Back in the old days, finding out your location on Earth was a pretty involved endeavor. You had to look at stars, use fancy gimballed equipment to track your motion, or simply be able to track your steps really really well. Eventually, GPS would come along and make all that a bit redundant for a lot of use cases. That was all well and good, until it started getting jammed all over the place to frustrate militaries using super-accurate satellite-guided weapons.
Today, there’s a great desire for more accurate navigational methods that don’t require outside communications that can easily be jammed. High-tech gyroscopes have long been a big part of that effort, allowing the construction of inertial navigation systems with greater accuracy than ever before.
Why Inertial Measurement?
The fact is that GPS and other satellite navigation systems can no longer be relied upon in contested battlefields, and even in peaceful areas at times. The technology to jam or spoof these navigational systems is now readily accessible, to both state actors and even the well-resourced individual.
Thus, there is a great desire for navigational systems that are impervious to jamming. An inertial navigation system (INS) is just that. The concept is simple. From a known starting position, an INS measures acceleration and rotation of a given vehicle or projectile. From these measurements, it’s possible to calculate position, orientation, and velocity over time via dead reckoning. In a simplistic example, if you start at your house, quickly accelerate to 2 meters per second, and run in a straight line for a certain length of time, you could determine your position relatively easily. An INS does exactly that, but in three dimensions, and really really accurately.
The problem with the dead reckoning approach is that errors are cumulative. If you get your speed measurement slightly wrong, for example, your estimated current position will rapidly become more inaccurate over time.
Thus, better measurements create a far more accurate fix that’s usable for longer without needing to check in with an external reference like GPS. Hence the interest in high-performance gyroscopes that can measure more accurately with less drift over time. The latest technologies eschew the spinning rotors and fancy mechanical contrivances of the past for altogether wilder approaches.
Ring Laser Gyroscopes
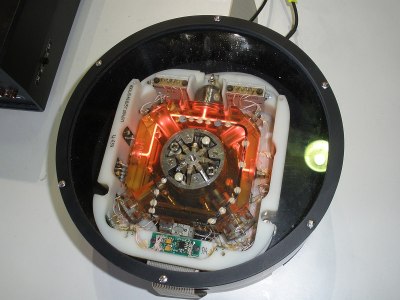
Ring laser gyros, or RLGs, use a closed ring of light to measure angular acceleration. At the heart of the RLG is a triangular or square-shaped optical path, formed by mirrors that allow light to travel in a closed loop. Within this loop, two beams of light, typically from a single laser source, travel in opposite directions. Under stationary conditions, both beams of light take an equal amount of time to complete a loop, resulting in no observable phase difference between them.
However, things change when the gyroscope experiences rotation, thanks to the Sagnac effect. When the RLG is subjected to rotation, the path length for the beam of light traveling in the direction of rotation effectively becomes longer. In turn, the path for the opposite beam becomes shorter. This difference in path lengths causes the two beams to experience a shift in their relative phase. As the beams complete their paths and reconverge, they interfere with each other. The phase difference manifests as an interference pattern. By measuring this pattern, one can calculate the angular velocity experienced by the gyro.
It’s worth noting that RLGs are remarkably sensitive, capable of detecting minute changes in angular velocity. This sensitivity arises from the fact that light travels at an incredibly high speed, and even small rotational motions can induce detectable phase shifts in the light beams. Moreover, the solid-state nature of RLGs, devoid of moving mechanical components, grants them a robustness that is especially valuable in applications where durability and reliability are paramount. From aviation to satellite navigation, RLGs have found their niche, ensuring precise rotational measurements even in the most demanding environments.
RLGs can be a little fussy though, particularly when it comes to detecting low rates of rotation. At these levels, the two laser beams moving in opposite directions can “lock-in” to each other, hiding any phase difference. To avoid this from happening, RLGs are often subject to “forced dithering”, where the laser cavity is actually rapidly rotated back and forth, usually around 400 Hz. This keeps the system’s angular velocity outside of the lock-in range. To avoid lock-in occurring at the extremes of motion, where the gyro is changing direction, white noise is often introduced into the 400Hz rotations.
RLG-based navigation systems have become popular in commercial aviation as well as in military contexts. Typical accuracy can be on the order of one nautical mile of error per hour of operation. Larger systems, or systems that stack up additional gyros to compare measurements can improve performance further.
Fiber-Laser Gyroscopes
A fiber-optic gyroscope, or FOG, works using the Sagnac effect, just like a ring-laser gyro. However, instead of a using a small rigid cavity as in a ring laser gyro , a long length of looped fiber-optic cable is used as the cavity. Two beams from the same laser are injected into the fiber in opposite directions. Their interference pattern, as impacted by the rotation of the gyro assembly, is then measured in the same way as the ring laser gyroscope.
