Although the concept of nuclear fission is a simple and straightforward one, the many choices for fuel types, fuel design, reactor configurations, coolant types, neutron moderator or reflector types, etc. make that nuclear fission reactors have blossomed into a wide range of reactor designs, each with their own advantages and disadvantages. The story of the pebble bed reactor (PBR) is among the most interesting here, with its development winding its way from the US Manhattan Project over the Atlantic to Germany’s nuclear power industry during the 1960s, before finding a welcoming home in China’s rapidly growing nuclear power industry.
As a reactor design, PBRs do not use fuel rods like most other nuclear reactors, but rather spherical fuel elements (‘pebbles’) that are inserted at the top of the reactor vessel and extracted at the bottom, allowing for continuous refueling, while helium acts as coolant. With a strong negative temperature coefficient, the design should be extremely safe, while providing high-temperature steam that can be used for applications that otherwise require a coal boiler or gas turbine.
With China recently having put its twin-PBR HTR-PM plant into commercial operation, why is it that it was not the US, Germany or South Africa to first commercialize PBRs, but relative newcomer China?
Flow Of The Pebbles
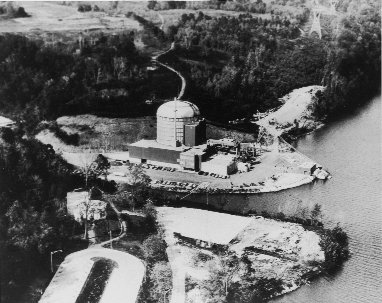
The idea of a high-temperature, gas-cooled reactor and the PBR in particular was first coined by Farrington Daniels, a physical chemist by training, which led to the first of such a unit being built in the US as Unit 1 of the Peach Bottom nuclear power plant. With the concept of the High-Temperature Gas-cooled Reactor (HTGR) taking hold, the UK built a somewhat similar design in the form of the Dragon reactor, though the Peach Bottom unit was the first to produce electricity.
All of these HTGRs have in common that they use graphite as the neutron moderator, with helium as the coolant. Graphite is highly temperature resistant, and helium is an inert gas that will not undergo chemical reactions with other materials or substances. Additionally, helium is not susceptible to neutron capture and will thus not become radioactive over time. These operating parameters is where the ‘high-temperature’ part of the design comes from, as the reactor core can run at much higher temperatures.
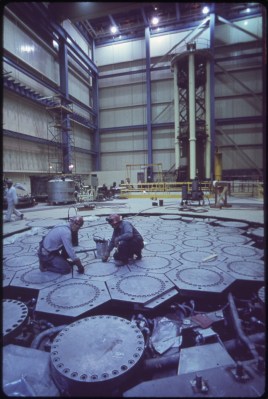
Whereas a typical pressurized light-water reactor (PWR) will have a reactor coolant inlet temperature of around 290°C and an outlet temperature of below 350°C, an HTGR’s inlet temperature is around 250°C, with around 750°C on the outlet. This puts it at or above the level of a standard coal-fired thermal plant’s steam temperature, and strongly increases its thermal efficiency over that of light- and heavy-water reactors. The US’s second HTGR (Fort Saint Vrain, in Colorado) was operational from 1979 to 1989 (one year past Peach Bottom Unit 1), but it being the first-of-a-kind (FOAK) of a commercial HTGR meant that many design issues were being ironed out during commercial operation, which eventually played a big role in its shutdown, and ultimately its conversion to a natural gas plant by 1996, as the US continued its general turn back to fossil fuels from nuclear power.
Beyond these two HTGRs, the UHTREX experimental reactor (1959 – 1971) at Los Alamos National Laboratory was also constructed, but used for testing fuel types rather than electricity production. This testing included direct cooling of the fuel without cladding, which enabled much higher burn-up as the fission products were able to move into the coolant flow.
With the shutdown of Fort Saint Vrain’s HTGR, the era of this reactor type in North America came to an end.
