To describe the constraints on developing consumer battery technology as ‘challenging’ is an enormous understatement. The ideal rechargeable battery has conflicting properties – it has to store large amounts of energy, safely release or absorb large amounts of it on demand, and must be unable to release that energy upon failure. It also has to be cheap, nontoxic, lightweight, and scalable.
As a result, consumer battery technologies represent a compromise between competing goals. Modern rechargeable lithium batteries are no exception, although overall they are a marvel of engineering. Mobile technology would not be anywhere near as good as it is today without them. We’re not saying you cannot have cellphones based on lead-acid batteries (in fact the Motorola 2600 ‘Bag Phone’ was one), but you had better have large pockets. Also a stout belt or… some type of harness? It turns out lead is heavy.
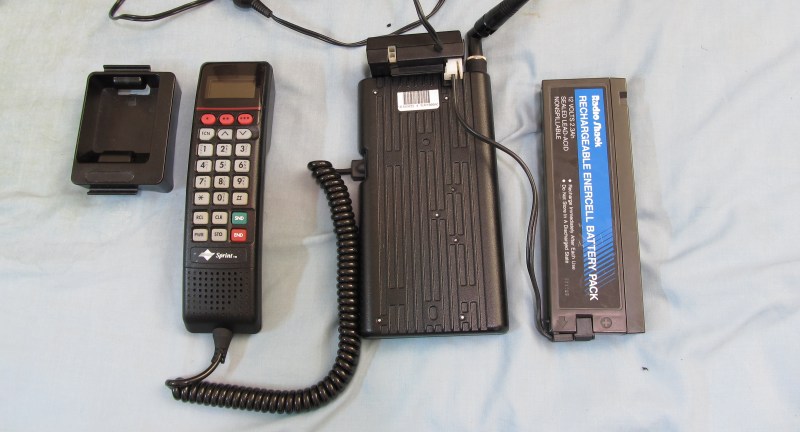
Rechargeable lithium cells have evolved tremendously over the years since their commercial release in 1991. Early on in their development, small grains plated with lithium metal were used, which had several disadvantages including loss of cell capacity over time, internal short circuits, and fairly high levels of heat generation. To solve these problems, there were two main approaches: the use of polymer electrolytes, and the use of graphite electrodes to contain the lithium ions rather than use lithium metal. From these two approaches, lithium-ion (Li-ion) and lithium-polymer (Li-Po) cells were developed (Vincent, 2009, p. 163). Since then, many different chemistries have been developed.
Despite a large number of chemistries, lithium batters still have several parameters in common that are very relevant to using them safely and effectively in our projects. While already familiar to many of our readers, what we will explore today is the reasons behind the characteristics of lithium cells and the rules that govern their safe use. Something less like mysticism and more like materials science.
Capacity: Lithium cells contain electrodes that can store and release lithium ions:
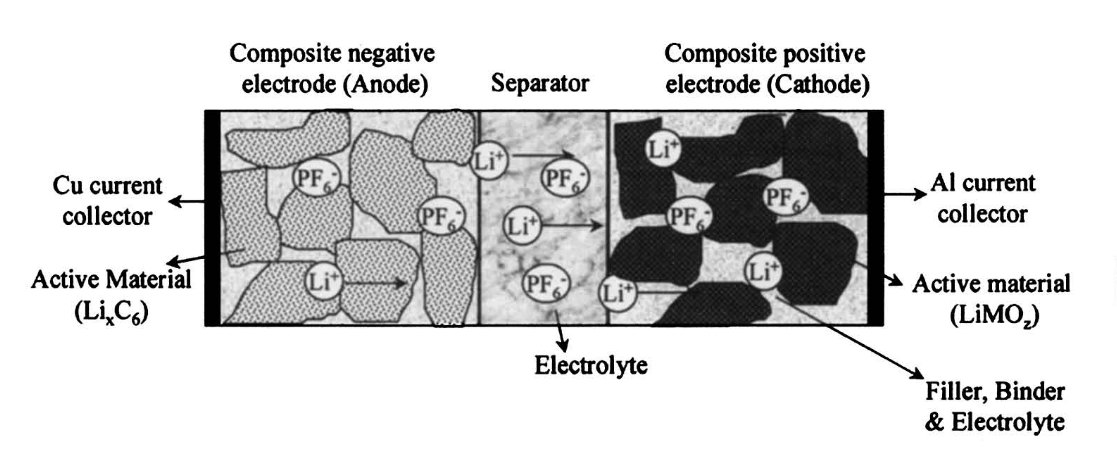
The capacity of the electrodes to reversibly store lithium is the main factor that determines cell capacity since the movement of lithium between electrodes is what causes current to flow (Kang et. al. 2006, p. 977). This is usually measured in milliamp-hours (mAh), the amount of current you can draw constantly in one hour from a fully charged cell before it is depleted. This rating tends to be exaggerated, especially by unscrupulous companies.
