Outdone only by nuclear fusion, the process of nuclear fission releases enormous amounts of energy. The ‘spicy rocks’ that are at the core of both natural and artificial fission reactors are generally composed of uranium-235 (U-235) along with other isotopes that may or may not play a role in the fission process. A very long time ago when the Earth was still very young, the ratio of fissile U-235 to fertile U-238 was sufficiently high that nuclear fission would spontaneously commence, as happened at what is now the Oklo region of Gabon.
Although natural decay of U-235 means that this is unlikely to happen again, we humans have learned to take uranium ore and start a controlled fission process in reactors, beginning in the 1940s. This can be done using natural uranium ore, or with enriched (i.e. higher U-235 levels) uranium. In a standard light-water reactor (LWR) a few percent of U-235 is used up this way, after which fission products, mostly minor actinides, begin to inhibit the fission process, and fresh fuel is inserted.
This spent fuel can then have these contaminants removed to create fresh fuel through reprocessing, but this is only one of the ways we have to extract most of the energy from uranium, thorium, and other actinides like plutonium. Although actinides like uranium and thorium are among the most abundant elements in the Earth’s crust and oceans, there are good reasons to not simply dig up fresh ore to refuel reactors with.
All About The Neutrons
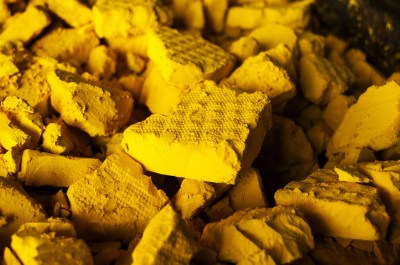
Forming nuclei as heavy as uranium requires something more than the standard nuclei-forming process (s-process) in the average star. An integral part of nuclear astrophysics, the s-process stands for ‘slow’ and refers to the rate of neutron capture of nuclei. Essentially it refers to the number of neutron captures that happen before nuclear decay can occur. The s-process is sufficient to create many of the elements we know from the periodic table heavier than iron (Fe) via various decay chains, with the remaining elements requiring the much higher neutron-density flux of the rapid, or r-process.
The difference between the s- and r-process is quite severe, with the s-process requiring seed nuclei from the proton, or p-process, while the r-process with many orders of magnitude higher neutron capture events can create its own own nuclei and from them the heavy elements such as the actinide series that include americium, plutonium, as well as a range of synthetic elements commonly referred to as the transuranium elements: the transuranics. Within an astrophysical context, however, neutron stars are probably the biggest source of these heavier elements.
Once this proverbial stardust has gone through planet formation, the first multicellular life can evolve into intelligent life over the course of a few million years. After this, said intelligent life can then proceed to dig up uranium ore for use in a nuclear fission reactor.
During the millions of years that humanity took to evolve to this point, however, the fissile U-235 has largely undergone decay already, while the fertile U-238 isotope, which can become fissile upon neutron capture, now makes up most of the uranium ore recovered today. This is why for certain types of fission reactors that use slow neutrons the uranium fuel is ‘enriched’, meaning that the amount of U-235 in it is increased from the approximate natural 0.7% to 3 – 5% for use in LWRs.
After the optional enrichment step, fuel fabrication can commence. The typically ceramic fuel pellets are then inserted into a fission reactor and the U-235 is exposed to a neutron source that then kickstarts a nuclear chain reaction.
Reprocessing And Pyroprocessing
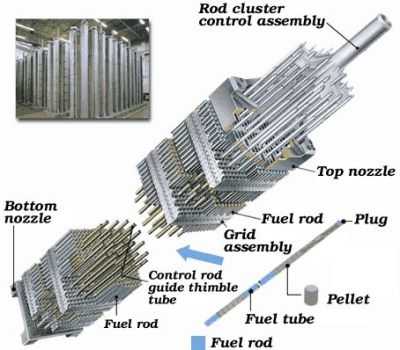
The exposure of fissile isotopes to neutrons results in rapid nuclear decay, along well-known decay chains into a range of different isotopes. Some of these are helpful – like fissile Pu-239 – but minor actinides, Pu-240 as well as other isotopes that are formed inside the ceramic LWR fuel pellets will interfere with the nuclear chain reaction, reducing its efficiency. After replacing such spent fuel with fresh fuel, the spent LWR fuel can then be processed in a number of ways to use up the remaining fissile isotopes, the primary ones being reprocessing and pyroprocessing.
