To those who have kept tabs on nuclear fusion research the past decades beyond the articles and soundbites in news outlets, it’s probably clear just how much progress has been made, and how many challenges still remain. Yet since not that many people are into plasma physics, every measure of progress, such as most recently by the South Korean KSTAR (Korea Superconducting Tokamak Advanced Research) tokamak, is met generally by dismissive statements about nuclear fusion always being a certain number of decades away. Looking beyond this in coverage such as the article by Science Alert about this achievement by KSTAR we can however see quite a few of these remaining challenges being touched upon.
Recently KSTAR managed to generate 100 million degrees C plasma and maintain this for 48 seconds, a significant boost over its previous record from 2021 of 30 seconds, partially due to the new divertors that were installed. These divertors are essential for removing impurities from the plasma, yet much like the inner wall of the reactor vessel, these plasma-facing materials (PFM) bear the brunt of the super-hot plasma and any plasma instabilities, as well as the constant neutron flux from the fusion products. KSTAR now features tungsten divertors, which has become a popular material choice for this component.
Researching the optimal PFMs, as well as plasma containment modes and methods to suppress plasma instabilities are just some of the challenges that form the road still ahead before commercial fusion can commence.
High-Confinement Mode
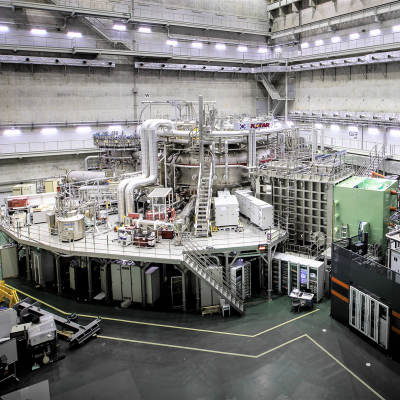
The high-confinement (or H-mode) operating regime of tokamaks was first discovered in 1982 during experiments with the German ASDEX tokamak. H-mode differs from the retroactively named L-mode (for low-confinement) in that it features a much more stable plasma edge, due to as of yet unknown mechanisms that take effect when the plasma is heated to above a certain level.
One issue with H-mode is however the occasional edge magnetohydrodynamic (MHD) instabilities, called edge-localized modes (ELM). These occur as disruptions in the otherwise stable edge barrier in H-mode, forming a sudden burst of plasma that can eject up to 20% of the plasma’s energy into the nearby PFM. This causes damage to the wall and divertors in the form of erosion (ablation) and has been a major research topic for the past decades, with control methods like resonant magnetic perturbations (RMP) being applied as early as 2003. In 2011 KSTAR became one of the tokamaks that had managed to successfully suppress (Type I) ELMs using non-axisymmetric magnetic perturbations.
Beyond causing PFM damage, these instabilities in H-mode are also problematic in that they reduce the efficiency of the reactor, due to heat and other losses that then have to be compensated for. Yet the corollary of such a stable edge in H-mode with Type I ELM suppression is that the removal of impurities and heat from the plasma becomes very difficult. This is where the much smaller, Type II ELMs are relevant, as they provide a means to transport impurities across the edge barrier without threatening the PFM.
This was simulated and experimentally tested in the ASDEX Upgrade tokamak in 2022, with the results by G.F. Harrer et al. published in Physical Review Letters. Suggested is an operating regime for ITER and similar tokamak fusion reactors in which these Type II ELMs are used as a functional feature of the plasma, whereas Type I ELMs would continue to be suppressed. As for whether RMPs are the ideal way to suppress Type I ELMs, this too remains the subject of research.
Greenwald Density
Beyond H-mode and ELMs, tokamaks also have to deal with what is named the Greenwald Density Limit (GDL), named after Martin Greenwald, whose 2002 review article (PDF) in Plasma Physics and Controlled Fusion is worth a read for a detailed summary of the subject. The short version is that it pertains to the plasma density, with each fusion reactor having an observable lower and upper limit to this density. Once the limit is reached and exceeded in a tokamak, the reaction will transition from H- to L-mode, along with other negative effects . Of note is that the scaling of these limits seems to depend on a wide variety of factors, far beyond merely the size of the reactor, such as the fueling method.
