Most of what humankind and other mammalian species on Earth experience of the Universe is primarily restricted to the part of the electromagnetic spectrum which our optical organs can register. Despite these limitations, we have found ways over the centuries which enable us to perceive the rest of the EM spectrum, to see both what is incredibly far away, and what is incredibly small, to constantly get a little bit closer to understanding what makes the Universe into what we can observe today, and what it may look like in the future.
An essential element of this effort are space telescopes, which gaze into the depths of the Universe with no limitations imposed by the Earth’s atmosphere, or human activity. Among the many uses of space telescopes, the investigation of the expansion of the Universe is perhaps the most fascinating, as this brings us ever closer to the answers to the most fundamental questions about not only its shape, but also to its future, which may include hitherto unknown types of matter and energy.
With the recently launched Euclid space telescope, another chapter is being opened in the saga on dark energy and matter, and their nature and effects on the Universe, as well as whether they exist at all. Yet how exactly do you use a space telescope to ferret out the potential effects of dark energy?
The Dark Universe
When Albert Einstein was working out his General Relativity theory, he had assumed that the Universe would be static, and introduced a cosmological constant to the field equations that would would balance out the expansion of the Universe in the model. When a number of years later Edwin Hubble demonstrated that the Universe is in fact expanding, which led to Einstein calling the cosmological constant his ‘greatest blunder’. Yet unbeknownst to Einstein, this cosmological constant would later be revived, as the factor that drives the expansion of the universe.
In both the case of dark matter and dark energy, their presumed existence is the result of the theoretical models that we have developed over the past decades. These models need dark matter and dark energy to accord with what we see. Since the 1990s increasingly refined observations of the Universe has allowed us to validate elements of these theories, with “dark matter” and “dark energy” essentially acting as placeholders until we can either demonstrate their existence, or develop a new model that does not require one or both of these.
Within the currently most prominent Lambda-Cold Dark Matter (ΛCDM) model, the presence of dark matter serves to explain observations made of galaxies, as their shape and rotation cannot be explained using just the observable luminous (baryonic) matter. Following Kepler’s Second Law, there should ultimately be an estimated 85% of dark matter in the Universe, with the rest being ordinary matter. This presumed existence of dark matter (the Cold Dark Matter in ΛCDM) is backed up by a number of other observations including of the cosmic microwave background (CMB) and gravitational lensing, even if we do not know what this matter is.
Dark matter is postulated to not interact with the electromagnetic field, which is the sense in which it is “dark”, while still imposing gravitational effects so that its presence can be deduced by gravitational lensing. Ultimately, gravitational interaction would be the primary way that dark matter could interact with the rest of the Universe, forming a massive part of its evolution due to its abundant presence.
Similarly, the existence of dark energy was deduced from redshift observations, primarily by using super novae of type Ia (SNIa) as a consistent reference point, albeit one limited by the accuracy of our measuring equipment. Based on the observed redshift and the energy that would be behind the needed acceleration, there should be more energy driving the expansion than we are aware of, or have so far measured as part of vacuum energy (also known as zero-point energy). This dark energy is calculated to form about 68.2% of the energy-matter balance within the Universe, with 26.8% being in the form of dark matter and a mere 5% ordinary matter.
Naturally, without more evidence and without reducing measurement error sizes, it’s hard to say whether any of this unseen energy and matter exists, is just a measurement error, or if the gravitational physics is just wrong. To this end we need instruments such as Euclid to refine and add to the available evidence.
Euclid’s Mission
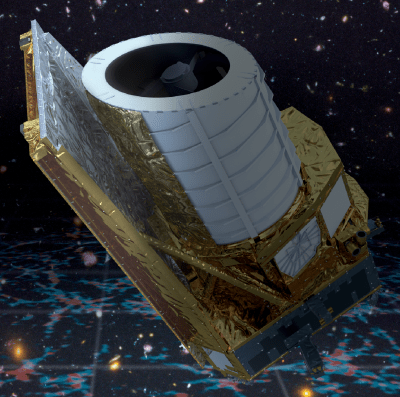
Against this background, Euclid’s primary purpose is to continue the research on the exact nature of dark matter and energy by refining previous observations made of the shape of galaxies, as well as the redshift of objects like Type Ia supernovae. Earlier missions included the Planck space telescope (active from 2009 to 2013) and the Wilkinson Microwave Anisotropy Probe (WMAP, 2001 – 2010), with now Euclid and the James Webb Space Telescope (JWST) having taken over most of the observation tasks, with both based at the Lagrangian point L2 to keep them in the shade of the Earth.
