This month the media was abuzz with the announcement that the US National Ignition Facility (NIF) had accomplished a significant breakthrough in the quest to achieve commercial nuclear fusion. Specifically, the announcement was that a net fusion energy gain (Q) had been measured of about 1.5: for an input of 2.05 MJ, 3.15 MJ was produced.
What was remarkable about this event compared to last year’s 1.3 MJ production is that it demonstrates an optimized firing routine for the NIF’s lasers, and that changes to how the Hohlraum – containing the deuterium-tritium (D-T) fuel – is targeted result in more effective compression. Within this Hohlraum, X-rays are produced that serve to compress the fuel. With enough pressure, the Coulomb barrier that generally keeps nuclei from getting near each other can be overcome, and that’s fusion.
Based on the preliminary results, it would appear that a few percent of the D-T fuel did undergo fusion. So then the next question: does this really mean that we’re any closer to having commercial fusion reactors churning out plentiful of power?
Science Communication
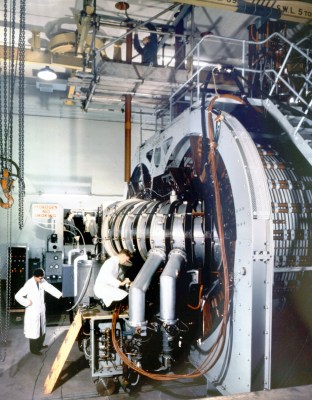
As the eternal jib goes, nuclear fusion is always a decade away, ever since its discovery a hundred years ago. What is sadly missing in a lot of the communication when it comes to fundamental physics research and development is often the deeper understanding of what is happening, and what any reported findings mean. Since we’re dealing with fundamental physics and boldly heading into new areas of plasma physics, high-temperature superconducting magnets, as well as exciting new fields in material research, all we can do is provide a solid educated guess.
With the Z-pinch fusion reactors of the 1950s, it seemed that commercial fusion reactors were a even just a few years away. Simply pulse high currents through the plasma to induce fusion, harvest the energy and suddenly the much touted nuclear fission reactors of the time already seemed like relics of yesteryear. With initially high Q numbers reported for Z-pinch fusion reactors, the newspapers ran the headlines in the absolute certainty that the UK would be building the first fusion reactors, with the rest of the world to follow.
Later it was discovered that the measurements had been off, and that neither the assumed fusion gain had been as astounding as reported, nor had anyone been aware of the seriousness of plasma instabilities in this type of reactor that complicated their use. It wasn’t until the Russian tokamak design, which added an electromagnetic field around the plasma, that it seemed that these plasma dynamics could now be dealt with.
Although an alternate solution existed at the time in the form of stellerators, these require a rather complex geometry that follows the plasma field, rather than constricting it. This meant that they didn’t become attractive until the 1990s when computer simulation power was good enough to model the requisite form of such a reactor. Currently, the Wendelstein 7-X (W7-X) stellerator is the largest and most interesting implementation of such a reactor, which has recently been fully configured with cooled diverters that should allow it to run continuously.
All of which is to say that since the 1950s a lot has happened, many theories were tried, some things stuck, while others flopped. It’s on this wobbly edge between the fields of practical and theoretical physics, as well as materials sciences and various engineering disciplines that humanity is moving ever closer to making a practical commercial fusion reactor work.
Inertial Confinement Fusion Is A Laggard
The NIF at Lawrence Livermore National Laboratories (LLNL) uses laser-based inertial confinement fusion (ICF) which essentially means that the D-T fuel is held in place while it gets blasted in order to achieve fusion. At its core this isn’t significantly more complicated than other fusion reactor concepts, all of which tend to use D-T fuel in the following reaction:
As part of the fusing of the two hydrogen nuclei, a significant amount of energy is released, which can be captured in order to create steam and drive a generator. Meanwhile the helium waste has to be removed, the high-velocity (fast) neutrons captured, and the D-T fuel replenished. When comparing this to magnetic confinement fusion (MCF) technologies such as tokamaks and stellerators, it becomes clear why ICF isn’t even in the same league.
Both tokamaks and stellerators are essentially designed as continuous use reactors, with a constantly maintained plasma flow in which deuterium and tritium nuclei fuse and contaminants are removed via the cooled divertors. Neutrons are captured by a lithium blanket that lines the inside of the reactor vessel, which causes tritium to be bred, allowing for this short-lived hydrogen isotope to be constantly replenished along with deuterium.
