for a week or so and still drive normally.
At my old house, power outages were a very regular occurrence. Had we not moved, we definitely would have bought the Ford F-150 EV (we also would have benefited greatly from owning a pickup). Our new place is on a much more reliable grid, and we don’t need as many building supplies any more, so neither factor a huge consideration for us now, but there are definitely people for whom personal energy storage in an EV makes a massive amount of sense.
Exactly. If you can ‘island’ your property it makes sense for the property owner, but no profit for the utility companies (which is probably why they aren’t interested).
Also if you can charge the EV from renewables in the first place (solar, wind) then it also makes sense from a carbon offset/avoidance perspective.
Why buy a brand new EV when you can pick up a wrecked one that still has it’s batteries…
My biggest grip with a BEV is the inconvenience of stopping to recharge on cross-country trips. The best batteries can still only last about 4 hours at highway speeds, and I routinely drive for 12 or more hours up and down the USA’s East coast. I highly prefer the ability to fill up a tank within 5 minutes when compared to sitting at a charging station for 45+ minutes.
I’d love to see a BEV that could be turned into a hybrid just by swapping out the battery pack (or a portion of it) with a generator. Even if the range were shorter or it reduced storage space, it gives some distinct advantages. Plus if that capability gained support and standardized interfaces, a market could develop around the use of various fuels/engines/fuel cells or even replacement battery packs. Competition should bring costs down and capabilities up, but getting auto manufacturers to agree to a standard would be… tough.
Yeah, you’re right. That’s a pipe dream. I’ll just bolt a fuel transfer tank and a Miller welder/generator in the back of my F150 Lightning, hook it all up, and hit the road.
You can sort of get that as it – there is a whole slew of plug-in-hybrid that have a pretty large battery, large enough for any in city drives and short hauls you won’t need the ICE, but still have the ICE and that quick fuel stop it brings when you do need to go further – its not quite modular as you suggest, but with the weight and size such an engine and battery swap would be that really wouldn’t be practical without a real garage to do the conversion in… Batteries and ICE power units (plus the fuel tank etc) of any size are rather heavy…
Glad to hear that you continue to ruin our environment because you don’t want to be inconvenienced. Thanks, Pete.
Pete’s needs for a long-range vehicle aren’t being met by electric cars yet. Don’t get all holier-than-thou because you might not need to drive as far as he does.
Electrification doesn’t need to be an all or nothing approach. Electric cars are making good inroads into the market, especially the early adopter market and those especially concerned about the environment. This growing market will reduce the tailpipe emissions from internal combustion engines as they get popular and occupy a larger percentage of the vehicles on the road.
There will be a need for internal combustion engines for a very long time because none of the alternates (so fare) are a drop-in replacement for them. The need will reduce as people move away from IC engines slowly as the cost of fuel increases over time due to market pressures, regulation, and future reduced demand. Also, electric vehicles will slowly get better at filling these needs. This will take a while, and that’s ok.
You younguns and your “long time”! We’re talking like a decade.
All of the big European carmakers have declared that they will stop producing internal combustion engines in or before 2035. None of them have plans for a new ICE design before then.
If you take good care of your current ICE vehicle, it could easily be your last.
That will change soon as it becomes apparent that people simply can’t afford to buy EVs.
Besides, the half-life of a vehicle on the road is about 12 years anyways, so from now till 2035 only about half the cars will have been replaced by EVs – assuming 100% new cars sold from today on would be. This won’t be the case until the bans take effect.
Since about 70-80% of the market for cars is second hand, come year 2035 it’s still going to be the case that most cars on the road are running on fuels. If the politicians insist on the current course, then what’s going to happen is a two-tier society where the rich 20% drive BEVs and the rest are mending old petrol cars.
This is because, for anyone working middle class and below, money now is much more expensive than money later, so the higher up-front cost of EVs makes them unattainable. There is also the prohibitive cost and safety limitations on DIY of battery replacements which mostly keeps them out of the second hand market.
One thing you don’t account for Dude is that the wealthy 20% won’t keep their existing EV, as they need the latest model to keep up with the Jone’s – that’s a huge amount of perfectly serviceable EV on the second hand market that means in relatively short order most middle class folks will be able to afford one (if they can’t just buy a new one – which with how cheap many EV models are getting isn’t a certainty).
And some time after that with how many really good chassis, motor, etc are being renderd useless by the failing battery the popular models will get off the shelf replacement battery, and the whole industry to change them cheaply pops up because there will be heaps of money to be made.
“the half-life of a vehicle on the road is about 12 years anyways”
That’s more down to consumerism than it is the ability of the vehicle.
If you take countries where they change their vehicles often vs countries where showing off, look at me me me and keeping up with the jones’s is seen as crass, they tend to drive older cars.
He in the UK we have people that have no job, live on government benefits and yet still manage to drive around in a brand new car cos it “only” costs them £150 a month to rent it
>That’s more down to consumerism than it is the ability of the vehicle.
Not really. Perhaps the word “half-life” was misleading: the average car is about 12 years old, the average scrapping age is about 14-17 years where most cars will develop some problem that costs more to fix than buying another used car. After that, there’s a long tail of “survivors” that hold on to 20+ without major drive train or rust issues, usually cars which haven’t been driven a whole lot in the first place.
>the wealthy 20% won’t keep their existing EV
Would you buy one from them for half a wooden penny if you knew it needs a $25,000 battery replacement two or three years later? If the car’s already a decade old and out of warranty, you can’t expect much.
> the popular models will get off the shelf replacement battery, and the whole industry to change them cheaply pops up because there will be heaps of money to be made.
Yeah, except for the problem with the materials supply chain where increasing battery production dramatically in the next 10-20 years will put the cell prices up, not down. The industry has to pay for the expansion somehow and the faster they expand the more it will cost you.
