The UK’s most famous fusion reactor – the Joint European Torus (JET) tokamak – saw its first plasma on June 25th of 1983. Its final plasma pulse was generated on December 18th of 2023, for a total of 105,842 pulses over forty-and-a-half years and countless experiments.
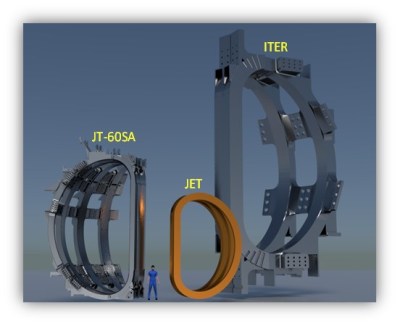
Originally designed in the 1970s by Euratom members, JET formed the core of Europe’s fusion research program, allowing many of the aspects of tokamak systems to be explored, including deuterium-tritium fusion. Its final day of experiments involved an inverted plasma shape prior to targeting electrons at the tokamak’s inner wall, to study the impact of such damage.
Although JET has received a number of upgrades over the decades, the MAST Upgrade and upcoming STEP fusion reactors at the Culham Centre for Fusion Energy (CCFE) are now headed where JET’s design cannot go. Current advanced tokamak reactors like Japan’s JT-60SA are increasingly using super-conducting coils with often plasma volumes far beyond JET’s, with the focus shifting from plasma research to net energy production.
This means that unless JET somehow gets repurposed/upgraded and recommissioned, this is the final goodbye to one of the world’s most famous and influential fusion reactors.
(Top image: Internal view of the JET tokamak superimposed with an image of plasma flows)
could we stop romanticizing lab equipment just because it is really big? you don’t say goodbye to a centrifuge or autoclave. it gives people weird ideas like trying to “save” things that are outdated, always using other peoples’ money, of course.
Hot take: the Hubble space telescope was essentially disposable lab detritus and we shouldn’t try to squeeze every remaining bit of discovery out of it.
Yes. Now they’ll have to drag it to some museum and spend even more money building an exhibit for it. “Yes kids. This is JET…it was fusion reactor that never actually produced any energy. People spent their whole lives trying to figure out how but never actually did.”
I’d say it’s more becuahe equipment is unique – you can throw centrifuge and get exactly identical copy next day. Plus big device gives big possibilities, that’s kind of unique too
Pour one out for Bela Lugosi the Autoclave and She-Ra the Centrifuge. Everyone should name their lab equipment.
Can’t see how a real fusion reactor will achieve that many pulses due to containment withstanding center at a temperature hotter than the sun. Temperature is strong and gives both high steady state and alternating thermal stress. Perhaps will get some alien materials. So far only a few seconds with other reactors bring that hot.
I worked at Culham, over 40 years ago. I sort of assumed they would have got the fusion stuff worked out by now and i would be telling my kids how daddy helped solve the problem :) However despite the naysayers I am still optimistic that we are on the final leg of workable fusion power, and people forget how far the technology has moved on in that time. When I worked there, everything was run via PDP-11 and superconducting technology was in its infancy. It is now a question of putting all the engineering together
However, there will now be a break-up of the vast experience of the JET team. Most will move to places like ITER, but I do feel that the site should have been kept going to ensure that 40 year knowledge base was retained
I worked with a guy that built the state machine that replaced the PDP11, all by himself, clever guy.
There will be plenty going on at Culham for the next few years, not least abjq-jr will be doing his PhD research there on some plasma physics thing for nuclear fusion. Also not so far away in Nottinghamshire will be the STEP reactor.
I think the Russians have had quite a laugh at our expense though with the Tokamak concept, it leaks too much energy to make a sustainable reactor.
Bumper Harvest:
* Virgin Hyperloop One – Poof Gone
https://www.youtube.com/watch?v=W_W_BDcebao
* Wireless EV Road Charging – Poof Gone
https://www.youtube.com/watch?v=sisD61ohzK0
* Joint European Torus (JET) Tokamak – Poof Gone
https://hackaday.com/2023/12/23/uks-jet-tokamak-retires-after-40-years-and-105842-pulses/
What do they do with it now? Those liner plates and surrounding metal must just glowing due to 40 years of neutron activation. I might make more financial sense just to keep doing science rather than try to decommission and decontaminate. Or leave everything in place and fill it full of concrete.
They’ve been planning decommissioning for a while.
https://www.niauk.org/wp-content/uploads/2022/07/Sam-Holdsworth-UKAEA.pdf
They’re not *that* radioactive (compared to like, nuclear disposal stuff) but it is high enough to be a problem for the actual deconstruction. Nothing’s higher than intermediate-level waste, and it’ll all be low-level waste within a decade.
As for “why not just keep doing science” – decommissioning it *is* doing science, since it’s the only significant D-T burning facility out there. The decommissioning process involves a lot of novel science to detritiate the materials and reduce it to low-level waste.
They have said for years that to make it work will take 40 years so I will make a prediction it will always take 40 years ad infinitum
Radioactive? You mean fusion power doesn’t just produce clean helium gas? I don’t know what to think about that. We’ve been told this is unlimited clean energy.
No, it’s unlimited tax money!
B^)
“We’ve been told this is unlimited clean energy.”
Please note
“Nothing’s higher than intermediate-level waste, and it’ll all be low-level waste within a decade.”
Clean is always relative.
Make me wonder if you could design a system, that at the end of its useful lifetime, could be fully dissolved with various acids. And then stored for 30+ years like the waste from fission reactors before either permanent storage or reprocessing.
My guess (no research done, yet) would that the low Z isotopes from a fusion reactor would have extremely short half-life. In many ways it is even scarier to work with, initially, than the waste products from a decommissioned fusion reactor (high Z isotopes, which I’m guessing on average would have a much longer half-life)
> decommissioned fusion
Sorry I meant fission
“would that the low Z isotopes from a fusion reactor would have extremely short half-life. In many ways it is even scarier to work with”
No, it really, really isn’t. First, if you have a lump of “stuff,” knowing its half-life is short or long doesn’t tell you how deadly it is. Yes, obviously, if you’ve got 10,000 *atoms* and they’re *all* radioactive, generally the longer half-life, the better, as evidenced by the fact that you can buy pure bismuth and it totally doesn’t matter. But you don’t, you have a lump of “stuff.” So more than “short or long half-life” it matters how much of your stuff is radioactive, and how many atoms are in that stuff.
Second: your initial premise is wrong anyway – the average half-life of low-Z isotopes is much higher… because most low-Z isotopes are *stable*. If you then say “that’s cheating, I mean just the radioactive ones” – it’s not cheating. Since most low-Z isotopes are stable, that means the decay chains for low-Z radioactive isotopes are only a single step, and that’s very important for dealing with them. A random mass of high-Z isotopes will have an utter mess of elements in it, making it much more complicated to deal with and process.
Managing radwaste in fusion reactors primarily means minimizing the formation of long-lived isotopes and preventing tritiation of materials since hydrogen is so mobile (and tritium is so valuable).
You want tritiation in the lithium blanket, though, to breed more fuel.