C-rate: This determines how quickly you can draw or store current. It is a simple multiplier – a cell with a C-rate of 2 can tolerate a discharge rate twice of that listed as the capacity. For example, in a 200 mAh cell, this means you could safely draw up to 400 mA.
The mobility of lithium out of one electrode, and into the other electrode determines the maximum discharge rate (Kang et. al. 2006, p. 977). This is mainly determined by the composition of the electrode (e.g. LiFePO4), electrolyte, and the surface area of the electrodes (more is better). Surprisingly, the lithium ions do not move far – on the order of micrometers per hour except in cells with exceptionally fast charge rates (and even then micrometers per minute).
It’s worth noting that the C-rate to charge lithium cells is typically significantly lower than the discharging rate (although it depends on the exact chemistry used, some are much faster than others). The reverse reaction in these cases is simply slower by nature, or produces unwanted side-reactions more easily. Besides heating the cell to higher than normal temperatures, charging at faster rates risks a permanent capacity loss through several mechanisms, mainly damage to the electrodes (Ning, Haran & Popov 2002, p. 169)
Undervoltage: We’re told not to let the voltage of lithium cells drop below a given voltage (varies by cell type but often around 3 volts). This is unfortunate because we’re often ‘gifted’ dead lithium cells registering a low voltage that would be nice to use again, for example in a new flashlight. Even though they may charge again, it’s not a good idea to do so.
It turns out that copper foil is generally used in the cells as a current collector. When the voltage drops below a certain threshold, some of the copper in the negative electrode starts to dissolve and migrate. When you then recharge the cell in question, it forms dendrites of elemental copper somewhere they shouldn’t be.
In a best-case scenario, this just decreases the ability of the cell to collect current. The internal resistance of the cell may go up, causing more heat to be generated during charge and discharge. In short, the C-rate is decreased.
In the worst case, this will form a short in the cell while it is charging, causing it to fail catastrophically (Arora, White, and Doyle 1998, p. 3658-3659). This may occur immediately, or some amount of time later. Below is the requisite image of that happening.
Some (but not all) types of lithium cell have a built-in circuit that permanently disables the cell if the voltage drops too low, as a consumer safety feature. Cylindrical lithium cells such as the 18650 are more likely to contain this type of circuit than pouch cells.
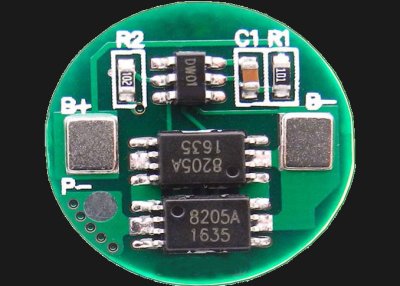
Regardless, it’s wise to safely dispose of any cells that are undervoltage. Any cells above that limit are fair game for salvaging.
Overvoltage: Cells can also be damaged if charged to too high a voltage. The reactions that occur depend on the exact cell chemistry, but a common one is the plating of solid lithium metal on the negative electrode, resulting in a permanent loss of capacity. Lithium metal is extremely reactive and can react with the electrode and electrolyte to release heat and gas, potentially leading to a fire.