The Greenwald limit is more easily found with stellarator fusion reactors, as these do not display the same strong negative response to exceeding this limit. Instead they show a soft limit, or quench, that sees the plasma temperature decaying. The density limit in stellarators has been found to be significantly higher (up to double) than in comparably sized tokamaks, giving the former an edge over tokamaks, as well as in terms of plasma stability. Despite this, it is thought that these limitations of tokamaks can be compensated for, with a 2024 article by S. Ding and colleagues in Nature detailing a high-density, high-confinement tokamak plasma regime in the General Atomics DIII-D tokamak.
Can’t Rush Science
If you ask an engineer how long it’ll take to build something, they can likely give you a fairly accurate estimate, as well as an idea of the required materials and manpower. If you ask a plasma physics scientist how long it’ll take to build a nuclear fusion reactor that has an energy gain (Q) of 8 or better, they’ll likely give you a quaint look. If you’re lucky they’ll humor you and introduce you to the wonderful world of nuclear fusion related research papers, especially those pertaining to ITER-related research.
During the century since nuclear fusion was discovered, scientists have at some points thought that they were close to tackling nuclear fusion on Earth. The most pertinent being the Z-pinch machine during the 1950s, which was the moment when the solution seemed so close. This was also the moment when the exciting world of plasma physics began to be more fully revealed to the world of science, along with plasma instabilities, heat losses and a rush to find materials that could cope with the neutron flux and heat exposure inside the then brand new tokamak reactor design.
Would larger tokamaks work better? This was just one question of many, even as fundamental research on plasma physics continued and the tokamaks at research institutes around the world underwent one revision after another based on newly gained knowledge and new directions in research. Along the edges stellarators continued to get some love too, especially once computer simulations became powerful enough to figure out an appropriate magnetic field configuration. Much like the plasma inside these experimental fusion reactors, so too did progress keep flowing.
Although sideshows like inertial confinement fusion keep stealing the show despite being anything but practical for energy production, it is clear that we are lightyears beyond where we were with nuclear fusion research in the 1980s, as well as the 2000s. We cannot say yet when the moment will come when the first Q >8 fusion reactor will come online, with sustained tritium breeding and all of the other amenities that befit a commercial fusion reactor, but that’s why we are still in the research and development phase.
Maybe it will take another decade, or two. Maybe we’ll have another sad Z-pinch moment where physics throws us another curveball and we have to sigh, collect our shattered self-esteem and waddle back to the drawing board for another shot at the challenge. This is both the thrill and curse of fundamental research and development, with often massive potential rewards, and absolutely zero guarantee of reaping any of them, other than learning so incredibly much along the way.
Can’t rush science (except during wartime)
Science is like tribbles, feed’em you get results, starve’em not so much. If you want practical fusion in 20 years a worldwide, coordinated effort and 10 trillion a year might get you there, don’t want to spend that much? You’ll have to wait.
That usually ends in “boom”.
You can’t rush it, but you can slow it down by cutting the funding – and by creating high profile “dummy” projects that eat up whatever funding remains without accomplishing much anything.
or during a global pandemic.
science moves quickly when ethics oversight mechanisms are switched off and the federal government is writing you blank checks.
I’m not sure they were “switched off” so much as put on high alert to give absolute priority and the speediest possible progress to one or two specific things over and above everything else.
Citation needed
I for one look forward to practical fusion reactors in only 20 or so years. Wait, you already mentioned dismissive statements, so I’ll show myself out.
(in all seriousness, I do look forward to seeing a sustained reaction that output more energy than what it needs)
Open the curtains and look up. There’s one going nicely there. Of course, we didn’t build that one so we can’t claim the credit, but it’s there’s for the using.
Perhaps we can shoot bags of money into it!
The Saint (1997) showed me the secret to Cold Fusion only needed rearranging of some scraps of paper.
Funny how things like that haven’t come true, and yet every movie about invasive Government spying basically has.
There is definitely a good chunk of unknown physic near the atom lattice interactions with hydrogen. Maybe it won’t be anything practical but it’s a dark area of knowledge.