Dude the wealthy that simply must have the latest model won’t be getting rid of it at a decade old, be lucky for it last 18 months in their care I’d think, certainly it won’t last more than a few years – heck Dads most recent car was 3 months old second hand or something stupidly short like that when he got it around a decade ago…
So yes I would buy one for way more than half a wooden penny, its going to have at least half its life left, of in my case probably entirely fuel cost free motoring (solar panels) – so a potential battery change in some years time is probably long after its paid that any extra upfront cost and possibly saved enough to pay for the battery change too – though of course that depends on just how many miles I would do and how stupid petrol prices will become, which even after this crap with Russia is over I doubt it will be going down fast, and as it stands sadly I can’t see Putin’s murder machine coming to an end any time soon either so its probably going to get worse for a while before it even thinks about getting better…
That I barely have half a wooden penny at the moment makes the whole thing a rather pointless thought experiment, but if I could I would as it stands, heck I’d even take one I know needs a battery replacement right now – rip out the old one and fit whatever capacity I can get in those small battery units folks have been electrifying their classic cars with myself…
> its going to have at least half its life left
In that case it’s not going to be cheap. The cars that are traded after just 2-3 years are still very expensive because the whole point of the scheme is to sell the car on while you can still get 70-80% of the money back. That’s the only sensible way to buy cars that cost 50-100k and up.
Many people still need to take loans to finance a 5 year old car. The point where cars start to become affordable for the unwashed masses is between 5-8 years of age at 10k or less.
The price of a second hand motor isn’t just a product of age, there is also how the previous owner treated it, and how desirable that model is – for very arbitrary and human reasons some cars are near worthless almost immediately as somebody of some influence says they are rubbish and it sticks…
Also when brand new EV can be had pretty damn cheaply if you want to have that less premium badge, without all the pointless shiny baubles or just simply the lower range smaller model from some of the luxury brands too – it doesn’t have to be turning a 50-100K range down to your 10K ‘anybody’ can have one range, its more like 20-40K for the new one in the first place, and having seen a BMW more budget end model in person it looks and feels like their usual engineering quality and the owner has been happy with (more like bubbling with earnest enthusiasm about it) it for while now, its not like these cheaper ones are bad…
Elliot, I think the newly made ICE vehicles on the road after 2035 will be mostly vans, trucks, and semis that require the long range and quick refill that liquid fuels provide. I agree that most of the new consumer vehicles will be electric shortly after 2035, but the market for used vehicles is strong and the products are robust.
My own vehicle is a well-maintained 20 years old, and when I compare it to new vehicles I see very few practical reasons to replace it until the costs of maintenance get higher due to age (probably in the next 5 years or so). New ICE vehicles are not fundamentally different than older ones if they are in good condition, which makes me think the used market for these cars will be much more robust than the used market for electric cars, as electric cars are still seeing significant performance changes from generation to generation. This might change as the technology matures.
I’d consider buying an electric car at some point. When I built my house, I wired it with two 50A charger circuits in the garage, because electric cars look to be in our future.
> All of the big European carmakers have declared that they will stop producing internal combustion engines in or before 2035.
Did they declared something about developing something much better than batteries that are obviously ruining all that nice and favorable EV idea? Fuel cells, may be? Or they are just eternal “next year” thing? May be something more advanced? No? What is the real point of that game with forcing people to pay absolutely inadequate money for the worst vehicle energy storage ever?
>10K ‘anybody’ can have one range, its more like 20-40K for the new one in the first place
18-22K is for regular/popular new cars. The mean value gets inflated by trucks, SUVs and luxury cars. I recently saw a study that actually estimated the average (median) road-going car (not truck etc.) to be valued at around 4-5k.
Nevertheless, the largest price drops happen in the first years and then it tapers off. After about 8-10 years, a typical car enters into that under 10k range and drives for another 10-20 years if so maintained. An EV does the same thing faster, and with a steeper fall-off in value at the end because the battery EOL is coming up and it’s not economical or affordable to “fix” it.
In other words, there is a relatively short window where a second hand EV is affordable AND driveable. People would have to change them like socks faster than the price of new vehicles drops, which means there won’t be enough cheap second hand EVs for the market.
Dude you have to be wrong on change them like socks for economical price reasoning – for the same reason refurbished I-phone etc exist there are millions of damn things in a busted state, but still in demand so its worth the labour and tooling up costs to create at least the common spare parts, and the tools to make the repairs easy and repeatable.
EV will be no different, now there is becoming a market the spare parts and garages that can do the battery swap effectively will start to exist. Though not commonly for a while yet, as the current crop of EV – the popular and truly usable mass produced ones still on average have at least 5 years till they start wearing out in any real number, and probably more like 10-15 years until there is enough wearing out to exceed the crashed battery salvage potential enough to really create the demand, but its going to happen (unless Putin’s Murderous Misadventures or some other global catastrophe take the demand away of course… Sigh, the world recently got even more depressing…).
Or put another way how expensive would repairing/rebuilding an engine be if you it wasn’t a mass produced casting, machined on specialist jigs in huge numbers but a person/company with a general machine shop making it all, which means making all the tooling that might been needed just for the one job too…
I’ll give you hint as you tend to be dense when it suits you, its going to cost more than the purchase price of the entire car, even a pretty high end car to pay the machinist a paltry salary (the sort of salary for their skills they would just bugger off) and make up for all the extra chips created by not having really good castings – which creates a very high stock ‘wasted’ material bill just to make the damn engine block alone, not even the whole working engine! Most likely anyway – some engine are simpler than others, and the sloppier required tolerance to make something that wouldn’t pass modern emissions rules would save significant time, but create a pretty shit product.
The wish for more convenience is one main drives for innovation. That’s the problem with the environmentalists: They question our convenient way of live and want to make life harder for us. Like some religious missionarys. The want us to worship their god of CO2 reduction and threat us with an invented danger of global warming. Humans do not have tho power to have significant influence on the climate.
To show off their new EV, Porsche did a cross the US drive. I don’t know if they set a time record, but they did set a charging time record. For the 2,834 miles (4.560 km) of the LA to NYC route they spent a total of 2 hours 27 minutes charging. That’s a charging speed of around 1.5 Mach.
The average trip speed using Tesla Superchrgers is about 55 MPH. The traveling public will not want to bring back 55 MPH travel. The solution is rented battery EVs powered by rented and easily swapped battery modules. RBEV vehicles would cost about $4000 less than gas powered types and have all the comfort and performance advantages of standard expensive big built in battery EVs. A national network of about 300 swap stations with an agreed standard battery module would do the job. Everyone could use the same standard module design. At 25 kWh per charged module a sub compact car might use one around town and 3 on a trip. An over the road 18 wheeler might use 2 dozen modules at each swap.
With all the draconian speed limits in most places globally I can’t see the average isn’t already near as makes no odds 55MPH for any vehicle, not that I understand how can you have an ‘average trip speed’ that is in any way meaningful?