Another common reaction is the decomposition of the electrolyte. The electrolyte is typically an organic solvent containing lithium salts, so can be electrolyzed (similar to salt water) producing gases and solids (Arora, White, and Doyle 1998, p. 3652). The gas pressure can cause mechanical failure of the cell, and the solids can form block pores on the electrodes, reducing their capacity.
Thermal runaway: Certain undesirable chemical reactions in a cell both generate heat, and occur faster at higher temperatures. Whether this leads to a fire or just a dead cell is an interaction of several factors, typically cell temperature, physical damage, and charge state (Wang et. al. 2012). This is a well-studied aspect of lithium cells due to liability issues, for example in this awesome study that tracks exploding lithium cells in real-time. The charge state is important as current flowing through parts of the cell or a foreign object (such as a nail puncturing a cell) create local hotspots. This is why any cells you receive by mail might be only partially charged.
Manufacturing defects: With the number of things that can go wrong with lithium cells, quality control is critical. For example, one thing reputable companies test for is metal contaminants in between the cell membranes that can cause shorts:
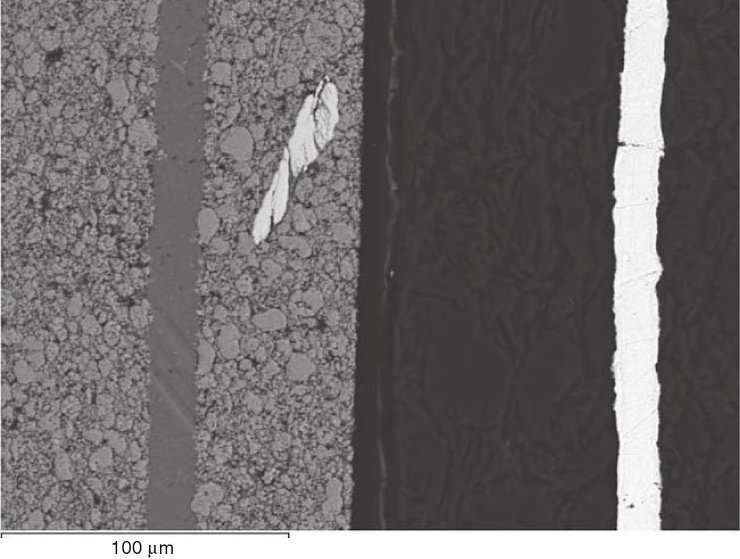
Unfortunately, it’s common practice for some shady companies to not only skimp on quality control but list their cell characteristics in a way that’s beyond optimism and well into the realm of pure fantasy. Generally, the most affected characteristic is the capacity. On the bright side, as long as you observe caution with regards to under/overvoltage and the C rating, the cells will still work at whatever capacity they actually are.
Swelling of lithium cells: Abusing lithium cells as above can definitely cause swelling, especially in pouch cells. However, even if you use them correctly, a few cells will puff up for reasons that are poorly understood.
Here, the main danger is that the pouch cell is punctured by something nearby. The pressure caused by a swelling lithium cell can also crack screens and warp keyboards. Good manufacturers leave a small void to tolerate small amounts of swelling – we’ve opened up laptops with (slightly) warped keyboards to find that this small gap probably saved the laptop.
A special note on LiFePO4 chemistries: Some relatively new LiFePO4 cells on the market are inexpensive, charge fully in 30 minutes, and are unlikely to have any thermal runaway reactions. While they store about 80% of the energy of most other lithium cells, they last for 5 times more charge cycles. It would be exciting to see them used in clever projects!
References:
Arora, P., White, R. E., & Doyle, M. 1998. Capacity Fade Mechanisms and Side Reactions in Lithium‐Ion Batteries. Journal of the Electrochemical Society. Vol. 145 (10), pp. 3647-3667.
Kang, K., Meng, Y. S., Bréger, J., Grey, C. P., & Cedar, G. 2006. Electrodes with High Power and High Capacity for Rechargeable Lithium Batteries. Science. Vol. 311, pp. 977-980.