Ultimately, a tokamak or stellerator would be self-heating, in that they have a Q of over 15. This means that the reactor can supply the energy needed to heat its plasma, while still producing enough energy to run the generator, or similar. This would make such a reactor essentially self-sustaining, none of which applies to an ICF system like the NIF. It requires the production of its special D-T fuel pellets, and insertion of each pellet into the ignition chamber. This makes continuous operation rather cumbersome.
In terms of net energy production, the NIF also doesn’t look very good. Whereas for example the UK’s JET tokamak has reached a Q of about 0.65 (below break-even), when the approximately 422 MJ input power for an NIF shot is taken into account, the produced 3.15 MJ is paltry, indeed.
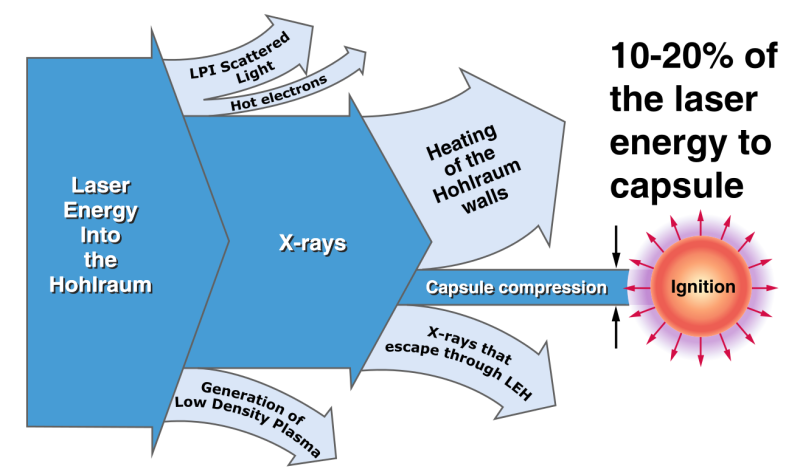
Putting A Price Tag On Research
During the Cold War era, the R&D budget for nuclear fusion research was rather significant, at least partially enabled by the lingering fear that possibly the Other Side might manage to tame this incredible new source of power first, also helped by the fascinating insights gained in how thermonuclear weapons might be tweaked and maintained more optimally.
When the Cold War ended and the 1990s rolled around, nuclear fusion research found its R&D budget hollowed out to the point where most of it ground to a screeching halt. These days nuclear fusion research is doing significantly better, with many nations running MCF research programs. The majority of these are tokamaks, followed by stellerators with the Ukrainian Uragan-2M and German Wendelstein 7-X being prominent examples. The rest are ICF devices, which are notably used for fundamental research on fusion, not energy production.
In this context, if we look at the NIF’s 3.15 MJ, it should be clear that we have not suddenly entered the age of commercial nuclear fusion reactors, nor that we’re on the verge of one. What it does mean, however, is that this particular ICF facility has achieved something of note, namely limited fusion ignition. In how far this will be helpful in getting us closer to commercial fusion reactors should become clear over the coming years.
What is beyond question is that putting a price tag on fundamental research makes little sense. The goal of such research and potential is after all to increase our understanding of the world around us, and to make life easier for everyone based on this improved understanding. Considering the wide range of responses to these recent NIF findings it does raise the question in how far the fundamentals of nuclear fusion research are at all being communicated with the general public.
Only three decades left!
Fusion has been 3 decades away ever since they started working on this and will continue to always be 3 decades away.
Yeah, they predicted you’d say that.
You’re aware that we *are* making progress on fusion power, right? That we actually are closer to practical fusion power than we were in the past? People who make that crack seem to think that we’re fumbling around in the dark on something that’s impossible. We’re not. It’s true that we’re discovering that it’s more difficult and more complicated than we thought, yes. But it’s also true that we’re steadily discovering the complications and learning how to work around them.
We’re learning as we go. We’re getting better at it. We’re getting closer with every round of experiments, and the fact that people have been overly optimistic about it in the past doesn’t change that. People being overly optimistic about it NOW doesn’t change that.
Sooner or later, we’re going to work out the last problem that stands between us and useful fusion power. Yeah, we’ve been wrong about how close the finish line is in the past, but we ARE moving forward, and as long as we continue to do so, we’ll eventually cross that finish line, no matter how far away it is.