I can’t see how you can ever create such a statistic that is at all representative of living with an EV vs ICE – as most trips will have plenty of city/town elements with the low speed limits, and even if you are on longer trips where most of it is the bigger roads those still have speed limits, its not unusual for motorways to be actively limited to 50 or 60 here for various reasons, combined with the fact that almost every EV has more range than MOST folks drive in a day anyway – most people would almost never need to hit the ‘Supercharger’ on a trip at all! So the average is just however fast traffic was flowing on that road at that time…
And if you are working in one stupidly long entirely motorway crawl that will need stops and only considering that one journey you are being rather unfair to the EV to imply that its ‘bringing back 55mph’ travel – as the ICE power units you MUST waste time and fuel actively driving to the petrol station, all the time – you can’t have the magic electric fairy fill it up while you slept, the detour for which could easily be considered 0 MPH or even negative as you are going the wrong damn way, putting wear on the vehicle for however long it takes which would tank the ICE’s average (whatever that may be) damn hard too…
Clearly the integral Battery EV won’t suit every current usecase and user, and battery swapping is an interesting approach to take to accommodate some of those, but its far from the only one. Having pantograph like tram/trains do on the slow lane of motorways is being trialed for instance, which seems to me like a vastly better solution for most users that the integral battery EV doesn’t suit, there is also the Fuel cell (still as far as I know a few fairly big problems with the tech making it unfit for mass vehicle deployment, but its got a great deal of potential and if you have the funding it does work right now), and you could still have ICE powered hybrids – though probably in future a gas turbine generator style (lighter than pistons, pretty efficient, more suited to being a primary electric vehicle with combustion backup than the hybrids that use the electric motor more like a supercharger just to boost acceleration of a primary combustion engine).
After the tsunami that brought on the Fukushima disaster, the Nissan Leaf, which already has v2g capabilities, and a commercial inverter box available, was used for medium duration (weeks to months) backup power until missing sections of power lines could be replaced.
They would drop one of the inverter boxes next to a small cluster of houses, that were up to 10km from someplace that still had power, and people connected to their equivalent to FEMA, would drive up in a charged car, and drive the now near empty one back to a charging depot.
As for conversion losses V2G compares very favorably with other storage schemes like pumped hydro.
This is a sad and great tidbit the news never shares…
Sad? I think its a bit of brilliantly rare good news around a tragedy – a solution that works and the communities supporting each other, a real proof that humans are not all that bad (all the time anyway), and really will pull together and use what they have to get one with life!
Works if you have power within 10 km and two very expensive cars that can shuttle back and forth – and two people to drive them. The complications of this scheme make it a rather marginal solution, as opposed to a diesel generator in a crate and a tank of fuel that costs a fraction and can be delivered anywhere.
No arguments there, its not the easiest method for all situations, but it works if you have them and scales better to wider areas taken offline than diesel generators for the repair work duration, as that is a heap of fuel to transport and use pretty inefficiently in small genny and lots and lots of small generators too – where transporting electric between the nearby places that have it and don’t in self mobile battery lets the spaces that are still on the grid support huge swaths of the cities/towns/suburbs that are disconnected and not need the daily fuel trucks.
Its clearly not the solution to use, even if you have the EV, when powering anything over long distances from the grid, but when you have a badly shattered local grid bridging the break over short distances it looks like a winner of diesel generators – as there you need a much wider infrastructure to ship the fuel, and probably more people involved – one person can drive the full car and limp an empty back many many times a day, but the fuel that would need shipping around will need multiple fuel trucks probably – as the source of the energy is so very much further away (not to mention in disaster recovery the diesel might well have more important demands on it – like the heavy construction vehicles).
> that is a heap of fuel to transport
Not really. A 1000 liter tank fits in a small trailer and will produce in excess of 3500 kWh of electricity. That’s actually a year’s worth of power for a single apartment discounting the heating/AC.
>the fuel that would need shipping around will need multiple fuel trucks probably
An average American household would need no more than 48 liters of diesel fuel to run a generator for a week for all the electricity – which about as much as fits in the tank of a diesel truck. A single fuel truck will carry 30,000 liters, so enough for 625 households.
Don’t under-estimate the energy density of diesel oil.
Not under estimating the energy density but when you have to ship it in and distribute it over huge areas, from a very long way away (which on average it will be as refinery and bigger storage tanks are very centralized), and leave enough fuel at every stop to last till the next fuel truck can come around. That is potentially only one trip serving a fairly small area, that probably takes more than a day with the long travel to get there and then dispense the tanker of fuel in drips to all the places that need it (hence the suggestion you need many trucks – not because they can’t carry enough, though that might be true, but mostly because the turn around time and man hours involved in all the dispensing is high) vs a little shuffle over short distances to bridge the short gap between the connected and disconnected grid elements, which is 10 mins of work probably only once or twice a day – its not at all cut and dry, and the EV to me looks like the winner there.
I think you only need one person to drive them.
But he must never leave the fox alone with the chicken.
Current pricing for the leaf puts it below the median U.S. new car prices. Not cheap, but battery prices keep dropping
And you only need one driver, who can deal with multiple locations. They drive the charged car there, and the empty one back.
> when you have to ship it in and distribute it over huge areas
Yeah, there’s already an infrastructure in place for that: fuel service stations.
Dude a fuel station isn’t going to be able to deliver to the heaps of individual generators in a disaster response situation, it probably doesn’t have the fuel capacity to handle that load anyway – the tanks will run dry so much faster when everyone needs to burn fuel to get fuel to burn and thus power their homes.. And that is if it is even able to operate at all till the power is back online in its area…
Then even if you assume it is working that is still many more man hours as the big tanker has to go to the station, and then hundreds of individual petrol cans must be filled, distributed and then emptied into the generator, or other fuel trucks take it straight to the generator (in which case why have a middle man) – it is a vast amount of work vs driving a short way and plugging something in!
>heaps of individual generators in a disaster response situation
What is a modern hybrid car?
>it probably doesn’t have the fuel capacity to handle that load anyway
It’s already stocked for weeks of regular demand. That’s plenty enough to bridge the gap, and most people will have their fuel in their tanks anyways.
The quick duck answers: “a typical gas station has 30,000 to 40,000 gallons in underground tanks”
A random station at a random time would have about half that. 1 kW is about 1/10th of a gallon per hour or 2.4 gallons per day, so a regular fuel station has about 8,000 kilowatt-days of emergency generator fuel supply available.