German Engineering Chops
Although the US and UK HTGRs used essentially fuel rods not unlike their water-cooled brethren, in Germany Rudolf Schulten – a professor at RWTH Aachen University – took it upon himself to develop a version using spherical fuel elements. This led to the world’s first PBR being built in the form of the Arbeitsgemeinschaft Versuchsreaktor, or AVR. As the world’s first ever PBR prototype, it suffered a number of issues after its commissioning in 1967, like helium leakage. Other issues were discovered after its shutdown in 1967, in the form of pebbles stuck in the bottom graphite neutron reflector, and a fine graphite dust contaminated with fission products, the latter of which was traced down to the high reactor temperature of 950°C not working well with the bi-isotropic (BISO, two layers of pyrocarbon) coated fuel particles that caused contamination with radioactive isotopes.
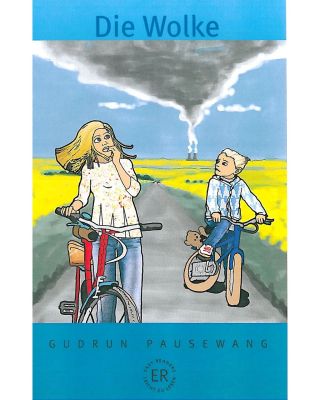
Years later, the prototype THTR-300 PBR was constructed, with commissioning in 1985. This used many of the lessons learned from the AVR, such as running the reactor at a lower temperature for an outlet temperature of 750°C and using TRISO (tri-isotropic with silicon carbon layer) coated fuel particles that are good up to about 1800°C. This reactor was however shut down in 1988 due to the growing anti-nuclear sentiment in Germany, which also affected what would have been the next step in the form of the modular ‘HTR-Module‘ (PDF) design developed by Siemens/Interatom. This modular design involves multiple reactor cores that are connected to a single steam generator, which eases scaling of the reactor total power.
When the HTR-Module project looked to be non-viable in Germany due to the societal resistance, a partnership with countries like China was established, with the Chinese HTR-10 PBR starting construction in 1995. Much of the mainstream resistance against nuclear power in Germany can be traced back to the 1986 disaster at the Chornobyl nuclear power plant (ChNPP), which led to fear-filled writings like ‘Die Wolke‘ (The Cloud, translated as ‘Fall-Out’), which is a book that became required reading for Germany’s youth in which the effects of even a Chernobyl-style disaster are massively exaggerated.
The irony is perhaps that in a country which adores soaking in radioactive radon spas and radioactive water, the effects of the ChNPP disaster would be thus exaggerated, despite this disaster causing a few dozen deaths for those near the destroyed RBMK reactor core, no appreciable increase in cancers and no genetic effects being passed down to one’s offspring. Yet because fear speaks louder than evidence, Germany’s PBR technology died in Europe, met resistance in South Africa and found a warm embrace in China, a nation which saw itself faced with a rapidly growing population and associated power demands.
Enter The Dragon
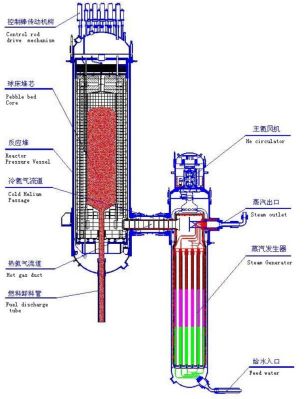
Nuclear power has been a core tenet of China’s energy policy since the 20th century, with HTGR designs being one of the many puzzle pieces needed to make things work. Alongside many foreign light- and heavy-water designs ranging from US AP1000s and Soviet/Russian VVERs to Canadian CANDU PHWRs, China sought to gain experience with as many reactor designs as possible, to incorporate this into new designs for use in the Chinese market, as well as for its exports. With the HTR-10 acting as China’s first PBR design, it would prove the stepping stone for following prototypes.
Today, HTR-10 is still online, after having been commissioned in 2000 and serving as a testbed for many aspects of the design, including various safety tests (alternative link) such as the complete loss of forced cooling, which is one aspect where the reactor’s negative temperature reactivity and TRISO fuel should prevent any accidents. The next Chinese PBR became the HTR-PM, where the ‘-PM’ stands for ‘pebble-bed module’, and which implements the HTR-Module concept for the first time with two 100 MWe (250 MWth each) cores. After construction began in 2012, it was commissioned in 2021, reached full power in 2022 and commenced commercial operations in December of 2023.