While it is a worthwhile endeavor regardless how long it takes, I think we can all agree this is not a breakthrough that we will remember in history books. Fusion technology is barely a notch further in progress than it was before this achievement. If we want to be honest here, Its more than likely no less than fifty years out and that is being optimistic.
With the knowledge of x-ray field containment to overcome the Coulomb barrier comes mechanical structural invention to create potentiometerical control. A pyramid shield with four point ejection configuration would provide zone of stability at the center, would it not? I’d love to see. Progress comes from diligent experimentation. Bravo on Q of 1.5, Bravo!
Well said, sir.
Can you measure the amount “closer”? Is it 1%? 60%? I don’t think it is possible to know except in retrospect. We won’t know until some mile stone is achieved that can me used as a metric. Fusion has always been a guaranteed full career research job. I’m pretty sure I will be long dead before anything useful happens.
Maybe an Elon Musk of Fusion will come along and quit building gigantic research projects and work on the engineering. By the way, the research versus engineering focus was the complaint by many scientists 40 to 50 years ago. See Riggitron – Robert Bussard.
A metric mile stone would be indeed a foot in the door of the SI units :o)
Exactly! A foot in the mouth is worth two in the door.
I won’t be around to see the day it happens. If I were a betting man, I’d bet on another 100 years before fusion becomes a thing that can be used for any viable power generation. And by *any* I don’t mean a lot of it.
Its not the science, its the engineering. Fusion will likely be a defining hallmark of the 22nd century. The engineering needed is mechanical, and high energy physics related. Unfortuanatley, those things do NOT advance exponentially like microprocessors and computer relatated tech via Moore’s Law. Computer modeling helps refine existing fusion modeling, but won’t likely lead to big breakthrough events. Big breakthroughs require out of the box thinking; perhaps merging or using hybrid fusion methods into one design, and similar. It’s not impossible, but it will take a number of successive breakthroughs over time.
So 2 MJ of energy left the lasers, 10-20% of that impacted the capsule, and 3 MJ of fusion energy was released. Doesn’t sound so bad until they tell you that the energy going INTO the LASER was in the neighborhood of 300 MJ. So the Q is 3/300, not 3/2. I want to know what the theoretical maximum (approximately) efficiency of the MJ into the laser, can be expected to ever be delivered to the fuel pellet, what the maximum expected efficiency of the laser to capsule is. If the electrical generation is achieved via heat/steam/turbine, we might assume that near 50% efficiency conversion of the fusion reaction to MWs produced. How much higher might the direct magnetic field generation be? Surely someone can give us some ballpark numbers. My cynical self says the obfuscation if the real target. Prove me wrong. Sell me with facts.
The latest I read is more like 1/400 for these experiments. OTOH they are just experiments to look at the contitions that can produce some fusion. I wonder how long it takes to charge up for a shot?
The people who are smashing hypervelocity pellets might have a better game.
I worked on NIF’s assembly then later on targets. It’s remarkable they achieved some measure of fusion. Congratulations. I agree the tokamak is a better path. Coincidentally I’m now assembling ITER. Let’s all be honest as far as we know we have a finite amount of fossil fuels. So we [mankind] must search for new sources of energy. The bonus is that along the way we discover new knowledge for other applications and thats a good thing. The question is will it be in time or too late for the next generations that will follow. Quite the pickel.
We know how to achieve fusion for decades now. Ever heard about so called “hydrogen bomb”? It’s sustaining it for longer time is the problem. NIF is just more fancy way of starting fusion with no guarantee of the success of it as a method of sustaining fusion for long. TBH no one can guarantee it even has sense to burn this much cash for decades now. There is even possibility that stuff achieved in the process would be achieved anyway as side effect of construction of stuff that is actually useful both in practical and commercial terms. It’s all moving in the multidimensional space of probabilities without ANY guarantees.
laser based fusion offers sub integer improvements every few years. if you need a couple orders of magnitude (q>100) more performance for the thermodynamic backend, then at the current rate of progress, then we will have fusion in a few hundred years. the time table for this kind of fusion is even longer than with tokamaks.
the iter route is looking at 2 (gigantic) tokamaks minimum before a power plant can even be built. all for a result that is less economical than fission. they at least tend to go up by factors of 10. the 20 years cliché is pretty much the amount of time it takes to fund, design, build and do science on the next tokamak and get to a point where you need to scale up. so were looking at a 60 year (optimistic) timetable before we get a single watt to the power grid.
fusion is a long term goal, its not going to be a get out of climate change free card that people think it is. we might see a few breakthroughs along the way, maybe a fringe fusion concept can progress faster than the glacial iter project. especially reactors which are smaller and lend themselves to faster iteration (granted they have to be viable first). needless to say i think we should be building fission plants now as a stop gap solution, along side renewables. keep plants we got online, and keep working on the storage problem to get better use of intermittent renewables.