There are about 168,000 retail locations that sell fuel in the US, which is roughly one for every 2,000 people, so there’s already enough of a fuel stockpile in gasoline stations to give everyone a kilowatt of power for four days – plus whatever they already have in their cars.
Of course not every individual needs a kilowatt – that’s more of a per-household scale. I personally need about 200-250 Watts. If I had a 160 liter drum of gasoline and a generator, I would be set for well over two months.
What is a modern hybrid – a perfectly usable mobile generator, if you have one…
And of no use at all if it doesn’t exist or isn’t where you need it when you needed it. So when you have battery EV and a nearby working charge point you end up with the same damn argument its a perfectly good mobile power source, if you have them, which they obviously did as they used them!
4 days of power per person… and yet Dude here in the UK at the mere suggestion fuel might become short at one point last year? year before now? the ques gets stupid and the tanks at the petrol station go dry instantly, and can’t get filled fast enough, and that is where there isn’t actually any extra demand on the fuel!!!! It is just folks all choosing to fill up at the same time.
The same thing would happen to the fuel stations in a real emergency recovery situation, just MUCH, MUCH, worse as there would actually be more demand for the fuel, assuming they can actually pump the fuel at all with no power – in which case yeah they are stocked with fuel to the end of days, as you can’t actually take any away…
p.s. I suspect it wouldn’t be nearly 4 days per person most places – you used USA as an example I expect they need vastly more fuel at each fuel station or per head than anywhere else in the world because the USA has a far greater number of vehicles that think 5 MPG is crazy good efficiency… I wonder how hard you would have to work to find a vehicle in Japan that doesn’t in the real world best 30mpg at all times…
Jeff, do you have a link for that? The Nissan Leaf was only released in 2010, and the tsunami happened in early 2011. There just weren’t that many of them around, and those first-generation Leafs only had a 24kWh battery. I’m having trouble making the maths work, especially if one Leaf is powering a “cluster of houses.”
The nearest thing I can find with a quick search is this article from 2018 about used Leaf batteries powering streetlights in Fukushima, which is nothing to do with v2g: https://www.cnet.com/roadshow/news/nissan-leaf-ev-batteries-power-fukushima-streetlights-reborn-light/
Personally I think there is better options for grid based storage than vehicles.
One simple one is to just use lead acid cells and treat them properly. Then they last a good few thousand charge cycles and typically it is the carbonate case that ends up cracking before the cells wear out. However, when most people hear “lead acid battery” they think of a car battery containing 6 cells with no cell balancing and put under frankly abhorrent charge and discharge situations, in an environment where over discharging isn’t uncommon, usually by leaving a light on overnight. Lead acid batteries hates to be discharged bellow about 1.85 volts per cell. In a grid scale installation, over discharging can be avoided. And lead acid enjoys being fully charged 24/7 for decades, unlike lithium batteries that lasts a decade.
Another storage medium is pumped hydro, but finding good locations for this is hard, and a lot of good locations are in use for this already. Though, I have seen some proposals for using a cave/tunnel as the storage tank instead. (ground water leaking in will though create a bit of self discharge. But the open ponds on mountains evaporates so they too self discharge slowly over time.)
Then there is compressed air energy storage. A rather debatable technology that usually gets beaten down by people looking at their lousy shop compressors’ laughable efficiency, as if high efficiency air compressors didn’t exist. And compressed air is a fair bit higher energy density that pumped hydro. All though needs to be built on a similar scale (in terms of energy capacity) to make economic sense. Then there is the technical issues of pressure vessels, but high pressure tubing for natural gas is a somewhat cheap and safe storage container. And personally I think pressure vessels is the main issue with compressed air for grid storage.
Some talk about hydrogen as an energy storage medium.
But using electrolysis to make hydrogen is rather inefficient. Fuel cells are though more efficient, but round trip this ends up at around 30-40%. And that is when one aims for efficiency above cost. So in comparison, vehicle to grid is likely more economically viable. And the other three stated here aren’t any worse.
Then there is the alternative energy sources.
Biogas can for an example be extracted from sewage treatment plants, composting facilities, and landfills, and biogas is easy to store, has little to no self discharge unless the tank leaks. And can quite efficiently be burnt when needed. As a backup for peak demand or when other sources are at a low, biogas isn’t that bad as an option. It is also quite energy dense putting everything above to shame.
Hydrogen is though storing 100 MJ/kg compared to methane at 55MJ/kg, but methane weighs 100 kg/m^3 at 125 bar (5.5 GJ/m^3), while hydrogen weighs 45.8 kg/m^3 at 900 bar (4.58 GJ/m^3). So hydrogen doesn’t even make sense here. (Though, we can technically also include the energy stored by compressing the gas. 60.35 MJ/m^3 @ 125 bar and 612.2 MJ/m^3 @ 900 bar, and this is more or less not making up for the difference. But pressure vessels are rather expensive. Especially when one asks them to contain 900 bars with a suitable safety factor.)
Some have though looked at gravity based storage. Literally lifting weights.
Problem here is the low energy density. Per kg we only get 9.8 kJ for every km we lift our kg into the air. A 1 cubic meter block of lead weighing in at 11340 kg would only give us about 1 kWh of stored energy for every 35 meters we lift it, not including inefficiencies of our lifting mechanism. But a construction crane that most people depict isn’t all that efficient. However, lead is 11 times denser than water, but water is a lot easier to pump.
I have seen some people look at molten salt.
Just use a big resistor to heat it up to 600-1100 degrees C (depending on the salt), and then keep it very very well insulated. When you want the energy back, have some pipes surrounding it for pumping water through, you get steam and run a turbine. Simple, but it sure is a lot of molten likely somewhat corrosive salt to have around. And if the Boston molasses flood, or the London beer flood should teach us something, then it is likely that large containers storing liquids has some risks involved. Though, this technology is in use currently and has actually proven itself useful. Though usually at concentrated solar power plants.
I have seen some look at the feasibility of electric freight trains and scheduling them to run downhill and use regenerative breaking at peak demand hours, and uphill during low demand hours, the freight needs to move regardless so why not plan it a bit. Though, with sufficiently high electricity prices they will do this naturally, unless diesel is cheaper. But how much energy this can store, and to what efficiency is debatable. But as stated, the freight needs to move regardless.
There is however many more interesting energy storage solutions, I likely missed a fair few. Know of any interesting ones to add to the list?