Ning, G., Haran, B. & Popov, B. N. 2002. Capacity fade study of lithium-ion batteries cycled at high discharge rates. Journal of Power Sources. Vol 117, pp. 160-169.
Pistoia, Gianfranco. 2016. Electric and Hybrid Vehicles: Power Sources, Models, Sustainability, Infrastructure and the Market, Amsterdam, North Holland: Elsevier.
Vincent, Colin A. 2000. Lithium batteries: a 50-year perspective, 1959–2009. Solid State Ionics. Vol. 134, pp. 159-167.
Wang, Q., Ping, P., Zhao, X., Chu, G., Sun, J. & Chen C. 2012. Thermal runaway caused fire and explosion of lithium ion battery. Journal of Power Sources. Vol. 208, pp. 210-224.
To be fair, it’s not a problem unique to batteries, any form of high density energy storage is basically trying to design a bomb that won’t go off, gas tanks, propane tanks, compressed air, tightly wound springs etc.
You’re mixing different classes of energy storage.
Oxidizer and fuel in the same package makes a bomb – the fuel alone is just fuel.
Technically you’re correct for 99.999999% of the universe…. However, since we are resident on a planet with ~20% atmospheric oxygen all around it, anything that reacts with oxygen is sufficiently bomblike under the right (wrong?) circumstances…. However, a quarter full tank of gas more closely meets your OCD preferred definition of bomb because the air and vapor mixture above the liquid is explosive, than does a 100% full tank of gas.
For most fuels the mixture would be entirely too rich to explode – wrong A/F ratio – or the fuel wouldn’t be volatile enough, such as diesel oil, or the tank is under positive pressure and air cannot get in, like with LPG.
It’s just practically very very difficult to make a fuel tank explode, or as you say “to design a bomb” of it.
Meanwhile it’s very easy to torch up an entire electric car in seconds by driving over a piece of scrap metal, as Tesla has proven. A lithium cell has all the components of a chemical fire, in the right proportions, in a convenient metal capsule, which when heated enough will explode.
Oh, lots of pressure stopping the air getting in makes it not a bomb?
Bombs, bombs, bombs, even diesel… https://www.osha.gov/pls/imis/AccidentSearch.search?acc_keyword=%22Diesel%20Fuel%20Tank%22&keyword_list=on
Next you’re going to tell me that mechanical damage or excess heat doesn’t count when it’s the very mechanism by which you assert a lithium battery is dangerous.
You’re shifting the goalposts.
For example, from the OSHA website.
>” an employee was welding a diesel fuel tank that had a small amount of diesel fuel still in it. During welding, the tank ex ploded and killed the employee.”
That’s not a tank quarter full of fuel like you originally said. Fuel tank explosions are exceptionally rare because they require very precise conditions to happen. If you put a welding stick to a quarter full tank of diesel, you will set it on flame, but no explosion.
>”Next you’re going to tell me that mechanical damage or excess heat doesn’t count ”
Crush a jerry can full of gasoline, and the gasoline spills out. Crush a lithium battery and it explodes. You’re just playing stupid at this point.
Interesting link to diesel fuel related fatalities. Sure, diesel has been around for a long time, but is there any equivalent list of fatalities caused by battery explosions/incidents (and to be fair to the web link, that would include being crushed by falling batteries)?
I love that Motorola released a phone with the model number 2600.
For the young’uns the number 2600 refers to the sound frequency that people can use to cheat telephone company for free long distance call.
Indeed, but you do need an original disc dial phone to do it
No, you just need an original and unsuspecting telephone company…
YES, nothing more than a small plastic whistle found in a box of Captain Crunch cereal, coupled with appropriately timed pulses, was everything needed to access LD networks. The REALLY talented could control the network using only their breath and lips. The telcos quickly moved control signals apart from the voice lines, and, poof! the hack was gone.
LiFePO4 seems nice, but as with anything, there are always the bad apples.. My e-bike LiFePO4 12-cell pack from BMSBATTERY lost its first cell after just one year, and seems like the rest will follow soon.