Fission plants and code. Both are about putting the “stop gap” into production till one creates something better.
You need fission anyway to create enough tritium to bootstrap the fusion reaction. Since tritium half life is around 10 years, we can’t store it until a fusion reactor is ready to use it, it needs to be produced continuously for feeding experiments and exit emergency lights.
“What it does mean, however, is that this particular ICF facility has achieved something of note, namely limited fusion ignition.”
I don’t know why reporting on this is constantly missing the important note here: NIF is *not trying* to make commercial fusion. That’s not the point. At all. They know this will never be a method for running a fusion reactor. No one believes it will be.
Again, to stress: everyone knows inertial confinement fusion can’t be a reactor design.
The entire point of this is to study a burning plasma, because we have *no experience* with it. There’s already evidence that it didn’t exactly behave as expected. Burning plasmas are *complicated* because you’re dealing with the far tail of the velocity spectrum – you don’t need all of the atoms to have enough energy to fuse, just a tiny fraction of them.
It’s plasma physics. It’s plasma physics you can’t do anywhere else, especially considering we, uh, don’t exactly have enough tritium to run in a large reactor for any significant amount of time.
I saw several news report talking about the ignition and I thought it was a bit fishy, so I found the actual announcement by the researchers. https://www.youtube.com/watch?v=Cmzep3YaRNI. At about the 3:40 mark he is talking about what this research is all about. He says, “we studied nuclear ignition to keep our nuclear deterrent safe, secure, effective and reliable, and to do so without the need for further underground nuclear weapons testing. Fusion ignition is a key process in our thermonuclear weapons.” He goes on to say that it will help maintain and modernize our nuclear deterrent. Then like it was kind of an afterthought, he talks about how someday fusion could provide carbon-free source of power. I’m with you Pat, this was never designed to produce usable power. It can’t be sustained(only one shot per day), uses expensive unobtanium fuel, 300MJ to create 3MJ. To me it seems like this is nuclear arms research designed to skirt the current testing bans, not for the production of energy.
The media difficulty comes because, back in the 70s, you did have people who thought that ICF would work. Like, prominent physicists, not like, wacko people. In fact, it basically started as “hey, what if we continually explode bombs to generate electricity via steam” and then after people realized that would be, uh, bad, it was “… what if they are *very small* bombs.” But eventually it became clear that the simulations used to justify those arguments were way, way wrong. Even by the 90s it was obvious that ICF was a non-starter.
But obviously there are other important points of understanding the burning plasma regime, and so that’s what NIF eventually morphed completely into. There’s definitely more merit to the nuclear weapons research, but obviously burning plasma research will help fusion power production too.
This is exactly why it had billions thrown at it. It lets you validate the simulations of bomb secondary stages.
Thermal conversion of heat to electricity is just engineering, and well understood engineering at that. So assume 20% efficiency to convert the heat to electricity. Maybe slightly pessimistic, but good enough for a BOTE calculation. (Yes, I am ignoring MHD conversion techniques.) Based on the statements of the lab director, “wall socket to target” efficiency is about 0.7% So we need roughly 3 orders of magnitude increase in efficiency to reach engineering break even. That is, conceptually the unit could power itself. Two to three orders of magnitude increase for economic break even? Something like that. Now add in the duty cycle of the existing lasers which is minutes (hours?) per pulse. All that waste heat must go somewhere.
So as “Pat” has said, this is excellent physics, and useful for the National Stewardship Program, but not much else. Sadly, I have already heard one talking head saying this is proof we should go to EVs because power for them is right around the corner.