At least I haven’t seen too many people postulate gigantic well oiled clock springs yet…
In short its an almost cost free and instant method for the grid to be able to massively decarbonise and still deal with the spikes of renewable energy better. Will not need as many (if any) massive expensive energy storage plants built to be able to turn off (well mothball probably) many more fossil fuel power stations.
Because everyone near enough needs personal transport to live (at least in many areas of more developed nations were such power grids that can benefit from V2G exist), so would need to have a vehicle anyway, hopefully many of which will be EV – that is a truely vast volume of batteries…
Which thanks to the huge scale, even more so if every vehicle was replaced with an EV connected to V2G you could take less current draw and energy out of whatever percentage of them are sat at a V2G charger – to the point its approaching zero wear on the battery and by the nature of such a huge distributed network get huge amounts of energy, probably orders of magnitude more than any primary power station outputs… (Will be very varied by nation, number of V2G chargers etc and of course everyone having a suitable EV will probably never happen, and certainly won’t happen overnight)
In the longer term to really develop technologies and expand renewables enough to cease all fossil fuel use the grid scale energy storage plants are almost certain to be required (though increasing efficiency and reduction of demand might negate that), and the methods that will get used will vary based on the needs and resources of the region. As I’ve said I quite like the CAES method, as its so very cheap, versatile, safe, and can be put damn nearly anywhere at almost any scale and still be efficient/effective enough, so I expect it will be one of the more used methods, but its not some magic cureall method that wins on every count…
The cheapest per kWh storage for compressed air I have figured is to use a TBM and drill a tunnel about 80-120 meters down, line the inside with steel plate to keep it air tight, and then pressurize it to 120-160 bar. Giving 16-22.5 kWh/m^3. Tunnel boring is relatively cheap if done in solid rock. But this requires fairly good geological conditions and wouldn’t be ideal everywhere.
Another solution is just high pressure natural gas piping, that is typically rated to 130-140 bar + safety factor. So storing 120 bar is safe, and gives us 16 kWh/m^3 of storage, but would require quite a bit of piping, though we can stack the pipes in a warehouse. Since then they are protected from the element and would be easy to inspect and service when needed.
Out of all the large scale grid storage solutions, compressed air is the one I think will be most practical.
Building efficient air compressors isn’t too hard. And if one keeps the process isothermal one can reach relatively high efficiency without having to worry about the extra expense of large amounts of thermal insulation.
However, isothermal compression and decompression requires good heat exchange in the compression chamber. But finned cylinder heads and pistons meshing together should allow for a very short path for air to conduct the heat through. And if one keeps it running at a low 45-60 RPM, then it should do quite well. But might have to run a water loop through the piston head. (45-80 RPM is what a lot of hydroelectric generators spin at with directly coupled generators.)
Getting large amounts of power would more or less just be parallelism. Perhaps have a large crank shaft with a radial cylinder configuration. If each cylinder is 10 cm in inner diameter and has a stroke length of 10 cm (where it actually does work so actual stroke length is a bit longer), then with an average pressure of 60 bar we get 4712 J of energy, and at 60 RPM, that would be 4.7 kW per cylinder. And each generator might have 50 of them. (though, that is only 235 kW, but we should have more than 1 generator.) Or one has a bigger cylinder, at 20 cm dia and a 25 cm stroke at the same pressure we get 47 kW per cylinder.
Though, then there is the flow resistance losses, friction in the cylinder and piston rod assemblies and generator efficiency to contend with. But for generating power getting upwards of 80-90% shouldn’t be too unrealistic.
And for a facility like this we would also look at a power/capacity ratio of 1:15 or even 1:20. Both to ensure a slow pressure drop in our storage tanks as to give time for any thermal change to be conducted away, but also so the facility can provide power not just for the peak hours during 1 day, but actually even out power over multiple days. (For seasonal storage, a ratio of 1:200 might be required at the very least. Though, even in the season with least power there is usually still times of the day where there is excess energy.)
However, since starting a generator of this kind would likely take a bit of time. There is potential that one might just leave it running continuously. And then through electronic valves regulate if it is a motor or a compressor. Or just “free running” acting as a rotating mass to stabilize the grid a bit. Would also remove the time needed to synchronize it to the grid, as well as make it more responsive in handling load transients. So there is advantages to just having it spinning 24/7.
But the mechanical components of it and maintenance required likely only makes it economically viable at a large scale. For smaller grid storage installations, I think properly treated lead acid batteries is a better alternative, since they last more or less forever if cell balanced and if they never see an over discharge, and they love being kept fully charged and ready and have similar self discharge to lithium batteries. Peak power is a bit lower, but for grid storage we typically want more than 10-20 minutes of operation.
CAES I think probably will end up scaling down economically better than you think – as if it becomes remotely popular (which with zero self discharge (at least potentially) and simple rugged nature of it I think it will) then there will be economies of scale in producing the small CAES battery kicking in to make even quite small CAES economically viable – probably even HGV trailer sized so they can be shipped in and set up for temporary needs size small scale too.
Of course if everyone’s existing love of Lithium battery continues that means research so that somebody may well find an easy way to extract it (and the rarer minerals in the power circuits) from sea water or something similar, in which case batteries are probably the way it goes at smaller scale.
I think the mechanical nature, the still somewhat low power density. And the fact that it deals with highly pressurized tanks is the main detracting qualities for small installations.
I can however see it as useful at capacities in the low MWh region, but something fitting on a trailer I suspect might be a bit unpractical. Though. I can see a balloon approach, where one has a truck with the mechanics, and then just roll out a large high pressure tube. Throw some straps over it to anchor it and then just pump it up.
But portable energy storage of this kind seems a bit odd, I can’t think of any application where one would need it… I think batteries are frankly more manageable at the sub 1 MWh capacities.
A small datacenter however might be a different story, since they can get a call to go off grid every now and then. Likely place the tank bellow the datacenter and then use that to run off grid during peak hours. Might be more economical long term than diesel generators for that application. But I still suspect a pair of diesel generators to be on site regardless as a backup. CASE wouldn’t last that long in a lengthy power outage, but riding off grid for a couple of hours a day it is good enough.