Effectively, the spent LWR fuel isn’t so different from the uranium ore, with the isotopes being separated from the ceramic material rather than the minerals in uranium ore. This process can be performed in a few ways:
- hydrometallurgy – dissolving into an aqueous solution, e.g. PUREX.
- electrometallurg – using electric current.
- pyrometallurgy – smelting the pellets to separate the metal from the mineral.
Of these hydrometallurgy is the oldest method, as well as the one most commonly used. France’s La Hague reprocessing plant processes about 1700 tons of spent fuel per year using the PUREX (plutonium-uranium-extraction) method which uses concentrated nitric acid to assist in separating the uranium and plutonium via solvent extraction steps along which various other isotopes (e.g. neptunium for Pu-238 production) can be separately recovered.
The remaining liquid after PUREX contains about 3% of the original used fuel material, which is generally disposed of as high-level waste with this reprocessing process. The recovered uranium and plutonium is then used together with fresh uranium to create a blend (mixed oxide, or MOX) fuel that can be used in LWRs again. There are a few variations on the basic PUREX process, but they all come with various trade-offs and the necessity for a long and tedious process.
This is where pressurized heavy water reactors (PHWRs) and fast neutron reactors (FNRs) can provide the missing link to fully close the uranium fuel cycle.
Fast Neutrons
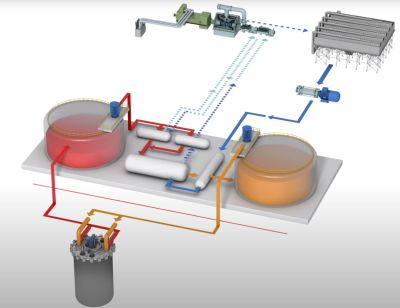
The PHWR reactor uses so-called ‘heavy water’ (deuterium), which unlike its lighter sibling does not moderate neutrons, thus allowing for a PHWR to also work with fast neutrons. Unlike the slower, thermal neutrons in water-moderated LWRs, this means that these reactors can also use much more of the fertile isotopes in the fuel. An interesting approach here is the direct use of spent LWR fuel in Canadian-designed CANDU PHWRs, called DUPIC. Normally CANDU reactors use either natural or only slightly enriched uranium fuel, using which they can achieve very high burn-up rates.
This DUPIC method does not require any reprocessing, but takes the ceramic fuel and merely puts it into fuel assemblies that work in the CANDU reactor. This and similar approaches are being trialed by South Korea, and China.
Effectively the use of PHWRs is similar to FNRs, which are a popular choice for closing the uranium fuel cycle, including the currently under construction Natrium reactor by TerraPower. By increasing the selection of available neutrons (thermal and fast), FNRs can effectively function as a breeder (turning fertile isotopes into fissile ones) while burning up all fissile isotopes. As this includes minor actinides and transuranics, this means that in an FNR theoretically every single last bit of radioactive fuel can be used, leaving no radioactive waste to handle. Through a constant process of neutron capture within the reactor, the isotopes will rush through their decay chains until finally reaching a state where their nuclear cross-spectrum no longer makes them viable nuclear fuel, or a radiological hazard.
So why is that we don’t fully burn up uranium fuel fully today, but instead usually use a once-through fuel cycle?
It’s The Economy, Silly
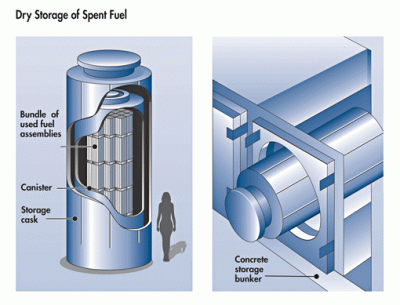
One defining characteristic of nuclear fission plants is that the fuel costs end up being practically a rounding error over their operating life. This is defined both by the abundance of uranium ore and the relatively small amounts of it needed by an LWR’s roughly two-yearly refuel cycle. Although countries like France reprocess virtually all of their LWR fuel, this is more a part of their energy independence strategy, even if it has the benefit of minimizing the amount of nuclear waste. While the PUREX process results in high-level waste, this type of waste also decays very rapidly, reaching background levels within a matter of decades.