To be clear, research like this is critical to fusion designs that *can* generate net positive energy, because you can study burning plasmas in small scale. The fact that it’s not a reactor design is a bit of a silly complaint: *no* fusion research facility is actually a reactor design anyway. JET, W7-X, etc. have no mechanism to inject fuel, for instance. ITER isn’t a reactor design, they have no mechanism for large-scale tritium breeding (that’s mostly what it’s being built *for*).
All the various fusion facilities (NIF, ITER, W7-X, JET, etc.) are studying different aspects of creating a working commercial fusion reactor. They’re all important. They’re not competing with each other.
The Helion is another interesting new design for a fusion reactor using pulsed magnetic fields to compress a plasma and subsequently to extract energy from the reaction: https://youtu.be/_bDXXWQxK38
I saw this the other day. It’s an interesting concept that converts the plasma pressure against the magnetic field to generate electricity, bypassing the need to convert thermal energy to steam, and steam to kinetic energy to move the generator.
Direct extraction/conversion of electricity would be much more efficient than the equivalent of replacing the coal grate of a steam engine with a nuclear fusion heater.
Smashing donuts. Seems Star Trek might have been on about with the pulsing animation feeding the power chamber.
I remember reading about this design back in the early 90’s. Essentially two ion beams or ion clouds pointed at each other, magnetically focused, and the oscillation of the ions between the two ends can be used to generate electricity through induction. The issue has always been the energy lost due to non-charged particles (neutrons) and photons (from bremsstrahlung radiation). Interesting design though. I always wanted to build one.
The recent successful work has a direct application for fusion energy production because it is informative in the areas of laser control (pulse shaping) and target structure/quality, even though it was using 1990’s era lasers which have had their efficiency greatly exceeded by more recent technologies. The Australian company HB11 Energy is developing Laser Hydrogen Boron-11 fusion to generate electricity using *laser-ignited non-thermal fusion.* It is an aneutronic and very compact path to small and affordable reactors that do not produce any radioactivity at all, nor does it rely on thermal engines to drive generators, the *electric charge is generated directly.* And if you are thinking pfffft what could Australia do, take note that the most advanced uranium isotope separation technology in the world uses lasers and was also developed in Australia.
Interesting, thank you.
The only fusion power sources we know of rely on gravitational confinement, something we cannot reproduce on Earth. That is, stars, which confine the plasma by their large mass.
Maybe we will find another way; maybe not.
And this is really not efficient at all. A cubic meter of the core of the sun is only producing ~200W, not much more than your heap of compost in fact.
Since we can’t waste that much volume here on Earth, we have to rely on a much stronger pressure & temperature confinement systems. That’s why it’s hard, because matter doesn’t want to be so compressed.
“And this is really not efficient at all.”
Oh, stars can be a *ton* more volumetrically efficient than that at fusing elements with gravity. You just don’t want to be near them when they are.
Ha ! Supernovae are not the best neighbours…
IMO the discovery this year that the proton mass is a flibbertigibbert cycling back and forward between heavier and lighter fundamental particles may lead to breakthroughs in other fusion related science that has been written off as experimental errors due to the huge temperamentality in replicating it. Fusors, and other waysided “blind alleys” may gain some traction due to now being able to statistically load the dice, now that we know there are dice to be loaded.
Wouldn’t it be neat to have Philo Farnsworth’s Fusor design become the basis of functioning fusion power generation? Shortly before his death he said he was working on something new on that, but after the television patent fight with RCA he wasn’t putting anything on paper yet.
> for an input of 2.05 MJ, 3.15 MJ was produced.
So, from 0.6kWh of laser energy they produced 0.9kWh of heat. Lasers are not very effective, so they spent at least hundreds kWh of electricity to do that.
So, where is a breakthrough?
“Breakthrough” of that level could be made by every third reader here for the sum of $100 approx. (mostly for heavy water for deuterium, it’s extremely boring to make it by yourself) and some parts from garbage bin. Just don’t forget about that nasty high-energy neutrons that will began to shoot out from your fusor and use some shielding. Concrete will be fine for this.
You will obviously get Q>1 as soon as thermonuclear reaction starts. Start of reaction you could control with regular Geiger tube gamma-rays detector – you will get gamma when neutrons from fusor began to bombard your shielding.
Did I already mentioned, that you absolutely don’t need billions and dozens of years to do same “breakthrough” in your garage?
Seriously, we all heard about that “breakthroughs” since 1960s without any hint of self-sustaining proof-of-concept. For tens thrillions, if not hundreds. You will not find any other invention that gave nothing for 60 years. Too tasty grants to finish the work?