Depending on what you need it for don’t need a balloon, can just put the internal pressure up higher, even liquefied to increase the energy density – sometimes staying in the isothermal efficient range isn’t the highest priority, just getting power out and you can still get very good efficiency out of such a tank if drawn slowly enough…
But really the advantage I see over batteries is that at small scale you can still just rock up with a new prefilled tank if and when you need to – making it that hybrid of UPS and onsite generator – can’t really do that with battery as a trailer of battery costs a fortune, a steel pressure vessel costs basically nothing… Which means you probably can do without the diesel generator, which is going to be great for things like festival where the noise and fumes of the generators are a problem (CAES being usually a turbine for power output should be basically silent if its balanced right), and potentially very useful in place of diesel where you need backup power but either can’t risk a spark, legally aren’t allowed to operate ICE in that environment or just want to be able to virtue signal how green your emergency system is (as for any longer term problem shipping in more compressed air really isn’t going to be greener than burning stuff unless the transport and compression can be handled entirely from excess renewable)..
Yes, the key word for CASE is “slow” at least as far as the isothermal approach goes.
Question though is how much energy one can realistically place on a truck.
If we have for an example a 12 meter long tank that is 2.4 meters internal diameter, and bringing it up to 160 bar, it stores 1.224 MWh.
If we discharge this at a modest 0.25 C, then we might get reasonable discharge efficiency. Lets say hypothetically that we get 70%. (generators and all included) That means we are getting 214 kW, a not that bad amount of power.
Though, a slower discharge would be more efficient.
But trucking energy in this fashion isn’t that impressive when 1 kg of biogas contains 15 kWh of energy, all though most of this energy would turn to heat in an internal combustion engine. Since these are typically about 35-40% efficient these days. So it only gives about 5,6 kWh/kg of biogas.
But we can compress biogas to 125 bar and store 100 kg per cubic meter, so there we would have for the same tank 30.4 MWh of energy when accounting for ICE efficiency.
All though, it is impressive that compressed air can reach 1/30th the energy density with only 28% higher pressure.
All though, one can convert the biogas to ethane without major energy loss (and some of the energy loss is due to byproducts being produced in the form of longer hydrocarbons.), and ethane only needs to be compressed to 42 bar for it to be liquid at room temperature. Giving us 360 kg/m^3 of a fuel containing 13,89 kWh/kg. And that is a much higher energy density that doesn’t result in too much losses along the way.
Methane and ethane also burns very cleanly as well, unlike diesel. And can be captured from a variety of sources that society will have for the foreseeable future, mainly sewage treatment and composting facilities as well as existing landfills. (a lot of these facilities either just release it into the atmosphere, or work on reducing the production, or just burn it. So why not actually use it.)
CAES isn’t always slow – that is just a choice you make when you decided what was important to you for this system, its also one of the best options for massive bursts of power at lower efficiency.
Also I never said trucking in power like that was particularly efficient – I just said it was possible! Though it also doesn’t have to be particularly inefficient either – as you rather neatly worked through one variation of – but now consider shipping it as liquefied air and just letting the new tank refill the inbuilt one at its own pace (as in no active pump or heating just let the environment do the work) – greater energy density still, though how big it needs to be to keep up with the required discharge rate is another question there that will be rather heavily influenced no doubt by the climate it operates in).
But really POSSIBLE is in many cases is good enough, when you really must bring in the backup generator ‘fuel’ it is largely a hang the costs moment we need it NOW! And your ethane etc methods are ONLY generators, they MUST have external fuel, where the CAES system (at least can be) acting as self charging UPS/grid leveling battery for those shorter outages and cheaper power rates…
I do agree though in many cases the gas powered generator has more merits, but like all these things its the engineering and operational compromises – with anything burning fuel you have to ask questions like does it go bad in storage, is it safe and legal to burn anything in this environment, can it be spooled up quick enough, how long will it take to get more fuel, how reliably can we source the fuel etc… So even though its a good choice for many situations there will be times, probably many of them when it isn’t the right choice.
As a medium to long term UPS, CASE makes a good case for itself, especially in environments where exhaust fumes are undesirable. It has moderately high energy density, isn’t really any more technically involved than a typical generator, might be a bit larger for efficiency reasons. And the ability to charge it is a major advantage.
For emergency power, it might work. But in most emergencies any power source is usually accepted.
In regards to long term storage of fuels, I have long wondered why diesel managed to take the lead, it has a shelf life of only a few months (unless one adds poisons to it to stop the growth of sludge). Methane and ethane are more or less non perishable, at least on the human timescale. And methane is easily sourced. Downside is one needs a bit bigger tank compared to diesel, and it is under pressure. (but the pressure practically means a fuel pump is redundant.)
However long distance transport of energy, compressed air, even liquefied might not be ideal due to the low energy density.
Localized temporary energy storage for evening out demand during events, festivals and concerts is a novel idea. One that might be very economically viable.
Though, where I personally think CASE is most applicable long term is:
1. Larger installations near major substations. (500-40 000 MWh)
2. Small installations at smaller substations. (25-800 MWh)
3. Long term UPS applications at hospitals, DCs, etc. (2-200+ MWh (for critical applications a generator is still recommended.))
4. Demanded semi/full “off gridding” of factories, DCs, transmission towers, and other major energy users with on site generators during peak demand hours. (capacity varies on application.)
Another thing already discussed earlier is the advantage of freewheeling the CASE motor/generator as to form a synchronous capacitor that can aid with phase balancing and handling short term transients while also effectively removing start up time, making it an even better UPS or grid balancer.
I agree entirely the economic case for the situations you postulate are very clearly good fits for CAES. And larger plants are great because the bigger the plant the greater range of output power it can produce in so it is both useful and in the isothermal efficient range.
The other suggestions I’ve made are very prevalent on the tech becoming mass produced in the smaller UPS type scale, which might happen to get power around clean air zones (etc) – enough that the smaller units are just available – at which point you can economically use it for a great many more marginal options as the biggest cost with CAES is building it initially, running costs and efficiency are usually positives for the tech, and its large volume to comparable energy storage isn’t always much of an issue.
Thermal storage is a great one anywhere there’s significant demand for HVAC or hot water.
Using ice for air conditioning gives about the same cooling per pound as using lithium batteries to run a conventional air conditioner, but water is orders of magnitude cheaper and has no inherent limit on lifespan.
https://www.youtube.com/watch?v=aFnSFWx13mo
>(Lead-acid) Then they last a good few thousand charge cycles
No they don’t. It’s a matter of how you count a “charge cycle”. It’s only above a thousand if you never fall below 10% DoD, but that also means your battery capacity is effectively just 10% of what you bought.