With the current resurgence in new nuclear construction, uranium commodity prices have also gone up, along with newly (re)opened uranium mines getting a lot of investor interest. Although uranium is incredibly abundant in the soil (with much more dissolved in the oceans), economics says that with more economical ways to close the uranium fuel cycle, reprocessing, reusing and burning up uranium fuel becomes the logical approach.
Before even tapping into fertile isotopes like thorium 232, the uranium we can extract today for energy production should be enough to last us many thousands of years. All because of the neutron flux in stars capturing all this energy, which is a process that continues to this day throughout the Universe.
Featured image: Solar flare on the Sun’s surface. (Credit: NASA)
The idea of leaving rapidly decaying nuclear waste is an important advantage. I’m not superstitious about fission power, but having waste products that last 100,000 years is a serious problem – and as far as I’m aware only Finland, in the entire world, has made a serious effort to inter them. If PUREX allows a reactor to produce only short-lived waste, it would solve a major problem with nuclear power. No doubt it has other disadvantages…
Nuclear fuel was radioactive before it came out of the ground and it’s less energetic when buried again. As for miners in 99,999 years randomly digging deep holes in a planet naturally full of things like asbestos, arsenic and radon without the basic precautions that would detect man made decay products feels far fetched.
Nah it’s not a problem. You just throw the lawyers and activists into the reactor for pre-processing, then you take out the waste and bury it miles deep in the middle of nowhere in Nevada or Utah. The waste will never will be an issue.
Nah, that waste will be far too toxic. I’ll take the plain radioactive stuff instead thanks.
“Nah, that waste will be far too toxic. ”
You’re referring to the lawyers and activists?
B^)
Its not the longer lived isotopes that are the biggest hazard. Its the shorter lived isotopes like CS-137 and SR-90 that give off nasty radiation.
Welcome to opposite town!
You tell me what is more “dangerous”.
a. Something that kills 100% of people who encounter it for 100 years.
b. Something that 10% of people who encounter it for 100,000 years.
The transuranic waste, which is the long lived stuff, is ORDERS OF MAGNITUDE more “dangerous” than anything else in there, because it stays dangerous ‘forever’ on human-civilization spanning time-scales.
That is why we cannot implement large scale nuclear power generation.
We have no way of dealing with the transuranics.
Infinite custodianship is not an option.
But that stuff is produced naturally in uranium and thorium bearing rocks, and we’re constantly exposed to it. If you have a granite kitchen counter, you have “transuranic waste” in your house. It’s a great part of why we have background radiation in the first place.
In other words, it’s not actually that dangerous. Forget the “hot particle” and LNT theories that suggest no amount is safe – life has evolved to deal with ionizing radiation and radioactive elements, because they’re all around us anyways.
Hmm, you say when you speed up a process from “in hundreds of million years” to “in hundreds of million microseconds” the amount of the decaying products at the end is the same? Try placing a leaking dish under a dripping faucet, watch the resulting level in the dish, then open the faucet and watch the level some more.
That’s shifting the goalposts. Transuranic waste isn’t dangerous because it’s long lasting – that point actually makes it less dangerous. It’s dangerous because it’s concentrated, which is a different matter.
And transuranic waste isn’t going to be around for “100,000” years. Most of the transuranic elements produced in a nuclear reactor have a half-life of a couple days to a couple hundred years. After a 1000 years they’re pretty much gone. There’s only a handful of more stable elements like Californium that have a half-life of 800-900 years, but that too would be basically gone after 10,000 years, not 100,000 years as erroneously claimed. Plus, the more stable elements are valuable as recycled fuel, so these materials would be recovered rather than thrown away. Californium is a neutron source and it’s actually used to start nuclear reactors.
But… the article you’re commenting on literally has a paragraph on burning up transuranics?
Ummm… You can hold pure u235 metal in your hand. Let me see you do that with pure cs137.
But what do I know, I’m only deal with it at work.
Seeing all the fear around nuclear waste and the outcry over the zero deaths at Fukushima and the less-than-if-it-were-a-coal-plant number of deaths from Chernobyl, I can’t help feeling the hand of the fossil fuel industry in stopping the one technology that could realistically avert climate disaster.