> managed to successfully suppress (Type I) ELMs using non-axisymmetric magnetic perturbations
I bet they managed to prevent side-fumbling as well.
I can never work out if it’s the spurving bearings being in direct line or the hydrocoptic marzlevanes that eliminate side fumbling. Having said that, I’m only a hobbyist not an engineer.
You have to wonder if they’ve tried applying turboencabulator technology to fusion. It’s a crudely conceived idea, but I think it could be brought to perfection here.
All politics aside, for over a decade inthe 70s and 80s, I very closely monitored the Fusion Energy Foundation founded by none other than the uber goofy Lyndon Larouche. They were nonetheless the outlet for updates in the field of interest.
https://en.m.wikipedia.org/wiki/Fusion_Energy_Foundation
my guestimate is about 100 years based on various milestones over time. also the amount of time it seems to take to build and operate a tokamak times how many more i think we need. iter claims it will be 2, but i think it will be more like 4 or 5.
i also dont think the fusion startups will play any significant role in the matter. they seem to always be asking for funding while iter drops some of the biggest superconducting coils known to man into its reactor. its really a case of go big or go home. i stopped watching videos about those because every one seemed like an ad for the machine.
Check out the National Ignition Facility.
https://lasers.llnl.gov/
A facility whose primary purpose is the simulation of thermonuclear implosions as just one part of the VERY impressive and extensive US nuclear warhead Stockpile Stewardship program required to develop accurate computer simulations of nuclear weapons to run on the various DOE supercomputers.
Besides potentially allowing the creation of some amazingly specialized and interesting 4th generation nuclear weapons if the comprehensive test ban treaty is ever ended, they allow the repair of our aging warheads and replacement of parts which require replacement while still being confident they’ll work after that’s done without the need to test them.
The construction of the National Ignition Facility began the year after the comprehensive nuke testing ban. What an amazing coincidink, no?
Fourth Generation Nuclear Weapons (FGNW): Military effectiveness and collateral effects – February 2, 2008
https://arxiv.org/pdf/physics/0510071.pdf
3.1 Inertial confinement fusion experiments and FGNW
Inertial confinement fusion (ICF), which basically consists of exploding very small amounts of thermonuclear fuel highly compressed by lasers or other means, enables to study the physics of thermonuclear secondaries in the laboratory (see Fig. 2). While this technique has the potential to be used in a thermonuclear reactor to produce energy, it has primarily been developed as an alternative to the underground testing of nuclear weapons, and as a tool for designing new types of nuclear weapons [1].
Basically, as can be seen by comparing Fig. 1 and 2, ICF reproduces in the laboratory the same arrangement than the one on which two-stage H-bomb are based: the Teller-Ulam principle.
————-
The French version:
Laser Mégajoule (Wikipedia)
Laser Mégajoule (LMJ) is a large laser-based inertial confinement fusion (ICF) research device near Bordeaux France, built by the French nuclear science directorate, Commissariat à l’Énergie Atomique (CEA).
Laser Mégajoule plans to deliver over 1 MJ of laser energy to its targets, compressing them to about 100 times the density of lead. It is about half as energetic as its US counterpart, the National Ignition Facility (NIF). Laser Mégajoule is the largest ICF experiment outside the US.
Laser Mégajoule’s primary task will be refining fusion calculations for France’s own nuclear weapons.[1] A portion of the system’s time is set aside for materials science experiments.[2]
Construction of the LMJ took 15 years and cost 3 billion Euros.[3] It was declared operational on 23 October 2014, when it ran its first set of nuclear weapon related experiments.
————
By comparison to the endless, incredibly expensive fusion experiments, how much is being spent on developing these?
Generation IV fission reactors
1. Nuclear waste that remains radioactive for a few centuries instead of millennia
2. 100-300x energy yield from the same amount of nuclear fuel
3. Broader range of fuels, including unencapsulated raw fuels (non-pebble MSR, LFTR).
4. Potential to burn existing nuclear waste and produce electricity: a closed fuel cycle.
5. Improved safety via features such as ambient pressure operation, automatic passive reactor shutdown, and alternate coolants.
Nuclear is still the best option.