The main failure mode of lead acid batteries is due to excessive buildup of lead sulfide during discharge.
Since the lead sulfide takes up more space than the lead oxide it exerts a force along the plane, when this force becomes sufficiently large, the adhesion of the lead sulfide is insufficient to hold it in place and it starts flaking off.
This flaking only starts to happen bellow about 1.85 volts. Ie, stay above this voltage and the lead sulfide never flakes off and will continue to contribute to the cell capacity.
While go bellow 1.85 volts and the flaking generally is going to start to happen, exactly when depends on contaminates and such, but bellow 1.80 volts it is more or less guaranteed.
Secondly, look at the discharge curve for a lead acid cell.
The majority of the energy is between 2.1 to 1.85 volts. Far a measly 10%.
In short, discharging a lead acid cell only down to 1.85 volts will see it give out about 85-90% of its “rated capacity”, while not being subject to any flaking. Occasional topping up of battery acid will however be needed every couple of years.
Another failure mode is excessive overcharge where it boils off the electrolyte by splitting water. But with cell monitor this can also be avoided. Preferably cell balancing should be used to ensure a more even discharge of the bank, and a more even charge. Even if one can just overcharge to balance the cells, but a proper cell balancer is generally more efficient.
Though, overcharging lead acid cells isn’t that devastating, as long as one doesn’t noticeably discharge it. If it doesn’t have enough electrolyte, the whole cell capacity won’t be available and one risks discharging it too fast and excessive heat buildup is then a concern.
In reality, discharging a lead acid battery to 85-90% DoD as you suggest would destroy it in a few hundred cycles. Never would it go into the thousands; I don’t know where you’re getting your info.
On a 12 Volt car battery going down to 85-90% is indeed likely only going to last a few hundred cycles before the smallest cell dies and makes the whole pack unusable. Especially since the cell to cell manufacturing tolerance is a good few %.
But I didn’t specify 6 cells in series with no cell balancing nor voltage monitoring.
There is a huge difference in what a car battery can offer and a set of individual lead acid cells being treated properly can offer.
With only 2 Volts per cell, using them individually or in large parallel banks as such is rather difficult, and I still find the claim dubious to say the least.
One uses a somewhat thick copper strap, and preferably have enough cells in series to not need to pull tons of current to get a decent amount of power. The straps won’t have to be all that long either since the cells usually aren’t gigantic.
But treating the cells properly does a huge difference.
Now most people only have experience with lousy car batteries that could as well be described as torture chambers. However, lead acid batteries don’t express their displeasure as violently as lithium ion batteries tends to do, so the industry hasn’t had much incentive towards treating them properly unlike lithium batteries.
To be fair, even a lot of installations that have individual lead acid cells still rarely use cell balancing. Usually due to the fact that one can just overcharge the cells to balance them out at the expense of boiling electrolyte. Here the principal is more of “don’t discharge too deeply.” And if a cell dies, one replaces the individual cell. But with active balancing and cell monitoring one can treat the bank even better and get out a larger amount of the energy without going down low enough for lead sulfide to flake off.
Is lead acid “the best battery”?
That depends, for mobile applications it is abhorrent due to its weight. For stationary UPS applications it is perfect.
If treated properly, the charger and inverter might break first, or the cell monitoring/balancing, or the case surrounding the lead plates might crack after 30+ years of service. And of course the acid needs to be topped up every couple of years.
Personally I wish that single lead acid cells weren’t a specialty industrial/military grade item carrying a somewhat hefty price premium unless one buys 10+ of them or a really really large one.
Interesting article – from what I know of the UK grid it doesn’t seem like it would be such an economic challenge to implement as it is in Korea apparently (not an expert though, just somebody who has a grid connected battery and home solar array).
I think the reality with any cost benefit type analysis of V2G type systems is you can’t know how things will change in the rather short term as it rolls out well enough to predict*, but there is one certainty – its alot cheaper and quicker for the governments and power companies to get the public to pay for and provide a lot of grid leveling storage as part of their own transit arrangements than store the same amount in bespoke purpose built facilties.
*as the coal/gas/oil gets shut down the cost/supply/demand all become very different to any historical data you can study, so its going to be really guesswork until somewhere rolls it out and finds a way to make it function (even if its with subsidies – as then you have a useful study of how the power grids can work when they are as decarbonised as they are going to become, and know what they were like only a short time before as a comparison).
Yes, very much agree. In the situation where a person has a solar system on their house v2g makes a lot of sense. In Australia we have very cheap roof top solar, some of cheapest in the world. it’s so cheap than even if power was free (commercial price does go to zero and below on days with excess solar and low demand) the cost of transmission is more the the cost of rooftop solar. about 30% of people have solar and many people now are installing batteries. Also as consumer we can now get access to the commercial power price (plus transmission cost) this price varies with demand and supply throughout the day and is updated every 5 minutes.
This makes battery storage potentially lucrative but also very necessary to manage our high solar supply. Instead of paying $15k for a 10kWh Tesla power wall using the 50+ kWh battery in the car you already own makes so much sense. The issues identified in the article are fairly trivial to solve and most in fact have already been solved.
Yes using your car for grid arbitage alone may not work in every market, and will depend on how the car is used, but when paired with roof top solar it can only ever be a win.
Yes, but how’s your house going to work when the car is not there? Or when it hasn’t had a good charge lately?
The problem with the transmission costs is that the cost is fixed, independent of use and dependent on local and total transmission capacity, so the more people go solar the higher the price per unit transmitted is going to be because the surplus needs to be transmitted and the electricity will only be paid by those who aren’t producing at the moment. In other words, the average power price isn’t coming down because of solar – it’s just swinging up and down a lot more.
The house is still on the grid when the cars not there. The car is helping manage the solar. I a system with a lot of solar and storage, it wont matter too much if my cars not home as there will be others available to soak that peak generation and discharge at peak demand.
As too solar purchasing up prices, the evidence clearly shows otherwise. Here in Australia the state with the most renewables SA (they regularly hit > 100% of demand generated by renewables), has by far the cheapest power in the country. Yes it can and is causing swings in price, further inflated by coal plants not being able to deal with large price differences throughout the day, but firming is becoming much cheaper. In the Australian market firmed solar/ wind is cheaper than both coal and gas.