PS: And no, that will not be “clean and cheap” energy. Fusion will have the same problems as NPP – nuclear waste (fast wearing fusion chambers will be highly radioactive because of neutron radiation), expensive fuel (deuterium and especially tritium are not cheap), complex design (complex reactor and all that steam turbine stuff, just like on regular NPP) and so on. And in no way it will become personal power sources to power your household independently. It will be same corporations milking you through the energy yoke.
Break even fusion power generation was first achieved Nov. 1 1951; over 70 years ago. Part of the Bikini atoll island group was vaporized by the first man made hydrogen fusion bomb. A fission explosion input of tens of kilotons yeilded fusion power generation of several megatons. Overall yeild was over 100 megatons but about 75% of that yeild came incidentally from fission induced in the uranium tamper. Still there was more fusion energy produced than energy input to trigger the fusion.
Like recent NIF experiments the purpose of this experiment was not commercial power generation. Also like NIF there was no practical mechanism for converting the explosive fusion power into electricity.
The NIF results are an advancement and might even be considered a breakthrough in the area of nuclear stockpile stewardship. To call it a breakthrough in the effort to create commercial fusion power is just ridiculous for any number of reasons. Set aside the fact that there is no practical mechanism to convert tiny fusion explosions in a huge chamber into useable electricity and the fact that no such research is contemplated.
The hohlraum targets are expensive meticulously created items with no path to commercial viability. Even if these targets cost “only” $1000 each the 1.3 MJ they generate is about 1% of the 121 MJ in a gallon of gas. So this “revolutionary” energy source is equivalent to gas prices well in excess of $100,000 a gallon; probably closer to million dollars a gallon gas since the hohlraum targets are literally diamond and gold coated and cost more than $1000 each.
The list of problems that need to be solved for commercial fusion is long. Ceating a sustainable non-destructive fusion reaction that yeilds usable amounts of power is just the first step. A mechanism to harvest that power is needed and commercially viable fuel sources don’t exist despite wild claims that a gallon of seawater contains deuterium enough to generate power equivalent to 300 gallons of gasoline. Separating commercial quantities of dueterium from seawater is possible but not economically viable and current fusion research tends to rely on much rarer and costlier tritium as part of the fuel mix.
Some level of fusion research is certainly worthwhile. However unless aliens show up with radical fusion technology they are willing to trade us for the “extra” water we have flooding our costal regions due to global warming (instead of just mining an ice moon around Jupiter? . . . crazy aliens) commercial fusion remains a generation or more in the future. Fusion research is extremely unlikely to address any current energy issues. Putting more research “eggs” in the tried and true fission power research “basket” to make something that we know works much cheaper and more widely available has a bigger and earlier payoff.
Perhaps the aliens prefer our water because the energy needed to melt the moon ice is too expensive…
This is an interesting backgrounder. Exploring fusion is important science.
At this point, my gut feeling is that we will get to a more efficient storage solution for renewables before commercial-scale fusion is achieved… at which time, fusion would not be worth the hassle to roll out. But I won’t be around to see that, I expect.
To the points raised about better communicating science to the masses… you hope for too much. If the the anti-science cretins can’t make the right call on getting vaccinated or wearing a basic mask in a pandemic, grasping plasma physics is a pretty big leap.
We went from an Atomic Bomb in 1945, to the first nuclear reactor in 1951. Only six years to practical use of fission. Even if you go back to the start of the Manhattan Project in 1939 (eliminate the first few years which, were focused on construction of factories to generate fissile materials), we’re still only talking ~9 years. Why does practical application of Laser Fusion require three or more decades?
I’m wondering if, after making a working fusion power plant, it turns out to be more expensive to build and run than all the other technologies we have. Fission is more expensive than wind & solar now, why do they tout fusion as being limitless energy?
I would like to draw your attention to David LaPointe’s primerfusion presentation on youtube. He details another approach to the HB11 fusion reaction. Depends on the self-organizing double layer properties of plasma for containment. Also check out the Saphire Project while you are there.
I think we’ll have a big breakthrough once we find a way to make the whole thing terribly polluting and ecology-destroying.
Perhaps they should research on that basis and see what shakes loose.
How to optimize ill effects.
A physics version of ‘gain of function’ if you will :) :| :/