The Euclid space telescope features visible light and near-infrared cameras, with both instruments being optimized for these specific observation tasks, whereas JWST is used for general observations in infrared. Although Euclid is currently still on its way to the L2 point before it can commence its science mission, JWST has been making relevant observations already, with some interesting data being published last year, such as last year’s findings on the redshift of very young galaxies (arXiv paper).
Both JWST and Euclid have near-infrared cameras, and can be considered to be complementary, but Euclid is solely dedicated to answering these specific questions in cosmology, allowing it to perform observations continuously and thus gather significant amounts of mission data over its projected six year lifespan.
Mission Implications
What could be the implications of this research on dark matter and dark energy? Clearly, they are both essentially placeholders until we can ascertain whether our observations regarding the Universe’s expansion and the physics of galaxies can be explained in some other way. It wouldn’t be the first time over the past 120-odd years that our assumptions about how the Universe works and what its ultimate fate will be have fundamentally changed in the face of new evidence.
If the ΛCDM model holds up, then the question is whether we can define what the nature of dark matter and dark energy is. So far a number of hypotheses exist here, including sterile neutrinos and many more. For dark energy, the simplest explanation would be that it is an intrinsic energy of space (akin or equal to vacuum energy), yet many more options are possible. There is also the possibility that Euclid and other missions will provide us with data that puts into question many of the assumptions made, or leads us towards new possibilities.
Although none of what we learn via these observations is likely to change our fundamental understanding of the shape and rotation of galaxies, nor will it likely override Hubble’s law regarding the expansion of the Universe based on observations of other galaxies moving away from our own, what it will affect is our understanding of the how and why. Ultimately, by developing our elementary understanding of the energy-matter balance in the Universe and its exact nature we should have a much better idea of both how the Universe began, and how it will ultimately end.
Yet regardless of what we’ll discover, the essential part is that through Euclid, JWST and whichever spacecraft will follow them we’ll slowly untangle the biggest challenge for science, in the form of the Universe itself. This is a challenge that has kept the world’s greatest minds occupied for well over a century now, with the tantalizing promise of understanding the very foundations of existence itself.
“Based on the observed redshift and the energy that would be behind the needed acceleration, there should be more energy driving the expansion than we are aware of, or have so far measured as part of vacuum energy (also known as zero-point energy).”
No, this is backwards. There’s no way to measure zero-point energy in QFT, because attempts to do so blow up to infinity – you have to just renormalize “zero energy” to an empty state. So you don’t know what the true zero is.
If you naively attempt to calculate it using naturalness arguments and quantum gravity scales, you get numbers that are violently *too big* – this is the “cosmological constant problem.” Dark energy is currently dominant, but still *exceptionally small* – about 3 protons per cubic meter in terms of energy.
That being said, there’s no real strong argument for connecting the “zero-point energy” idea in QFT to the cosmological constant anyway. It would’ve made for a simple explanation, but the Universe has no requirement to be simple.
Are both spacecraft on the same L2 point? I understand that it’s more of a region of space where they dwell around in complicated “orbits”, but isn’t it challenging to keep them from colliding?
They’re in halo orbits around L2. Lagrange points except L4/L5 are all unstable, so you orbit it instead of being located at the exact point. You prevent multiple spacecraft from colliding by just making sure they’re in different non-intersecting orbits.
Really nice spots for telescopes since they’re very clean locations due to being unstable. JWST, Euclid, and the Gaia spacecraft are all there, and several others were there as well (Planck, WMAP) before being moved out when they retired.
Sorry, still not buying the dark matter/dark energy nonsense. Instead of saying “Hmm, maybe the theory’s wrong” someone said “Quick! Make something up!” Placeholder? It has to be a placeholder for a *thing*. There has to be a *thing* to hold a place for. It’s handwaving pure and simple. Let’s change the names to Boogeyman and Sasquatch.
Also it seems that the author doesn’t understand what vacuum/zero-point energy can and cannot do. Nothing or almost nothing and anything or almost anything respectively.