It’s not like nuclear waste is a non-issue, but it would be nice to see it more frequently compared to the consequences of fossil fuels. A comparison of human deaths and square kilometers rendered uninhabitable per terawatt-hour should be in the foreground of every one of these discussions.
There’s no need to attribute much of it to conspiracy, though. Hanlon’s Razor accounts for it quite well; just assume that journalists are no smarter than the average schmuck and are just as vulnerable to overemphasizing dramatic but rare events. Add in the economic incentive to produce sensationalist clickbait instead of sober reporting, and it’s no wonder that a singular event like Fukushima gets a thousand times more coverage than the constant background of dead coal miners, cancer and asthma clusters downwind of plants, lush valleys turned into barren moonscapes of tailings and ash, and so on.
According to these metrics, most of human history would have been dismissed as a “conspiracy.” Believe it or not, human agency does happen. The world is not just a clockwork of materialism–human will matters, and people do plan things (and most often they don’t tell the entire world about it).
Defining conspiracy not as
“Area 51 lizard aliens telepathically control world events”
but instead
“Any event in which a smaller group covertly pursues its own interests against the interests of the out-group”
is a form of self-lobotomy. That stuff happens every single day, and drives most of human history.
No, there’s a difference between a conspiracy and a conspiracy theory. Conspiracy theories need a different name, really. They’re not conspiracies, they’re some wackjob on the Internet. You can’t give up the term “conspiracy” to those, unless you’re going to give another name for the crap that often happens with large corporations burying stuff illegally, and it seems pretty asinine to let wackjobs ruin a perfectly good word.
“Area 51 has lizard aliens” is a conspiracy theory.
“The Phoebus cartel conspired to limit light-bulb life” is a conspiracy.
When somebody says something like “There’s no need to attribute much of it to conspiracy” I think that most of the time the rhetorical trick being used is to conflate the former with the latter.
“Conspiracy theory” as a term was actually workshopped by PR man Cass Sunstein in order to come up with a media strategy to discredit people who questioned the Warren commission. Strange but true. Otherwise I agree with your basic points. The meaning of the term has evolved. But it is still very often used in bad faith, trying to minimize or make incredulous even the most common and petty plots of man.
“and it’s no wonder that a singular event like Fukushima gets a thousand times more coverage than the constant background of dead coal miners, cancer and asthma clusters downwind of plants,”
I actually think it’s more likely that the issue is primarily that the constant background of coal issues happen in the poorest areas of the country that uh, don’t attract very many journalists.
The less cynical side of me would also point out that the other issue that nuclear waste had is that its risks were known straight up at the creation of the plant, so everything needed to be documented and detailed and there’s a nice liability paper trail if anything went wrong, and that’s not the same for stuff like coal mining, so it’s easier to bury/hide/dodge liability.
… wait, that wasn’t all that less cynical.
Fukushima was a minor burp compared to chernobyl. Only reason its regurgitated constantly is because of the illiteracy and absentmindedness of this generation.
I think the truth is boringly somewhere in the middle. Fossil fuel interests have, in fact, astroturfed opposition to nuclear power in the past, but also the public are organically prone to nuclear hysteria all on their own. It’s unfamiliar, it’s associated with secret apocalyptic weapons, it involves invisible poisonous light – screenwriters were never going to leave all that on the table.
And then there’s bad luck like the (silly) movie /The China Syndrome/ coming out the week before the Three Mile Island accident. Because people are wired for drama, not evidence, this felt like the movie’s scaremongering was vindicated, even though the rational takeaway should have been “this IRL nuclear accident didn’t harm anyone”.
This is a general theme in public policy: when people are afraid of something, they end up seeing their fear itself as evidence of the thing. E.g. the increasing fear of crime even as crime rates steadily decrease. And the media have every incentive to fuel the process.
Uranium mining is an ecological disaster, at least the way it has been done in canada. It can easily compete in devastation with any other types of mining.
Rare earth minerals mining is worse, since the waste products are uranium and thorium bearing rocks ground up and left in piles under the weather. At least the whole point of uranium mining is to extract the radioactive metals, whereas with REEs you’re left with literal mountains or nuclear waste just sitting out there in the open after the extraction.