Adding more distributed storage in the form of ev v2g batteries only helps to futher bring down this price.
source on prices: https://opennem.org.au
> it wont matter too much if my cars not home as there will be others
There’s the unfortunate habit of most people who drive cars to not be at home during workdays, and they tend to take their cars with them.
It creates a curious pattern where most V2G connected cars would charge up while their owners work, at the workplace with electricity generated at home, and then they drive home in the afternoon and discharge the electricity for consumption, adding a completely unnecessary and a wasteful transmission step in the middle. Should the battery stay at home, you wouldn’t need the grid in the middle.
There are technology changes in the medium to long term. Straubel’s comments from 2016 sound a bit off to my 2022 ear, most of it was about battery degradation but lithium iron phosphate has changed the lifespan of batteries and I think a lot of cars up to 50 or 60kwh will switch over to them, so where does that leave v2g? At what point is it profitable?
Checking octopus energy’s Agile export tariff the average over the last week is something like 30 or 35p per kwh which I bet they didn’t see coming. A 50kwh car good for 2000 charge cycles nets you £30k at that point so it’s gotta be close to profitability.
Also not just tech changes the price for energy is changing enough that a less than optimal battery for heavy grid connected use actually could end up be being economically a no brainier, and better than having a great for grid battery and an EV battery used strictly for their designed goals – that costs more upfront etc..
> lithium iron phosphate has changed the lifespan of batteries
There’s plenty of types that have better lifespan that that, but LiFePO4 and the others come with other compromises, chiefly price per kWh. They’re less than economical.
> I think a lot of cars up to 50 or 60kwh will switch over to them
Unlikely, since the energy density of LiFePO4 is relatively poor. It’s half that of NMC/NCA, so you get batteries that weigh twice as much. It kinda-sorta works in a large sedan aimed for the luxury market, but the capacity is too small for those, and it’s too heavy for subcompacts and hatchbacks so the capacity would be less.
For stationary use weight is a non issue and cycle life is everything.
Though NMC and MCA battery have a lot of pollution involved in their manufacturing from the cobalt.
One reason I feel plugin hybrids are better you can get by with a smaller 30kwh battery and have enough range for most short trips and have an ICE for when you need to go far.
LiFePO4 are perfectly fine for hybrids even ultra batteries basically a hybrid of the lead acid battery and ultra capacitors work fine in them.
In fact in something simulation dealing with the duck curve in solar UltraBatteries lasted through enough cycles to turn a Tesla battery into scrap a few times.
Who says you can’t teach an old dog new tricks.
https://en.wikipedia.org/wiki/UltraBattery
The only niggle about LiFEPO4 batteries is that they really don’t like to accept charge below 0 C which is also the reason why they haven’t picked up as lead-acid battery replacement in cars. It takes a heck of a long time to heat them up before the charger will actually charge it and you end up running the battery flat in normal day-to-day commuting in the winter.
The benefits/savings from V2G come from not having to size the expensive parts the grid to the peak demand.
Except EV’s may very well not be where you want them or in a useful state of charge during peak demand.
Everyone wakes up in the morning, they run heat & light & hot water to get ready then they jump in their EV to commute to work – pulling a load of charge out of your EV just before you want to use it is not ideal.
Then during the day, everyone’s at work, so unless their works car park has a V2G charger per employee the EV is sat there doing nothing.
Then everyone drives home in their EV which now probably has less charge, uses light & heat & cooking which pulls even more charge from the battery.
Sure you can recharge to 100% overnight when there’s low demand, but stressing the (expesnsive and finite-lifespan) EV battery twice per day to even the grid load feels sub-optimum
EV batteries are expensive and delicate compared to other grid storage solutions, even other battery technologies that are more robust & cheaper but heavier & less dense, it feels like we could be killing lifespan of a relatively expensive resource (and massively complicating our grid) rather than install a far better alternative in some more scalable bulk storage system.
Alternatively as an extreme example – what if you work in a smelting plant and they generously decide to provide V2G for every car in the car park to smooth their peak load, so everyone comes out at home time to find their EV has been run nearly flat to cut the top off the peak load?
Yes and no – even if you take your situation to be what 100% of the EV’s do most commutes are short, very very short in many cases – so the EV that can do 200, 300, 400 miles can easily dump 50% of its charge every morning, probably even more than that (not that it would) and still have way more than the user actually needs that day. And as many more EV are connected the amount that will be needed out of any one of them will drop ever, quite possible reaching the point when the user wouldn’t even know they were not at full charge in the morning, as effectively everyone is just running their own morning peak load – which won’t even be close 1Kwh for most I would think – the spikes drawn will easily hit 2 maybe 3 Kw, but the duration is damn low, only takes minutes to put the kettle on, make toast etc, that isn’t enough energy to very far, Cars are heavy…
To meet the peak demand it would probably not take much of anything out of the EV batteries – I don’t think that 1% would an unreasonable number to assume. You’re just filling in the gaps that the grid can’t meet, not running an entire country. The more EVs in use the better the situation becomes.
As for stressing the batteries, this can be alleviated somewhat by not charging/discharging them as strenuously. Slow charge overnight by default being a easy example. This is a luxury that not everyone will have; being able to charge at home or at work. But the majority of EVs will likely charge this way.
The whole battery lifecycle has been cited as a risk, both by manufactures with their conservative life estimates, and by EV detractors. The reality has been that a properly managed battery (read: temperature maintained) has excellent life. And that is only going to get better as quality and technology improves (capacity increases will not be very dramatic though)
JohnU I think you have unintentionally found a USP.
Most of us don’t work in smelting plants.
If employers provided free EV charging points to all employees they could use the plugged in cars to do load balancing during the day, and then around an hour before going home time make sure all cars are topped up.
Then the employees drive home and can use any excess energy garnered to heat, light and watch the TV, surf Hackaday, etc, both in the evening and the next morning (if it’s a work day).
How about a revse power system,where the main house breaker
when thrown,uncovers a socket for conecting a reverse power cable.Good for permanent off grid and power outage situations.
It needs human management,but there is no scenario where useing car batteries for grid back up,unless someone creates an
allpowerfull acronym that robotisizes people.
Going to agree V2G is a daft idea as the losses would be high and it would drastically shorten the life of EV batteries which is already a serious issue.
Then there’s the issue vehicle batteries and grid batteries have very different use cases even old school iron nickle batteries are better suited for grid storage than most EV battery chemistry.