With the successful operation of its second PBR, the logical next step with such a HTR-Module-based plant will be to ramp up the number of modules to 6, which will be done with the aptly-named HTR-PM600. Its design is unchanged from the HTR-PM, just with more modules to increase the thermal power, with as design goal to make it fully compatible with the steam generators in existing (Chinese) coal plants. Since coal boilers operate at much higher temperatures than conventional water-based nuclear fission reactors, this was not possible before.
Currently there are 3,092 coal-fired plants operating in China, many of which could likely have their boilers replaced with HTR-PM600 PBRs while retaining the rest of the infrastructure. Along with applications in the (petro)chemical and other industries where high temperatures are needed, the HTR-PM600 as well as other HTR-PM configurations could be used instead, while providing flexibility in terms of load-following, and the used fuel, with thorium also being an option; a resource that China has significantly more of than uranium in its soil.
High-Temperature Future
At this point in time, China is the only nation in the world with commercial HTGRs in operation, as well as the only nation to run PBRs. Japan has been running its own HTGR called the HTTR since 1999, which is of an HTGR design with fuel rods that’s more closely related to the US’s HTGRs. It resumed operation in August of 2021 and is considered an important testbed for its own HTGR ambitions.
Whether PBRs or other HTGRs will make their way back to Europe or the US any time soon is uncertain, although US start-ups like X-energy with its Xe-100 PBR are looking to make it in the commercial market. The bright side for such start-ups is probably that a lot of the hard R&D work has been done by now, with general design aspects and fuel design less of a complete guess like in the 1960s. Going from there to a competitive design and finding the right target market still remains a major challenge, one which probably has many players looking at China as its modular PBR tries to establish itself first in the green pastures of the Chinese market.
The fascinating article mentioned “Die Wolke” (the cloud), but not the famous children song “Karl der Käfer” of 1983 (Carl the bug).
Luckily I’m here for you to fix that! 😁
https://www.youtube.com/watch?v=SLErVV1TxUI
https://de.wikipedia.org/wiki/Karl_der_Käfer
https://www.songtexte.com/uebersetzung/gansehaut/karl-der-kafer-englisch-bd7a18a.html
I forgot to mention the relationship here.
The song was often being associated with destruction of nature (forests etc), which nuclear plants were being associated with.
So it’s a song that environmentalists identified with, as well.
Die verdammte Grünen
Anyone else think that the power station on the book cover looks awfully like a coal plant? And what is coming out looks like either the usual cloud from the coal plant’s cooling towers (just harmless water vapour) or a bad case of smoke from the tall slender chimneys (obviously hidden behind the cooling towers from the vantage point of the cover illustrator). Coal plant smoke and ash actually has more radiation in it, due to trace levelsof uranium and other radioactive ores found in the rocks from whic coal is mined, than anything which comes out of a nuclear plant (even during a nuclear accident). Germany was VERY silly post-Fukushima in shutting down its nuclear plants, and opting to burn dirty lignite instead. Japan was also very silly post Fukushima in causing major social disruption by unnecessarily evacuating people from areas far enough from the damaged plant that they were at no risk from radiation hazards. If society ever wants to some day cease being dependent on fossil fuels, then nuclear energy is the only path to letting us move even slightly in that direction. Far too many “environmentalists” are anti-nuclear, you have to wonder if they really do care about the environment, or if they just want an excuse to boss people around and ruin the economy. If they cared about the environment they’d support an immediate mass construction programme for nuclear power plants.
Yes, I thought it was a coal plant too. Connecting it to Chernobyl never crossed my mind.
> Far too many “environmentalists” are anti-nuclear, you have to wonder if they really do care about the environment, or if they just want an excuse to boss people around and ruin the economy. If they cared about the environment they’d support an immediate mass construction programme for nuclear power plants.
In the environmentalists’ defence, there was considerable funding by the fossil fuel industry to make people scared of “nuclear power” so that fossil fuels could avoid scrutiny. e.g https://www.forbes.com/sites/kensilverstein/2016/07/13/are-fossil-fuel-interests-bankrolling-the-anti-nuclear-energy-movement/
https://www.ornl.gov/sites/default/files/ORNL%20Review%20v26n3-4%201993.pdf#page=26
This the 1993 report ‘Coal Combustion: Nuclear Resource or Danger?’ by Alex Gabbard, curtesy of Oak Ridge National Lab, Tennessee USA.