That’s one of the reasons why the rare earths industries were moved from the western countries to East Asia, where the environmental laws are more relaxed and the local governments are willing to ignore the waste dumping – especially since it affords them near total dominance of these materials on the world market.
The issue with NPP is even if they themselves don’t emit much CO2, they still require very precise steelmaking, and in terms of emissions, making a ton of alloy steel is equivalent to 10000 people driving their cars daily for over 7500 years. If we seriously think about decarbonisation of our environment, then we should focus our attention on sustainable architecture and solar power, not massive monuments to 1950s nuclear hype.
I call wholesale BS. According to the EPA, an average passenger vehicle emits 4.6 tons of CO2 per year. You are saying making a ton of steel is equivalent to 75 million car-years or 345 MILLION tons of CO2. That’s an overestimate by a factor of about 200 million. How can you even spout such nonsense without recognizing immediately it is ridiculous?
When you have invented a solar plant that will work near the polar circle from november to beginning of march, let me know. Until then, solar power is useless.
… as useless as refrigerators
Fridges are a fair bit easier to use, but technically irrelevant for most of the year in the nordic countries. When the outside temperature is under 10 C for 8-9 months of the year, refrigeration isn’t really an issue.
It was quite common back in the day for homes and even city apartments to have a “cold closet”, which was either a box, a cabinet, or a small pantry with a hole through the wall to the outside. People only started buying fridges around 1950-60, even though they were introduced in the 1920’s. In the 1970’s there was the oil crisis and energy prices went up, so people started wrapping the housing in plastic to eliminate air leaks, and that did away with the hole-in-wall cold closets.
Do you know how much carbon emissions it takes to produce silicon for solar panels?
Hint: refining silicon also uses coal, as the metal is reduced by a chemical reaction with carbon and emits CO2. Making solar panels involves converting methane (CH4) and silicon into silane (SiH4), which also releases CO2 and involves some nasty solvents that tend to leak out of the process and destroy the ozone layer. The ozone layer hole started to close, but it’s now growing again because of these chemicals being emitted from China where 80-90% of the solar panels are made.
A nuclear power plant requires a lot less – orders of magnitude less – materials per kilowatt-hour compared to solar panels and all the supporting infrastructure around them.
It’s not just the hand of the fossil fuel industry. It’s the hand of the climate NGO industry. They can’t allow nuclear because it would solve the problem and end their infinite grift. Pollution and climate damage are real, but simultaneously it is also true that people are exploiting that fact for the sake of their own power. And horrible, garbage renewables which will never work are a lucrative mega-industry in their own right.
Agree!
100 percent on the money. I’ve seen so much snake oil in the pursuit of the “green dream” that I’m quite happy to comment on all the items of that type on facebook and let people know it’s baloney. Get’s to be fun honestly.
Im a proponent of nuclear power, but Chernobyl was, and is, the worst catastrophy in nuclear history and the amounts of dead will never be known.
While technically correct, the scale of how many people will eventually die can be estimated, and it’s not even in the millions. Hardly in the thousands, since the radioactive elements that were released had a relative short half-life and so far more than half of the nastiest ones have basically disappeared.
If you attribute e.g. every future cancer death in the region to radioactivity, you’ve fallen victim of propaganda. There are so many other causes, that after a point these other points become the dominant factor of why the person is dead. The leading cause of death for the Chernobyl survivors is still understood to be alcoholism and poverty.
Russia already closed their cycle with BN-xxxx fast neutron reactors. Beloyarsk NPP with two fast neutron reactors is running on the fuel made from waste from regular NPPs. And it runs commercially, not in experimental mode. For years already, since 2018. Fast neutron reactors waste then processed for use as fuel on regular NPPs. More BN-xxxx reactors are in stages of building.
It is a huge step forward, because instead of 3% of energy extraction it is possible to extract up to 30% from the same amount of fuel, along with resolving problems with waste from regular NPPs.
I can’t really understand why western countries don’t do the same already, having much more money for such projects than Russia. AFAIK, China is building (or already built) experimental fast neutron reactor, but I don’t know if they really closed cycle with it or not.
The problem we have in Western countries is what we call “Tree-Huggers“ that have instilled and installed themselves at all levels of our governments, and prevented innovation in nuclear reactor design.