It is all ‘theory’. Even the ‘big bang’ is just a theory. And your right, to fit a theory, man has to make stuff up to make it fit there idea. Now they are still trying to ‘prove’ it. Point is I agree with you :) . But the telescope will give man more data to manipulate and ‘maybe’ more insight. If it completely disproves the theory then ‘we know’ and can move on … and visa versa. Some noses may be point out of joint for awhile though as life-long work possibly being moved to the side.!!!
I personally would like to see more activity on ‘how’ to ‘move’ about the universe in a ‘timely’ manner than spending time on millions of years in the past, that has no real bearing on our immediate future.
How do you know that’s not where we’ll find the answer?
We’re already moving through the universe in a ‘timely’ manner. You want to move faster, just because you’re from somewhere that’s incredibly hot in universal terms.
“Even the ‘big bang’ is just a theory.” Not disagreeing. I like to phrase it as “The universe *looks* like there was a Big Bang.”
If the theory predicts something that, up till now, hasn’t been observed, but then you find it and observe it, that is somewhat more powerful.
Has Big Bang theory predicted anything we haven’t previously observed?
Yes. The Cosmic Microwave Background.
The Big Bang as a quantitative model was developed back in the early 1930s-1940s, with major contributions by Gamow, Alpher, and Herman. The CMB was discovered in the 60s, to good agreement with the predicted temperature (by astrophysical standards).
There are also a number of “anti-predoctions” from big bang nucleosynthesis as well: primordial isotopes other than hydrogen, helium, and lithium-7 were predicted to be ultra-rare, and they have never been found (although not *impossible* to find).
“Placeholder? It has to be a placeholder for a *thing*.”
There are *plenty* of possible explanations for both of them. The problem is that the observations we have now are royal pains in the neck and prevent us from distinguishing anything. Pretty much the most difficult thing in astrophysics/physics is that the Universe kept taunting us with easy explanations for both and then ripped the rug out from underneath.
Keep in mind even if you completely took away all of the dark matter/dark energy astrophysical observations, there are still *basic physics* reasons that would make you think both of them existed.
For dark energy: quantum mechanics seems to *scream* at you that the vacuum itself should have energy. There’s a friggin Higgs field at an excited energy level *everywhere throughout space*! So before you had to explain why there wasn’t *any* “dark energy.” Now you have to explain why it’s so small. But you had to explain it either way. You also have the inflation problem, which again gets explained by some constant energy field permeating all of space, although that’s an astrophysical issue (but one that’s early enough that it’s really particle physics).
For dark matter: quantum field theory has a *ton* of goofball problems that really, really could use additional particles to fix. The lack of matter/antimatter symmetry in the strong force, neutrino masses, the matter/antimatter asymmetry in the Universe, why are there 3 generations of matter, etc.
So yeah, they’re placeholders for something. *Lots* of possible somethings. That’s the problem. I mean, for dark matter specifically, if it *isn’t* coming from a particle explanation, that really sucks, since you can’t use it to explain any of the *other* problems you have.
“the vacuum itself should have energy” — Sure, virtual particles pop in and out of existence all the time. The problem is the books balance and/so there’s nothing you can do with that energy.
“Not being able to do anything with the energy” doesn’t mean it has no *effect*. That’s the entire point of the Casimir effect treated as a zero-point shift (which it *isn’t* – see Jaffe’s 2005 paper – but it’s an important thought experiment).
Likewise, there’s also a difference between *us* (matter) not being able to do anything with the energy and the Universe not being able to do anything with it.
“dark matter/dark energy astrophysical observations” Not sure I understand you. There *are* no such observations because dm/de are *unobservable* which gets one out of a lot of messy lab work. (Difficult to observe something that doesn’t exist.)
Apparently DAMA/LIBRA is seeing *something*
Oh boy. This’ll be long. I’ll stick with dark matter since it’s easier, since dark energy’s newer and weirder. Although saying “dark energy doesn’t exist!” is silly – people have been talking about and setting limits and estimates on the cosmological constant for a century. It was only called “dark energy” when we actually measured it. Oh, and it was pretty darn close to where it was estimated to be, mind you!
But it’s important to note that “dark matter” is really just a name for an *entire set* of puzzles that can be explained with the same explanation: *something* that gravitates, with positive pressure, does not couple significantly to light, and dominates the Universe over baryonic matter by roughly 5:1.