I draw your attention to the plot a couple pages in, showing global release of Uranium and Thorium, according to which by now we ought to be at around *eight thousand metric tons* of U into the atmosphere, due to burning coal.
Also points out coal makes about 6 MWh/ton, whereas U-235 does about 2000 MWh/ton. Even with particulate filters on the exhaust, “The amount of U-235 alone dispersed by coal combustion is the equivalent of dozens of nuclear reactor fuel loadings”.
And that’s not to say anything of the mercury, arsenic, lead, chromium and others being dusted down upon our heads and into our childrens’ lungs. I mean forget the radioactivity: These are ‘forever toxins’.
Anyway, an article further into the file, on the future of nuclear reasearch facilities says: “The challenge for nuclear power is to develop solutions to the twin problems of accident potential and waste disposal.”
The problem with triso fuel is this:
Sure, you’ve solved the ‘meltdown’ problem by making it basically impossible for the fuel to melt.
Better, you’ve banned water from the core: A highly sensible thing to do, because the defining feature of ionizing radition, is that it does radiolysis: it breaks molecules. Especially covalent bonds. Elemental nonreactive, or ultra-stable ionically bonded molecules for coolant need only apply. Also nothing that can boil, expand, and cause a steam-bomb explosion.
So PBR with triso has full marks for the ‘accident potential’, and this sort of thing is why we should never allow water-*cooled* cores again.
But what about waste disposal?
Solid fuelled reactors are literally ‘as dumb as a pile of rocks’, they ‘shit where they eat’.
So the limiting factor on fuel use is not actually using all the fuel, like you’d expect, but actually more than 80% to even 98% is typically *wasted*, because of the build-up of that ‘shit’.
Triso by design tries to seal everything inside, including all the reactor poisons which kill the neutron economy, which is why you only get to eat one slice of your cake, before having to throw the entirety of the rest away.
And *still*, even with that waste: roughtly 1/3 of a thousand times better than coal. And it could be about 50X better: Just by using a reactor that allows the waste to be removed. This is the promise of Liquid Fluoride Fueled reactors (whether or not they breed Thorium – whole other issue: only 0.72040% of natural U is U-235. But if you can use Th-232, then you get to use it all. And there’s typically 4 to 5 times more Th-232 basically anywhere you find natural U).
The really telling metric, btw, is when you figure out how many tons of earthmoving needs to be done, to mine for the energy. This is a lot harsher on the Uranium, since it’s never found at high concentrations the way coal is. Once you take that into acount, then solid-fuelled, shit-where-they-eat, dumb-as-a-pile-of-rock, steam-bomb reactors come out almost equal with coal. And that’s giving both a ‘free pass’ on waste disposal: For nuclear, that cost gets paid, so it works out as more expensive energy. Even more so, if you’re stupid enough to scale-down those plants. (eg: the Nuscale scam).
But merely for going liquid-fluoride fueled U, you get a factor of 5-50x *better than that*, whilst still being seriously accident-proof.
Sure, it can ‘melt down’, but in a liquid-thorium reactor, that’s so not a big deal: They are designed to melt down and auto-safe themselves without the slightest risk of damage to anything, and in such a way as they’d never even wear themselves out doing so. There isn’t even a valve that might fail (look up ‘freeze-plug’).
Rather than try to ‘banish’ melting and flowing of the fuel from the core, like TRISO does through brute force, they take advantage of it to move the fuel away where it can be safely passively cooled for years without attention.
And on top of that, no need to ‘play with your food’ like solid fuelled reactors. LFFR’s *do not care* what physical form the fuel is in, before you let it dissolve in the molten salt. No pointless expensive fuel manufacturing required.
So on the TRISO stuff: Meh.
China is also building an all-up Liquid Fluoride Thorium Breeder reactor. They’re deep in the guts of the predictable-as-hell schedule overrun you always get with a FOAK, but that one is the one to keep an eye in the news for. They’re a few years yet away from commercial operation, but I have no doubt they’re pulling out all the stops to get there.
