The UK’s most famous fusion reactor – the Joint European Torus (JET) tokamak – saw its first plasma on June 25th of 1983. Its final plasma pulse was generated on December 18th of 2023, for a total of 105,842 pulses over forty-and-a-half years and countless experiments.
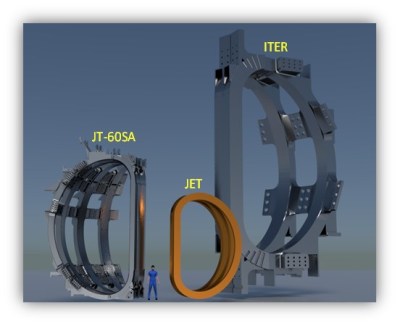
Originally designed in the 1970s by Euratom members, JET formed the core of Europe’s fusion research program, allowing many of the aspects of tokamak systems to be explored, including deuterium-tritium fusion. Its final day of experiments involved an inverted plasma shape prior to targeting electrons at the tokamak’s inner wall, to study the impact of such damage.
Although JET has received a number of upgrades over the decades, the MAST Upgrade and upcoming STEP fusion reactors at the Culham Centre for Fusion Energy (CCFE) are now headed where JET’s design cannot go. Current advanced tokamak reactors like Japan’s JT-60SA are increasingly using super-conducting coils with often plasma volumes far beyond JET’s, with the focus shifting from plasma research to net energy production.
This means that unless JET somehow gets repurposed/upgraded and recommissioned, this is the final goodbye to one of the world’s most famous and influential fusion reactors.
(Top image: Internal view of the JET tokamak superimposed with an image of plasma flows)