Those cell packs, are they readily available? I’m looking to convert a bike to an ebike using one of those BBS02 motor/controller kits and would like to use LiFePO4 cells
Check Julian Ilett’s channel on Youtube. He converted an eBike from Pb to LiFePo4 and posted some videos about that.
Same for me.
Got my lifepo4 when it was half the price of lipo.
Twice the volume and weight is definitely not worth it for an e-bike.
Failing, and unbalanced cells were the last straw.
Went back to 18650 cells. Happy as a camper.
Maybe for a powerwall, but it would be more economical to go for lead, or 2nd hand lipo.
Longevity must have some use case.
Especially in a powerwall longevity is an important feature. Lead-acid does not like deeper discharge cycles, starter batteries being the worst in this case, lead-acid deep cycle batteries are better, but far inferior to LiFePO4.
Can someone explain to me why a lithium battery designed specifically for a motorcycle is considered damaged beyond repair if it drops below 11vdc (even once)? Why can I take a lithium battery for a power drill and charge and drain to zero over and over. But the motorcycle battery is damage? Is it because a motorcycle needs 13.5vcd to crank the starter and when the lithium is drawn down below (even once) 11vdc it can never reach back to 13.5 charge?
Run your drill battery dead, then measure with meter, bet it’s not zero.
I bet it is actually zero….
Only if the drill has a properly designed BMS and has switched off the discharge MOSFET upon reaching the safe low-cell-voltage limit of it’s weakest cell.
Yah, I forgot that some ppl would take it as meaning to measure on the BMS side, where it presents cells as dead, whereas they’re gonna be sitting at the safe discharge volts the other side of it.
It’s strange that your ‘cycle battery went bad. The article discussed raw batteries / cells as typically used by RC or robotics. In commercial products – laptops, power tools – there is a battery control circuit that manages the charge and discharge rates. It also has a shutoff circuit to prevent the voltage from going too low. The replacement motorcycle batteries that I’ve seen have that management circuit. In part that is because the charge / discharge voltages and curves for a Li battery are different than for lead acid.
For a ton of information check the site http://batteryuniversity.com/
The difference may be the current handling capacity of the isolation device. A small battery (power drill) will have a FET that can handle the moderate number of amps required by the drill. A FET that can handle motorcycle starter-motor current will be expensive (and large) and as a result will probably not be fitted to the battery. A FET (or relay) that can handle the currents required of a car starter-motor will cost 30% to 50% of the price of the bare cell pack, hence those batteries are not only hard to find, but damn expensive.
A 30V/300A (continous) FET costs about 2€ at Digikey. even if you parallel 2 or 3 that is way below the expensive cells.
Yes, it turns out that this very article can explain that! FTA:
“It turns out that copper foil is generally used in the cells as a current collector. When the voltage drops below a certain threshold, some of the copper in the negative electrode starts to dissolve and migrate. When you then recharge the cell in question, it forms dendrites of elemental copper somewhere they shouldn’t be.
In a best-case scenario, this just decreases the ability of the cell to collect current. The internal resistance of the cell may go up, causing more heat to be generated during charge and discharge. In short, the C-rate is decreased.
In the worst case, this will form a short in the cell while it is charging, causing it to fail catastrophically (Arora, White, and Doyle 1998, p. 3658-3659). This may occur immediately, or some amount of time later.”
But is it possible that the cutoff voltage wasn’t actually met, but that the management circuit was not designed to reset after a low voltage event – one that didn’t go below the threshold? Kind of like how some older batteries management circuit stores have some volatile aspect and they need more than a mere cell transplant to work again.
Maybe a peak drop in voltage showed a set of parallel cells to appear to have failed and thus the battery (programmatically/logically) no longer feels safe to deliver the current.
After all, the designer may have accounted for a bad cell disconnecting due to high internal pressure and thus it is safer to not ride bombs around on the road.
As far as I know, it’s not even about “old” battery management systems – many new, “smart” BMS behave this way, once they go over a certain level, or some internal cutoff triggers, they won’t ever turn on again unless, presumably, fed a sequence of proprietary commands.