The benefit of the FOG is that the impact of the Sagnac effect is effectively increased by a multiple of the number of loops of fiber optic cable. This is because the laser’s path is much longer, by virtue of passing through a long coiled-up fiber optic cable. The longer path length means the path difference is also greater between the two interfering beams, giving the device a greater potential resolution.
Thanks to the capability to pack a very long laser cavity into a compact space, practical fiber-optic gyroscopes can be built with excellent performance in a compact space. Large marine examples tuned for optimum performance can offer accuracy on the order of 1 nautical mile over 360 hours of operation.
Hemispherical Resonator Gyroscopes
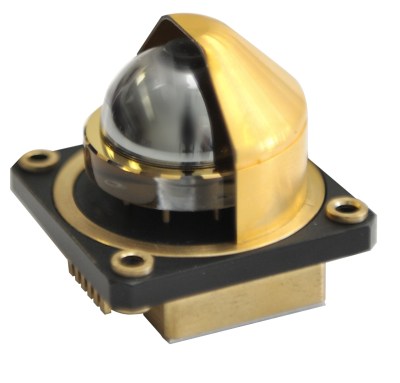
Hemispherical resonator gyroscopes, or HRGs, use mechanical resonance as the basis of their operation. They offer benefits over traditional mechanical gyros, as well as laser types, in that they have drastically reduced Size, Weight, and Power (SWAP) requirements. They make it possible to create highly accurate intertial navigation systems in compact, portable packages.
At the core of the HRG is a thin, hemispherical shell, typically crafted from quartz. This shell is anchored at its base and can resonate, or vibrate, in a specific mode when appropriately excited. Just as a wine glass produces a distinctive tone when flicked, the hemispherical shell of the HRG resonates at a particular frequency when driven by external actuators.
The shell must be delicately tuned for peak performance via techniques like ion beam erosion or laser ablation, much in the same way that quartz radio crystals are tuned to a specific frequency. The resonator is then coated with an ultra-thin metallic film. To initiate the resonance, electrostatic actuators are strategically placed in the vicinity of the resonator. Some designs use a hemispherical electrode within the hemispherical resonator, but more compact and cheaper designs can be achieved with simpler flat electrodes located on the equatorial plane of the resonator. Once activated, these actuators cause the hemispherical shell to vibrate in its resonant mode.
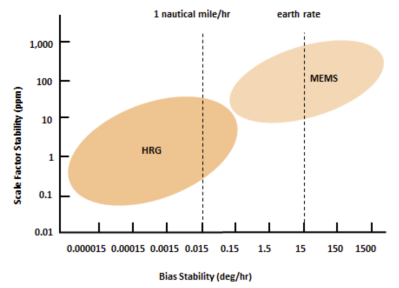
Now, when the HRG experiences an angular motion, the Coriolis force comes into play. As the HRG itself rotates, the pattern of the vibration rotates too, but not by as much. The shifted vibration pattern can be picked up by the gyro’s electrostatic sensors, which convert them back into electrical signals. By analyzing these signals, the angular velocity of the HRG can be accurately determined.
HRGs have been used in a wide variety of contexts, from spacecraft to commercial aircraft and in guided weapons. Their compact size means they are well-suited to more compact mobile platforms. Peak performance figures are, to a degree, classified secrets, but they are orders of magnitude better than popular MEMS gyroscopes commonly used in consumer hardware.
Conclusion
If you’re eager to design a navigational system that can operate in areas where satellite communications are denied, you’ll likely find yourself exploring these advanced gyroscope technologies. Whether you’re tangling with congested radio spectrum, or operating underground or underwater, these advanced gyros are key to achieving the minimum in drift over time. They don’t come cheap, and they’re certainly not easy to build, but these devices have become staples in the world of high-end inertial navigation devices.
It would have been great if you also referred to future directions – e.g. Quantum Sensors https://www.advancednavigation.com/tech-articles/the-future-of-inertial-navigation-is-classical-quantum-sensor-fusion , as I recently found this topic an interesting read (whether it is correct or not is not my domain of expertise)
What’s interesting is that the “classical sensors” that the linked document describes actually rely on quantum mechanic principles involving the photon. RLGs and FOGs both rely on the interference pattern caused by the phase difference of photons. Also, given that this quantum sensor involving atoms has such a small bandwidth; it seems more practical to have multiple FOGs of different lengths and composite their measurements for both precision and range, than to hybridize the atomic quantum sensor with a FOG.