I wouldnt trust a russian power plant any further than i can throw it. Having russians build nuclear plants is equivalent to letting a former alcoholic run a liquer store, sooner or later he will delve into the stock and fall off the wagon.
“Within an astrophysical context, however, neutron stars are probably the biggest source of these heavier elements.”
It’s not exactly that the neutron stars are the source, it’s the process of forming the neutron stars that’s the source. The neutron stars themselves aren’t producing anything – when the star runs out of fuel and collapses, you start to get rapid neutron stuffing due to absurd neutron densities: those elements can get ejected either basically right away in the supernova or later if there’s a binary merger where some of the neutron star material gets ejected.
Also, the s-process doesn’t need seed nuclei from the p-process: it needs heavy seed nuclei from something, meaning it’s a secondary process: you’re not going to get significant s-process element formation if your star originally started from H/He (a pop-III star, with zero metallicity). In other words, the s-process needs seed nuclei (primarily iron) from former generations of stars, because you’re not going to have any iron in a star that starts with H/He because once it reaches iron, it’s dead (fusing iron takes energy rather than producing it).
Not sure where you saw stuff on the p-process: the p-process is proton capture (as opposed to neutron capture) and requires very different conditions. The B2FH paper (the seminal nucleosynthesis paper) referred to the p-process as occurring in supernovae, but that turned out to be wrong. The entire point of the p-process at all is that there are a few isotopes which are proton-rich (rather than neutron-rich) and so can’t be produced by the s or r processes (which are neutron-capture processes).
The issue with p-process elements is that it requires both high proton density and high energy, which is tough: a lot of p-process elements are likely formed from the shock front of a supernova, as opposed to the collapse of the supernova. Basically, imagine that the shock front reaches the older shell of the star (with lighter elements) and you just superheat/supercompress them.
Essentially if you look at one of the Charts of the Nuclides (which typically has Y as proton number, X as neutron number), anything basically on the ‘stable’ line is s-process, stuff above the stable line is p-process, and stuff below is r-process. This is super simplified but a decent understanding.
Pretty strange to think that everything you know and love was at some point cleaved off a neutron-star-to-be, with the neutronium core being all that remains
Well, not most of you, the majority of stuff humans are made of can be made in lighter stars via straight up nuclear fusion, churned through the star itself, and that stuff likely just gets sloughed off by stars over time. There’s a reason why we’re made from CNO: because every star can make CNO.
But it’s totally true that the most important molecule in your blood is a huge protein trap for a tiny number of precious atoms almost certainly ripped off of a newly-forming neutron star.
heh i am a frederik pohl fan so i know it as CHON and i think it’s illustrative that you take the H for granted. we really are the lighter elements. crazy that heavy-element technology is, by apparent necessity, a function of time
To be clear I left H off because it’s not (significantly) made in stars at all – hydrogen’s primordial.
The biology types would usually say CHONPS – you can make stuff from CHON alone, and there’s some research into whether or not you could make a phosphate-free biology, but the key is kindof that it’s hard to store energy in links with CHON because they’re very strong bonds. Think of it like building stuff with Legos – you could build a bridge fine, because they’ve got strong structure, but good luck building a trampoline. For that you need bonds that are a bit weaker, but still strong – you add in P/S, and now things are a lot more dynamic with energy.
Of course I have my doubts you could get a dynamic and active biology without heavier ions, too: it’s just that life hoards those ions like crazy, and so you get stuff like chlorophyll with 130+ CHON atoms wrapped around a single precious magnesium atom.
in school we learned CHNOPS as main elements in organic chemistry, slightly similar to the german word for spirit (the beverage, not the ghost … or was that liquor? Anyways, cheers)
Great comment thanks
Isnt the S process for elements LIGHTER than iron? The article says ‘The s-process is sufficient to create many of the elements we know from the periodic table heavier than iron (Fe)’
Bad memory! Do better next time. I am wrong
Lighter than iron is just called “fusion.” :)
Thank you Pat
Nice to read something from someone educated who knows what they are talking about.
Makes a nice change from reading uniformed opinions.
Not just nuclear fission, which happens anyway (eventually), but chain-reaction nuclear fission.