First, if you have a lithium battery, you’re not draining the battery to zero volts. You’re draining the cells from 4.2V each down to 3V and then the circuitry inside the battery shuts it down to prevent it from being damaged. Of course if your battery lacks that circuitry then there is nothing to prevent you from going below 3V per cell and damaging the battery.
Second, are you sure you’re talking about a lithium battery? Lithium cells have a nominal (average) voltage of 3.7V and a max voltage of 4.2V. Neither of these values gives 13.5V if you have 3 cells in series. You get 11.1V or 12.6V. And if you have 4 cells in series, you get 14.8V for the average voltage. A quick google indicates 13.5V is the fully charged voltage of a 12V lead acid battery.
Finally, this link has a discussion explaining why there’s no point in going below 11V on a lead acid because it’s almost dead by the time you reach that voltage, and how going too low could potentially damage it by causing polarity reversal on individual cells inside the battery making it impossible to charge:
[link removed because my post wasn’t appearing]
Google “how-bad-is-it-to-undervoltage-a-12-volt-lead-acid-battery” to read about it on Stackexchange.
A determined person might disassemble the lithium motorcycle battery, and check the voltage of each cell inside. Disassembling lithium cells can be dangerous, so be sure to take all necessary safety precautions.
Safely dispose of any cell whose voltage is below the cutoff. Then recharge any cell above the cutoff using a lithium cell charger.
These cells can be used in later projects. If you wish to actually repair the battery that’s more complicated, it depends if the motorbike has a cell balancer to charge the cells in the battery.
Personally I never repair batteries. I salvage the functional cells and use them in projects (or make new batteries out of them using only the same cell type).
My motorbike still uses a Pb battery unfortunately.
Disassembling batteries is kind of fine, but I would advice against disassembling the cells, it is also rather useless to do so.
Disassembling the cells is useful when you want to make ice…
When I want to make ice I need a functioning battery to deliver enough current to run the freezer.
If you are talking about organic chemistry you will probably not be happy with disassembled Lithium Ion cells, as they don’t contain metallic Li.
Color me embarrassed.
While certainly still a true statement, I meant to say ‘Disassembling lithium batteries can be dangerous, so be sure to take all necessary safety precautions.’
I suppose the only reason you would want to disassemble a lithium cell is if you were stranded and needed to start a fire (and even this would only work in certain conditions). Nonetheless, it’s amusing to imagine a post-apocalyptic future where desperate survivors start a fire with a Samsung phone.
No, a typical starter needs >6V – a typical lead acid battery in freezing conditions can drop that low (cold cranking). The power drill hopefully switches off, before a deep discharge state is reached, at least my cheap ones (discount-supermarket grade) do this.
11V would be 2,75V/cell in a 4S LiFePO4 setup. I did not expect this quite high deep discharge threshold. It is definitely possible to bring the voltage up again, but obviously this is considered dangerous.
EDIT: The 100Ah LiFePO4 battery I bought some days ago is specified from 10V to 14,4V. Another battery from the same shop with 90Ah, specially designed as starter battery and not to be used as storage battery (why?) states 10V to 16V and is supposed to be a “LiFeYPO4” technology. Never heard before.
The voltage required to start the bike is higher than the rated nominal output voltage of the battery. If the battery is too low, starting the bike would draw so much current that the battery will actually start to heat up (internal resistance at work) to the point that gases will form that can, under pressure, explode because of the presence of fuel, heat, and air (with an electric spark to kick off the reaction) mixture. As such, your motercycle’s battery management sysyem will break the circuit to prevent that once it hits a certain voltage level.
This is the one thing holding wearable electronics back. Batteries exist that are cheap enough and small enough, but they aren’t safe enough to sew into clothing.
The potential danger depends on the energy capacity of the cell. And phone batteries are considered safe enough for people to keep in their breast or trouser pockets, when in a phone. Besides that, on a commercial scale safety is a statistical thing. It only has to be relatively safe compared to all the other dangers people live with.