Interesting. It looks like the Soviets copied the Litton ring laser gyro design. The one in the photo looks almost identical to the ones I worked with in the ’80s. They are amazing technology. The mirrors in the corners have to be 99.99+% reflective. (I didn’t have the clearance to find out how more nines in the number)
You might have worked with my dad there. How funny!
I have talked to some people in Russia regarding civil aviation INS in the past. What i was told is that Soviet / Russians never had laser gyros good enough for INS in an aircraft. Most of their tech was only good for missiles, where the drift in time can be larger as the predicted flight time (and life time for that matter) is way shorter. I don’t know about this particular example from Ukraine, but what i was told that they tried to copy Litton / Honeywell, but they never got to comparable drift with it.
I was there also, in the gimballed mechanical gyro lab. We used an 8X300 microcontroller to PWM the motors that kept the gyro platform orientation constant.
The 8×300 wasn’t so micro and ran hot enough to fry an egg.
It might not be a hack in the strictest sense of the word, but I find it quite impressive that someone managed to make a quartz dome detect rotation.
And then there’s the quantum gyroscope which you covered.
https://hackaday.com/2023/06/07/royal-navy-tests-quantum-navigation/
They still need to be “stabilized” and given an origin(via GPS or a known “calibration box”, takes about ten minutes. And drift is about 1 mile per thousand, which is pretty good considering they are accounting for latitude, the rotation of the Earth, the Earth going around the sun to a point, and all the moments of inertia associated with moving a vehicle. Amazing technology.
But how well will it work in guided ordinance, you know, shot out of a gun?
1 mile per thousand isn’t great for car navigation, let alone a cruise missile.
Surely over land optical guidance would be far more effective, and is now trivial with modern image processing?
For mechanical gyros/accelerometers, better accuracy is available through superior design, superb machining, extensive calibration, accurate (and secret) gravity maps, and all sorts of fiddly bits. If the system doesn’t have to handle high acceleration, more accuracy is possible.
Just as a sidenote.
For a long time, dead reckoning was the to-go-methode for navigation on boats. In the large scale, determining longitude was a problem (until the invention of precise clocks). In the small scale it was difficult within poor visibility (fog, nighttime) to get a good position. Then they relied on devices like ship logs.
Often ships go in formation and it was a not so rare occurrence that the summed up errors by leading ship brought the following boats into the same distress.
18 February 1942: USS Wilkes (DD-441, leading ship) grounded, USS Pollux (AKS-2) and USS Truxtun (DD-229) sunk, 203 lost souls.
Yep, my class (2007) at the Academy was the last year to have a full 4 years of Celestial Navigation. That stuff was *hard* and it is amazing old timey sailors used it so effectively.
The one thing I will say about it, is that accuracy with positioning doesn’t matter as much on the open ocean, and when close to shore the amount of time needed to do Cel Nav and the lack of accuracy isn’t worth it. Instead within visual range of land it is easier and quicker to take 3 simultaneous visual bearing lines to charted (and visible) objects on shore. You then put those lines at the correct angles on the chart and where they intersect is your position.
RDF uses similar methods.
“The latest technologies…” makes it sound like RLGs are a new thing, when in fact they predate GPS by years. An early working RLG was demonstrated in 1963. Ten years later (1973), work BEGAN on GPS. Twenty years after that (1993), the GPS constellation was complete.
The vulnerabilities with using GPS positioning by the military have long been understood. I worked on the navigation system of the Trident missile over 20 years ago. The Trident missiles would receive their destination coordinates while still on the subs, then when launched, they would use mechanical gyroscopes and optical star positioning to provide position corrections, completely autonomous.
That’s fine when you are >35,000 feet, you have access to the stars.
I don’t really want to be the party pooper, but I was really expecting from the headline we’d approached an INS error of inches/centimeters over tens of thousands of miles/km. I’m not impressed. Call me when we can circumnavigate the Earth underwater without radio systems and arrive back within inches of the planned destination.
Submarine grade systems are pretty accurate, but details are classified. I worked for a company that made inertial nav systems and never heard any details about submarine systems and only vague accuracy claims for the aircraft ones.
Yeah what you are describing could only be a cutting-edge weapons guidance system and would be classified
Luxury! I was expecting 3d printable gyros that could fit on an ant so that ant circumnavigation of the Earth would be down to millimeters.
I’m sure someone will let you know when we break the laws of physics.
Or use them.
https://www.ion.org/publications/abstract.cfm?articleID=17701
I can recall , when I was interviewed at the USAF Academy in the late-70s, the tour guide bragging the F-15 (?) could fly across the United States simply by using some sort of INS and land within a few feet of the destination coordinates. Fact or not, I have no idea.