Only question, is if Copenhagen Atomics gets there first? Those guys are doing one also, and their plan is to take full advantage of the LFTR design, to make even smaller modular reactors which can be mass-manufactured and shipped almost-ready-to-run. They intend not only beating those two problems – safety and waste disposal, but also doing so whilst making LFTR’s the *cheapest* energy on the earth, and that’s including coal.
Given the numbers and ratios above, I hope you can see why the physics say this is easily possible, even given additional costs of recycling both waste and used reactors.
>why is it that it was not the US, Germany or South Africa to first commercialize PBRs, but relative newcomer China?
Because the Chinese government really doesn’t care if the thing goes belly up and a couple million people die or get sick.
When Areva/EDF was building the new EPR fission plant in Finland, they also started building a copy of the reactor in China. The Finns noted several design flaws, omissions and errors that didn’t meet the safety standards and requirements, resulting in Siemens pulling out of the deal and numerous rounds of revisions and re-designing parts of the whole plant with years and years of delay and billions of dollars in cost-overruns.
The Chinese just went with their original EPR – “no problems here” – and the plant was built on schedule as it were.
“Because the Chinese government really doesn’t care if the thing goes belly up and a couple million people die or get sick.”
Yet a few years ago China banned import of scrap plastic for recycling because people were getting sick. From I think 1960s until a few years ago, China imported many of the plastic types that USA and Canada (and few more other countries) deemed not profitable enough to sort properly and clean residue before shredding them and sold as recycled pellets.
Apparently a large number of Chinese workers were getting sick off toxic residue found on waste plastic, and since China’s heath care are government funded, China choose to ban import to stop more of their people from getting sicker and costing more in medical expenses.
Nuclear accident will create a whole lot more problem than what imported toxic residue created. I would think China government would prefer not to have any accident at all, and will be holding every workers and their families’ head under guillotine should something go wrong.
I’m certain the Chinese can handle a nuclear accident just as well as the Soviets did. And make everyone else pick up the bill.
It’s a schizophrenic system. This is the same country that fischer-tropps coal into methanol to be blended into gasoline, which then causes formaldehyde smog in the cities. Left hand creates problems and the right hand “solves” them.
It just depends on who exactly is getting sick – if it’s some backwater peasants then who cares, right?
Would have to be pretty damned high % of meth in that gasoline to cause formaldehyde to become an issue.
It is a significant percentage, and there are a lot of cars, and the cars have poor emission controls.
“It just depends on who exactly is getting sick – if it’s some backwater peasants then who cares, right?”
You do get that you are describing the capitalist system perfectly right?
In China you run the risk of getting the death penalty for crap that kills lots of people.
In the US you run the risk of a bonus at best, and as a punishment you have to become CEO of the next company..
I capitalism, if “backwater peasants” are your majority of customers, you do care about what happens to them.
In communism, they’re just serfs to be used for profit to the state elite.
Healthcare in the PRC receives minimal government funding. From my observations in Beijing healthcare is all cash in advance.
LOL.
You think China sorted waste plastic…They burn it for fuel. At too low a temperature. Still, local supply is sufficient to poison population.
Sorting mixed plastic isn’t economical, even in Africa. At best/worst, they pick mixed waste plastic for the few types that can be recycled for a profit. Burn, bury or throw rest in river.
They banned waste plastic import as a f-you during a trade dispute. Was marginal to begin with. Only ever happened because of all the empty containers going to China.
Where did you get that derp from?
Statedepartment.us the ”grassroot propaganda’ recruitment page?
Going by the comments all over the internet I expect visiting that is mandatory.
Pebble bed reactors are wasteful of U-235. They need a higher enrichment (about 3 times what a LWR requires). Other than hype I don’t see real advantages over other reactor types.
There’s no way to entirely eliminate the cracking and chafing of the pebbles into dust, even with the improved pebble materials, so each and every one of these reactors will eventually become a contaminated nightmare that is very difficult and costly to decommission.
And knowing who’s operating the reactors, it’s entirely plausible that someone simply drops a fuel ball and fractures it, then puts it in the reactor in the hopes that nobody would notice.