A plastic case is safe enough for a phone’s battery, should be fine for whatever wearable gizmo.
That’s the one thing?
Would the batteries survive a wash – dry cycle?
That’s a good question and I thought about the same thing.
On one hand, you could seal everything and put it through a lot of testing. On the other, you could make the lithium cell removable, which also makes your clothing easier to repair, doesn’t tie you to the manufacturer’s battery, and means you have the option of swapping in a fresh battery when one runs dry.
I’ll now fail to avoid the joke about wet cells and dry cells with regards to washers and driers.
holy crap. a blogger that knows how to footnote and provides references. I like it.
This isn’t a blog, it’s more of a publication. A commercial enterprise. I dunno what their legal or scientific obligations are, but when you’re aiming a site at geeks your reputation can die easily based on accuracy. OTOH geeks are responsible, as a whole, for a lot of money getting spent, so there’s a place for places like this.
Thanks!
I didn’t find the details I was looking for through general research… most sources stated the rules for using lithium cells safely but didn’t go into gritty details (which is what I was curious about!). So I ended up down quite a rabbit hole of journal articles.
In addition to giving them credit for their research, I found a lot of it interesting to read, so thought it would be nice to share. All the articles I used can be accessed without going through paywalls (google’s filetype:pdf is your friend here) The sheer volume of research that goes into lithium batteries is astounding!
There currently are cells on the market that CAN go to almost 0V and survive (different electrode material)… Also, LiCo cells still remain kings in capacity, which can easily offset LiFePO4 advantages by simply not cycling the cells so hard. Tesla chose them for a reason.
Tesla actually uses a proprietary chemistry that is more or less lithium cobalt tweaked to be a lot less flammable. It is not the same as what caused the infamous 787 fiasco.
Tesla uses a tweaked version of Panasonic’s NCA cells. (Nickel Cobalt Aluminium)
https://qz.com/488596/teslas-coattails-are-carrying-along-panasonic-but-a-battle-for-battery-supremacy-is-brewing/
It’s not “a lot less flammable”, it’s very much flammable, and it has a thermal runaway point as low as 155 C. The safer version is NMC that replaces aluminium with manganese, but Tesla doesn’t use it for cars because it costs over twice as much per kWh.
And also because NCA has the highest specific energy of the different types. The battery upgrades in Model S for example have come about because Tesla has managed to fit better cells in the pack. With NMC cells, they’d run into mass and size limitations and couldn’t provide the same range.
Apple built some Macbook models with a pair of flaps in the top of the battery housing, covered with tape. Those flaps are directly below the trackpad. When the battery puffs up, it breaks the trackpad.
They could as easily have designed a ‘blowout’ panel on the bottom of the laptop, with screws holding it from the inside of the bottom cover.
Worse than their designed in trackpad destruction is they also put something in the batteries for recognition. If you don’t replace it with a genuine Apple battery or one that the vendor has successfully cloned the recognition (Other World Computing) the Macbook will only run the CPU at its slowest speed.
Yep that’s my MacBook your talking about – it actually bent the trackpad and cracked the screen and pushed the bottom casing out.
Replace the battery pack, bend the track pad back and live with the crack across the top of the screen
1. You have abused the word science.
2. “Cylindrical lithium cells such as the 18650 are more likely to contain this type of circuit than pouch cells.”
Is False. Unless they are “26650” cells which are physically a different size, the jelly-roll cells do not use a circuit at all, they use an electromechanical as a thermal fuse / current limit fuse. It looks like a small disc. Some large 18650 battery arrays use large sheets of these fuses so that each battery in a parallelled bank is individually fused. If you are buying a battery shipped into the US or most countries, it will likely have to comply with UN DOT 38.4 testing.
3. “we will explore today is the reasons behind the characteristics of lithium cells and the rules that govern their safe use.”