The problem with saying that “dark matter doesn’t exist” is that all of those puzzles now need to be solved *independently*. You need to explain the CMB peaks separate from galaxy rotation separate from galaxy clusters separate from large-scale structure separate from the Bullet Cluster interaction dynamics.
Your immediate response might be “but that’s easy, just modify gravity, that’s what’s wrong!” And the issue with that is that really, you’re just pointlessly changing the name. In order to explain all of the above observations with one modification… you end up with something that for all intents and purposes is dark matter.
In a lot of ways, saying “we don’t observe dark matter” is like saying “we don’t observe black holes.” We don’t observe them – we can’t, they don’t interact with things outside of gravity. Sure, we’ve imaged a black hole’s horizon, but hey, who’s to say that gravity just doesn’t work differently to exactly explain what we see and there’s nothing actually there? Except if you twist your theory around enough to make it fit the observations, to an astrophysicist, you haven’t “disproved” black holes – you’ve just explained them.
And if your issue is “I can’t believe there are massive particles out there that are zipping through us and practically never interact” – you’re about 70 years too late for that idea. Neutrinos fit the bill there, and in fact they *are* part of the dark matter explanation, just not a particularly large one – they make up about 5% of dark matter in the sense that I’m using it above.
bleah, that last statement was supposed to be “they *could* make up about 5% of dark matter in the sense that I’m using it above.”
Looking at all of your comments, you really are a vehemently grumpy person, eh? Well at least with a username like that I can tell you are humble…
But to respond to a couple of your comments:
The observations related to dark matter and dark energy refer to the observations that we can only make sense of so far using them. Such as the accelerating expansion of the universe.
So instead of trying to refine the theories by using such placeholders, you would prefer we just throw it all out? And then what? Haven’t you ever seen empirical equations that evolve over time by expanding a single coefficient into one’s that depend on more inputs? Such as manning eqn using roughness size parameters vs chezys eqn. Just because you don’t have the whole thing right doesn’t mean the rest is wrong. And that the “wrong eqn” isn’t useful in some circumstances.
Also your criticism of the author is unfounded and just rude.
“So instead of trying to refine the theories by using such placeholders, you would prefer we just throw it all out?”
This is a really good point and what I was trying to say above. There’s a difference between a physical theory (one you have a fundamental explanation for) and a phenomenological theory (one just designed to match observations).
Dark matter isn’t a physical theory, it’s a phenomenological one – the only way you could “get rid of it” is by explaining the various observations *separately*. If you explain them all with “some other thing,” then that thing will have to approximate to the behavior of dark matter.
Example Finaglers’ constants:
= Answer you want / Answer you got
= Answer you want – Answer you got
Tensor forms left as an exercise for reader. See also Fits.
This is literally how the entirety of quantum field theory renormalization works.
Euclid, with its two very different types of measurements approach, will provide a value for Einstein’s cosmological constant, very precisely, plus or minus ten minus six. If it is the value the most commonly prominent model expects, then so be it. Personally I would love to hear that it is a very different value: all our models are based on « matter », since the Greeks, then Newton, Kepler, etc. while matter as we usually think of it represents only an extremely tiny fraction of the barely 5% of « ordinary matter » of this « commonly prominent model ». Most of the « ordinary matter » in this model being lonely wandering proton and electrons, all sorts of radiations, etc., not the usual matter we touch and normally think of. Hence I would not be surprised at all that the result of this Euclid endeavor is a total shakeup of our establishment. Building a model based on the 5% of our universe we observe at the moment is simply all we have at this point, no shame about it. So Euclid finding anything about the other 95% we now know is around, and just do not know anything about, will almost certainly deeply change our perspective, affect this commonly prominent model, and restructure our views of where we come from. You each should in my humble opinion be prepared to fasten your seat belt, it is in my opinion one of the most potentially revolutionizing mission of the decade.
“plus or minus ten minus six” Reminder: there are people here for whom English is not their first language, so
“plus or minus four”? Whaaa?
I suspect it’s 10^-6, not 10-6.
Yes, thanks.
That is a refreshing look. Thanks.
At only 5% of the universe, what if *we* are the dark matter?…..
… and, does it matter?
“Dark” in “dark matter” mostly doesn’t mean “unknown,” it means “does not couple strongly to light.”
The “dark” in “dark energy” has more of the “unknown” sense.