Absolutely guaranteed to be the usual M.O. in China
But isn’t every reactor such a nightmare, kind of ?
I mean, the reactors from East Germany were very modern when they were new (~60s). Yet at same time, reverse-building them is a task that’s still not entirely completed, I assume. And their locations are surely still radioactive, too.
There’s some neutron activation of the materials in the reactor vessel anyways, but not fine radioactive dust from the fuel elements coating every imaginable surface. The biggest issue of the pebble bed reactor is that the fuel balls expand and contract, bump and chafe, as they move around the reactor and get extracted out the bottom, so you get that dust going everywhere.
In a conventional reactor, if a fuel element cracks and spills its contents around, that’s considered a failure. In a PBR, that’s normal operation.
The loose fuel particles will also contaminate the cooling loop outside of the reactor itself. The debris can also block cooling channels, and there’s at least one recorded incident where the dust was accidentally blown out of the reactor through an open valve and into the environment.
Thank you very much for the explanation.
The NRC just authorized the construction of a new reactor down at ORNL by Kairos Power, to test pebble bed fuel. 20 years ago, the chief of fuels in NRR (a real flaming asshole, I understand ) told the people who wanted to build pebble bed reactors that they needed to get real data about the fuel performance before anyone would accept their calculations. Real data about fuel performance at design rated temperatures, at design rated burnups, in the coolant that they wanted to license.
Now, it appears that the newest proponents of pebble bed fuel have decided to build an actual test reactor to see if the fuel performs as they expect.
The Germans could not produce consistent fuel pebbles unless one particular person was operating the machine that made them. And they had problems with the data from the fuel that they did irradiate because by its very nature, you never know where the pebbles are going to land in the reactor vessel, or what the power level is going to be at that location, or how the pebbles are going to move thru the pile and accumulate fission products or fluence. They had no data for fuel at its rated design burnup.
And the Germans admitted that it was hard to figure out which balls burned in which way. They had tagging wires in the balls to identify them, and give them some idea of the temperatures the balls experienced when they came out – the balls are recirculated constantly during operation. Some balls experienced higher temperature than was predicted. Some experienced higher exposure than was predicted. Some balls NEVER CAME OUT OF THE REACTOR DURING ITS OPERATING HISTORY.
How are these people going to come up with fuel performance models for fuel that is burned beyond its rated/tested/modeled experience?
We have no idea what the Chinese are doing, or what sort of qualification program they have for their fuel. If they have an accident, we will probably find out about it from radiation monitors in Korea or Japan, at their nuclear plants.
Chernobyl is in a sarcophagus and will remain sealed for centuries. When the new reactor is too contaminated and can’t be cleaned up anymore, they’d just seal it in their version of sarcophagus and forget about it. I just hope the sarcophagus isn’t built by the lowest bidder with corrupt inspector and the tomb starts crumbling after less than 10 years. Like some of the high profile housing disasters.
Reminds me of how nuclear weapons are currently tested, underground in a pit that caves in on itself to smother the fallout.
Perhaps that’s how nuclear power stations should be constructed, instead of sending in liquidators and sarcophagus builders to clean up the mess, build it in a hole and backfill it later, or underground with blast doors.
IIRC the Swiss have a melted down, gas cooled, weapons grade production, reactor inside a hollowed-out mountain. Right where they left it.
Only the Brits thought a convection flow, open _air_ cooled, weapons grade production reactor was good idea. Anybody experienced with English cars could have told you how that was going to end.
Heineken!? F*** that s**t!
Pebble! Bed! Reactor!
@lol – LOL!
Is it not caring about the possible down sides that has China researching nuclear to the extent that they are, or are they simply doing what they see as the best thing for carbon emission reduction? Where China differs from much of the rest of the world is that their economy isn’t at the whim of dozens if not hundreds of special interest groups looking to profit from competing energy sources.
Will this march to nuclear by the Chinese cause more accidents? Are they under the scrutiny of the IAEA? Or can we all benefit from what they’re attempting?
Going green is great, but you gotta have a power source that isn’t at the whim of the weather for times when mother nature isn’t being cooperative. That can be fossil or nuclear. Pick.