Personally I’m a fan of the Astroinertial Navigation System on the SR-71. Obviously you could combine this technique with new gyroscopes and get something more reliable. Having to be at altitude does limit its scope however.
(Sure, on a clear day/night it might work fine in the middle of the ocean too)
I’m clueless, as usual.
But.
I assume that “the military” has spend lotsa sums of money into terrain contour maps for matching each and every millimeter (that is 0,0393701 of an inch) of our earth.
A submarine “sees” sound reflections from the coast and (within limits) the seabed. Can someone enlighten us how good that works for a submarine?
Let me start by saying most of my submarine combat knowledge comes from spy thrillers and movies, so if anyone has more experience they should absolutely be trusted over anything I’m about to say. So, there’s how big a grain of salt to take the rest with….
Stealth is why you wouldn’t want to do that. Making a local map of the seabed to compare to stored ones requires active sonar pinging (or emissions of some kind) and assumes you’re not in water too deep (or on the wrong side of a thermocline) to effectively bounce a signal off the seabed with enough strength to get an accurate image. The military isn’t going to want anyone with a hydrophone to be able to go “oh there’s a submarine sneaking around here pinging the hell out of everything”.
Even in peace time operations I’d imagine that wouldn’t be ideal because it’s the equivalent of navigating a mall by screaming at the top of your lungs and listening to the echo & once you’ve got a couple people doing that anywhere near each other, nobody’s going to be able to tell where they are at (On top of concerns about active sonar disrupting ocean life which I’m aware of but totally unqualified to do more than make a passing reference to).
There’s a whole US government agency, DMAHC(Defense Mapping Agency Hydrographic Center) that does undersea mapping and services the Navy and Coast Guard. I believe it is attached to the NGA, and the DMA is a sub-section further divided for different tasks.
This agency generates charts used by the Navy and Coast Guard and eventually trickles down to the public in the CG’s Marine Chart products. It was the failure of this agency in providing accurate charts that in part led to the USS Connecticut collision a few years ago as well as the USS San Fransisco a few decades ago.
We could often get a star lock on a similar system in Los Angeles on a summer day.
My father in law worked on these when he was at Honeywell back in th’ day. Very cool stuff.
https://aerospace.honeywell.com/us/en/about-us/blogs/the-still-amazing-honeywell-ring-laser-gyroscope?utm_source=google&utm_medium=cpc&utm_campaign=23-aero-ww-dsa-blogs&utm_content=dyn-en-lp&gad_source=1&gclid=CjwKCAjw7oeqBhBwEiwALyHLM33a6NqKaw6kBAPGfY-cEH3CsDGmX5xfqCwAuMUVeGN6p87bRZb3qBoCM5IQAvD_BwE
Fascinating article! It strikes me as somewhat puzzling since, as far as I understand, a Gyroscope typically measures changes in angle over time, while an Accelerometer tracks changes in velocity. However, navigation systems primarily deal with changes in position or displacement, rather than just angle alterations. Furthermore, I’ve noticed that various error estimates for different gyroscopes are provided in Miles per hour, which represents deviation in position over time. This begs the question of how a device designed for angular measurements can yield accurate positional data. It’s apparent that there might be some missing context or knowledge on my part. Could you please shed some light on whether my interpretation of the article is off or if there’s some preliminary information I need to grasp to make sense of it? Your insights would be greatly appreciated.
My try on that would be: a laser gyro measures the time (better: the time difference between two light beams). There is a direct relation between time and distance, dictated by the constant speed of light.
With the knowledge of the time measurement error and the summed up error over time we know the distance error over time.
I was wondering the same- I guess the bit we are missing is that there must also be three linear accelerometers to tell the system which XYZ direction the platform is accelerating in. They gyro tells the system if the platform has rotated (so the XYZ is now oriented in a different direction). Cue an article on linear accelerometer tech?
You’re absolutely right. It makes no sense to characterize gyro performance in distance deviation. What’s happening here is that someone is misquoting the performance of an INS system, not a gyroscope. An INS system combines a gyroscope with other sensors, typically an accelerometer, and potentially GNSS as well. Those systems provide a total, 6 DOF navigation state, including the rotational state (provided by the gyroscope output), as well as the translational state (usually driven by accelerometer errors, but with a small component of error from gyroscope (you need to rotate the accelerometer measurements based on the rotation of the vehicle)). So the quoted performances are missing the implied accelerometer accuracy, which probably contributes the most to the performance. Replace one accelerometer with a better one, and you’ll get better nmi/hr, with the same gyro.