But this article does not mention any of the current safety standards or their requirements like UL1642, UL2054 or EN/CSA/IEC/UL62133
Here’s a link:
https://www.ntsb.gov/news/events/Documents/2013_Lithium_Batteries_FRM-Panel2a-Florence.pdf
Here’s another:
http://www.metlabs.com/battery/top-3-standards-for-lithium-battery-safety-testing/
4. “Undervoltage: We’re told not to let the voltage of lithium cells drop below a given voltage (varies by cell type but often around 3 volts). This is unfortunate because we’re often ‘gifted’ dead lithium cells registering a low voltage that would be nice to use again, for example in a new flashlight. Even though they may charge again, it’s not a good idea to do so.”
We’re told is not a scientific reference. Battery chargers for lithium packs can recover deeply discharged cells by pre-charging the batteries up to a low voltage limit. After that threshold is reached, regular 3 stage lithium battery charging can occur (constant current, constant voltage, 90% burp charging.)
5. “This is why any cells you receive by mail might be only partially charged.”
No, they are partially charged because lithium cells begin to self-degrade the day they are manufactured. The self-degredation presents itself as a permanently lower usable capacity. The effect is worse if the battery is stored at high temperture, the effect is significantly worse if the battery is stored at full-charge. The effect is minimized if the battery is stored at 50 – 60% charge.
6. “Manufacturing defects: With the number of things that can go wrong with lithium cells, quality control is critical. For example, one thing reputable companies test for is metal contaminants in between the cell membranes that can cause shorts:”
Reputable battery companies require each and every cell to undergo several charge and discharge cycles to weed out units that have internal shorts or other manufacturing defects.
7. You may want to add what the different commercially available lithium cell chemistries are, their advantages / disadvantages and what their intended target applications are, maybe add them in a chart or table that you add to all Hackaday articles on Lithium batteries for a technical reference.
Why would you want to use Lithium Chloride cells? Why are 18650 cells in a cylinder shape? How are cells balanced in series or parallel battery management systems and why? Why do cell phone battery packs use mostly parallel cells whereas battery operated lawn mowers use series cells, why would you choose one over the other? What are the safety implications of each? What does NASA have to say about 18650 cells being used in large battery arrays that have multiple series and parallel banks of cells?
Thank you for sharing those links about safety standards. While our position may differ on several of the items you mention, I found them an interesting read.
As you say, there are many lithium cell chemistries on the market, and an investigation on which chemistry is best for what purpose would be quite useful! If you wrote something like that up, I would be excited to read it.
I found this article that provided a summary of some different chemistries which you may enjoy (although you may already be familiar this particular website):
http://batteryuniversity.com/learn/article/types_of_lithium_ion
However while reading the primary literature I saw that there were in fact many more chemistries than that. In fact, one of the biggest challenges in this article was trying to make statements that applied as generally as possible across chemistries!
The ‘lower charge % for shipping’ was one of those things. It was listed in some articles as a method to make thermal runaway less likely during shipping, but what you’ve brought up sounds like a perfectly valid (perhaps better) reason not to ship them fully charged as well!
And what about the Samsung Galaxy phones who’s self destruct function was active practically from the beginning? Did they figure that one out yet?
I think it was an additive tolerance/defect thing. Slightly off battery in good phone, no problem, good battery in good phone no problem, slightly off battery in slightly off phone, fireworks.
Been using LiFePO₄ cells to power the lights and radio on my bike for years now. They’ve never given me trouble.
LiPol I use with great caution… as they tend to be a lot less forgiving. Basically the only LiPol batteries I have are commercially made packs built for consumer devices, and they are used with those consumer devices only.
Even then, I am reminded of the battery from the laptop I am typing on now. This 2008-era MacBook has had to have its second battery removed because the battery became bloated like a puffer fish. The same happened to the original battery a few years ago. Never had that in any other laptop I’ve owned or used, and I’ve used quite a few over the years. I’ve just decided to put up with a laptop with literally no battery (leading to interesting problems with the RTC when I disconnect from power).
I’ve been working on some (hopefully “clever” ;)) projects using LiFePO₄ for a while now (see https://hackaday.io/xorbit). Thanks for the nice writeup on Lithium cells!