> their economy isn’t at the whim of dozens if not hundreds of special interest groups looking to profit
Instead, their economy is at the whim of dozens if not hundreds of communist party affiliates and insiders looking to profit from lucrative government projects with little to no oversight or responsibility, since they are the authority themselves. If something goes kaboom, scapegoats will be found elsewhere.
Except for the party name, this is de facto the same in almost every other country as well.
Cronyism is a problem the world over. It’s just worst in countries that operate under state capitalism, since the largest business owners and the government are the same people.
No such thing as ‘state capitalism’.
It’s just pure state, without competition there is no capitalism. Can a state business fail?
Like ‘corporations’ under Mussolini.
Beware commies redefining terms.
Remember, ‘they’ think they’re the smart ones. Are in charge of language and word definitions. e.g. They’re not commies, they’re for ‘economic democracy’. Fact: Are commies, the renaming habit is tell.
>without competition there is no capitalism
Depends on what you mean by “capital”. The way I understand it, capital refers to the productive self-replenishing assets of the society, or any value convertible into such. Capitalism refers to the private accumulation and ownership of such means of production, while state capitalism means the state ownership and control of these means of production – as opposed to socialism where the means of production are supposedly owned by the people.
However, as socialism in theory doesn’t really consider individuals to be individuals in their own right, but only “actualized” as parts of a state, in both communism and socialism, the state replaces the individuals and assumes control of the productive apparatus. When combined with the realization that the actual state is never the same as the people, but composed of a small administrative elite, it comes around to note that socialist and communist systems will always be state capitalists since they consist of a private group of people who aim to accumulate and control all the means of production in the society.
it’s the top-down management. NIMBYs are less prominent in China because they get squashed. Just like my comments on Chinese news sites lol
“With China recently having put its twin-PBR HTR-PM plant into commercial operation, why is it that it was not the US, Germany or South Africa to first commercialize PBRs, but relative newcomer China?”
Why China?
1. Design is easy, fast & cheap to copy.
2. Science-based design, not politics-based.
The nucular power industry has an empirically-derived, well-proven probability for causing wide-ranging environmental devastation. Any chance, over time, approaches…certainty! – Exorbitant, black energy.
There is no known chemical or nuclear reaction, for reversing the damage caused by nucular power. It takes time, and a very, very large amount of it. Don’t let what cannot be undone, add to the legacy of what can be remitted, conceivably but with great difficulty, for an undeserving future to deal with.
This guy is why we can’t have nice things
But you can, abundantly so. You just have to do a little of your own thinking.
Where did you cut and paste that derp from?
When we were kids we would bicycle over to the St. Vrain power plant because they had a pretty cool little public area where they had a bunch of details about how the plant worked and some cute energy conversion demos. The plant was always one minor modification away from working, as they found one expensive thing after another that wasn’t quite designed right and needed modification. It would have been cool if they’d ever actually gotten it economically feasible, but it was the most amazing money sink.
In the meantime, the dragon keeps moving along while the West is getting entangled with onerous regulations!
As a German growing up in the 80s I never heard about this Book
Kommt drauf an, wann du geboren bist; das Buch erschien auch erst 1987. Ich bin Jahrgang 81 und bei uns war das in keiner Stufe Pflichtlektüre. Aber in den Kreisen, in denen meine Eltern verkehrten, war es definitiv ein must read und wurde eigentlich von allen Gleichaltrigen gelesen, die ich kannte.
Ich erinnere mich noch, dass es nicht die sehr abstrakte Gefahr des havarierten KKW war, die mich damals beeindruckte, sondern der Tod Ulis auf der Straße – ganz schnöde in einem Verkehrsunfall. Ich erinnere mich aber heute noch an 1986 und Chernobyl war für viele Leute damals ein Vorkommnis wie später erst wieder der 11. September.
It depends on when you were born; the book wasn’t published until 1987. I was born in 81 and it wasn’t compulsory reading for us at any level. But in the circles in which my parents socialised, it was definitely a must-read and was actually read by everyone my age that I knew.
I still remember that it wasn’t the very abstract danger of the damaged nuclear power plant that impressed me at the time, but Uli’s death on the road – quite simply in a road accident. However, I still remember 1986 and Chernobyl was an event for many people at the time, just as 9/11 was later.