There are also Radio alternatives beside GPS or more general GNSS like Loran-C or R-Mode (https://www.r-mode-baltic.eu/publications/)
Loran is highly inaccurate. Also Loran-C operations ceased in 2010 when I was still stationed on a ship.
Russia still uses a version of Loran
They set up 3 transmitters around Ukraine before the 3-day war, err, special operation.
Doesn’t seem right to use the word ‘staioned’ for a moving object.
Is there an alternative?
You’re overthinking it. Does the instrument cluster not give the “state” of your car’s engine while you drive down the road? It’s the same root word.
Why is some paper called stationery, when it is intended to be mailed?
B^)
Very cool summary article. As others have mentioned I guess the accuracy of one mile drift per hour can be either fantastic or woefully inadequate.
Instead of GPS RF signals I’d imagine a very similar system but instead of broadcast radio waves it would function with direct pointing of lasers from satellites to a designated (friendly) vessel. Spoofing would be harder. But it would be subject to atmospheric problems I guess. For above the atmosphere (missile) use would be fine or the current method of a ground unit (or satellite with laser designator) can spot up the target and the warhead navigate itself by whatever means till it’s close enough to lock on the laser.
“This sensitivity arises from the fact that light travels at an incredibly high speed”
That is the opposite of the truth. If light travels faster, for any given frequency of light the wavelength would be longer, thus the phase shift for any amount of rotation would be less.
So if your fiber optic INS fails, you could end up “lost in the fog; without a FOG”.
B^)
Nit: The RLGs (and most gyros) can actually directly measure angular velocity, not angular acceleration.
Ï would assume so also. And that acceleration is just a derivation of this measurment. Like a reverse of how acceleration sensors are used to derive velocity in phones and GPS devices (in tunnels and other places wihout satelite covverage)
At a guess, measuring things like the magnetic and gravitational fields would help keep these stable long-term if I were to challenge you to know an object’s rough locale after a year inside a wooden crate instead of more precisely after a number of hours.
I believe if you were trying for accuracy, you could find almost your exact magnetic field vector direction in three dimensions with affordable small sensors. Local objects might disturb that, or some of the finer points of geomagnetism I’m not experienced with. But the coarse shape of the earth’s magnetic field is known on an ongoing basis and while it changes from year to year, it doesn’t accelerate that quickly, so it’s still quite stable if there’s not some specific disturbance. By comparing your direction to the global maps, you can figure out that if the field is plunging into the earth at such-and-such angle, you’re probably in one of a few country-sized regions. If your accelerometer is good enough, I think you can use the oblateness of the earth to gain slight info about latitude as well. Maybe there’s some space maps with more precision on that since it disturbs orbits gradually. But mostly, with your gyroscopes that can track so well, the 15 degrees per hour rotation of the earth is going to be easy to pick up. You can therefore also find true north is very definitively. And a gyroscope should be able to actually get your latitude better than each of those by checking how precession proceeds – you make a circle around the axis but that circle is only perpendicular to gravity at the equator, and varies towards the poles. A Foucalt pendulum as you may find in large buildings would on its own allow you to infer the latitude by simple calculation based on its precession rate, but a gyroscope is a lot more convenient to carry. Then once you have a confident latitude, and you can directly tell what the current declination is, you also have a half-decent longitude even without actually detecting the sun from inside the box. But non-visual-light means of detecting the sun may add to your total knowledge of probable latitude. Stuff like radio effects or maybe even cosmic rays?
Most of that isn’t going to give you the kind of pinpoint precision these systems start out with, or won’t work if you’re moving, but should be more stable long-term and with the addition of context clues like “I’m on land at 14000 ft and I have a barometric altimeter and a global topographic map with a list of mountains”, you could really begin ruling out quite a bit of long term system drift if your algorithm knew how to factor in that information, I think.
the Earths’s magnetic and gravitational properties are not exactely steady things, the magnetic poles travel; around, and even flip sometimes and the metal core swirls and the moon pulls things left and right and up and down.
The things that are more stable are the position of various stars and, if you can pole them, some airport beacons maybe.
Even mountains move due to continental drift and can’t be used.
Well in a variable length of time that’s a bit less than 13000 years, the axis will precess about 47 degrees or so. The magnetic poles will wander but usually take much longer than that to fully flip. I’d be amazed if an airport outlasts a continent or the majority of mountains.
Anyway for shorter scales of a year or so, and specifically disallowing manmade inputs like GPS or radio beacons, the magnetic field is not *that* bad.