They talk very little about South Africa’s involvement with PBMR’s. The University where I got my degree (in South Africa) did research on pebble bed reactors. Today at the Mechanical & Nuclear Engineering Faculty you will still see pictures of it on the walls. They made a lot of breakthroughs. Different companies were created and software like flownex was developed to manufacture and simulate the PBMR.
From what I understand, the funding was cut and the government took the research. The whole thing was just stopped. People are still upset about it today. Most of them retired and/or left the university.
I suspect that the research was given/exchanged/sold to China, and this helped them to make great strides in the technology and development of the reactors.
And no it does not make any sense. Welcome to South africa.
Maya should do some more research. I suggest that she go into any working-class bar in the vicinity of the Three Mile Island nuke plant and shout out the words “Nuclear power never killed anybody!”
But that is all past and gone. A team of Koreans has shown us the way to a new and better world. “We don’t need the Laws of Thermodynamics!”
“…The technology aims to revolutionize energy generation by providing constant output frequency without the need for fuel…This innovation is scalable and can be used in power plants, a house off-grid, electric vehicles with no need for recharging or refueling, ships, planes, and mobile phones. The sky is the limit…It is a self-powered generator, has a compact footprint, and a near-permanent lifespan. It is the next leap in the evolution of technology’…”
https://interestingengineering.com/innovation/first-ai-nonrotational-power-cop28
The view of Dubai at night on the Web page below shows once again that “Forests precede civilizations, deserts succeed them.”
https://www.semp.or.kr/en/%EB%B0%9C%EC%A0%84%EA%B8%B0%EC%9D%98-%EC%97%AD%EC%82%AC
3 mile island was barely an accident compared to chernobyl.
The NRC just authorized the construction of a new reactor down at ORNL by Kairos Power, to test pebble bed fuel. 20 years ago, the chief of fuels in NRR (a real flaming a@@hole, I understand) told the people who wanted to build pebble bed reactors that they needed to get real data about the fuel performance before anyone would accept their calculations. Real data about fuel performance at design rated temperatures, at design rated burnups, in the coolant that they wanted to license.
Now, it appears that the newest proponents of pebble bed fuel have decided to build an actual test reactor to see if the fuel performs as they expect.
The Germans could not produce consistent fuel pebbles unless one particular person was operating the machine that made them. And they had problems with the data from the fuel that they did irradiate because by its very nature, you never know where the pebbles are going to land in the reactor vessel, or what the power level is going to be at that location, or how the pebbles are going to move thru the pile and accumulate fission products or fluence. They had no data for fuel at its rated design burnup.
And the Germans admitted that it was hard to figure out which balls burned in which way. They had tagging wires in the balls to identify them, and give them some idea of the temperatures the balls experienced when they came out – the balls are recirculated constantly during operation. Some balls experienced higher temperature than was predicted. Some experienced higher exposure than was predicted. Some balls NEVER CAME OUT OF THE REACTOR DURING ITS OPERATING HISTORY.
How are these people going to come up with fuel performance models for fuel that is burned beyond its rated/tested/modeled history?
I think the reason, it was not commercialized in US or Germany was, that it was not allowed to have basically unlimited energy resources. And as soon as the people would have been used to it, no one could argue for higher energy price tag. But increasing the cost of anything, and especially energy, is a tool to reduce population growth, which is planned since a long time. The other thing is, that all high temperature fission plants would burn up the fission waste products very rapidly. Solving the radioactive waste issue. Same is true for the molten salt reactor concept or the sodium cooled ones. We will see, if China is getting away with it.
You can buy solar panels today for 1% of the cost of these. They provide a basically unlimited energy resource right now?
Solar energy is actually extremely limited. Even in ideal conditions found in only a few parts of the world, solar power budget is limited to about 10 kWh/m**2 per day. The “best of the rest” is closer to half that.
And that’s ignoring practical efficiency and materials+energy costs of the panels themselves.
Solar is very useful when appropriate, but it’s hardly “unlimited”.
Given an unlimited number of snakes, you could literally and unironically power the world on snake oil. The problem is feeding the snakes…