No, it’s really not… It’s more than twice as expensive as solar and wind. It’s less modular. Less safe. Has waste disposal issues. Is not falling in price exponentially over time. Has fuel procurement issues. Can not be modified or maintained post hoc. And is subject to e.g. earthquakes and terrorism and extreme weather… and say lack of water in an increasingly drought ridden world… And btw for all you sports fans out there, here’s a statistic you can bet on: we’ve depleted many of the aquifers in the US by e.g. 400 km^3 of water volume… It takes 10 years to permit and build a reactor. It takes 6-24 months to build a solar field, depending on the size. And solar captures 20% of 1.2 GW/km^2, which is not much less energy dense than fission.
And don’t even mention the term “baseload power” to me in this conversation. That term is obsolete and fyi, there’s an energy storage revolution going on with dozens of prototyped technologies and at least a handful in commercial production.
Concerning fusion power, the author is too cynical. The progress is greater than is being reported here in a number of dimensions. My ph.d. is in statistical inference… here’s my inference. Whereas many startups among 60-80 contenders are talking about working prototype reactors in the 2025-2028 time frame, I figure that means 2030 or so. They won’t be commercially viable. From there I think it’ll take 10 years to scale things up and bring something to market… E.g. 2040 for commercialized, deployed fusion power.
Meanwhile there’s very very good reason to believe climate change is going to knock the world off its rocker… e.g. 2040 is also the year when the pH of the ocean falls to a point where the bottom gets ripped out if the marine food chain, the SML on the ocean’s surface disappears and we have uncontrolled evaporation leading to steadily mounting vapor in the atmosphere which is a more potent GHG than CO2…
And also, it’s past the point where we hit 2 deg C of warming. Heat waves are 4x as common and crop failures 4x as common at 1.5 deg C of warming. Heat waves are 13.8x as common and crop failures 20x as common at 2 deg C of warming.
We had the hottest summer in 2000 years last summer at a 95% level of confidence by 0.5 deg C. India and SE Asia had temperatures at or over 100 F for > 28 days during April 2024. The wet bulb effect is starting to boil people alive. Meanwhile this week monkeys are falling dead out of trees in Mexico as they record their hottest temperatures ever. And Mexico City is looking at a complete failure to provide water to its 22 million residents within 2 months.
How’s your Spanish and Hindi and Mandarin and Vietnamese everyone? I hope you’re prepared for e.g. 10% of 2 billion climate refugees between now and 2035. If they stay where they are, they die. Therefore… a certain amount of regional instability is likely to come to a future near you…
I’m not at all clear we’ll make it to 2040 for fusion to ever happen. I could talk about tipping points and feedback cycles for hours and still not finish what I have to say on the matter.
A few choice aphorisms:
“Agriculture is the Achilles Heel of humanity”.
“Scarcity breeds aggression and stupidity breeds scarcity”.
“One year’s lost wheat crop is a disaster. Two years in a row is a recipe for WW3”. (Red Storm Rising, Tom Clancy, anyone?)
“The world is like a lilypad… with every frog in the pond trying to jump to the same lilypad for refuge while expecting every other frog in the pond to sit still”
“The transient response of a positive feedback cycle is an exponential function of time. Avalanches don’t meander. They avalanche”.
” ‘To the victor go the spoils!’ said no rat on any sinking ship, ever! More like ‘There can be only one!’ ”
Humanity’s exploitation of > 1 million years worth of stored sunlight energy in chemical form via combustion within 100 years is tantamount to moving the Earth closer to the sun. Burning 100 million barrels of oil a day releases the same amount of energy into the atmosphere (1%), land (5%), ice(5%) and oceans (91%) as detonating 15 Hiroshima sized nuclear bombs every second of every day. And that’s why we’ve sunk 500 zetajoules, ie 500e21 joules, ie 500 billion trillion joules into the Earth’s oceans… And surprise surprise. Actions have consequences blame Newton. Or find whichever government politician created the Law of Conservation of Momentum and sue them…
>It takes 10 years to permit and build a reactor. It takes 6-24 months to build a solar field
That’s not a fair comparison, because it’s missing half the picture where you also need to build the extended transmission infrastructure, energy storage capacity, and demand side management infrastructure and control systems to deal with the intermittency and availability issues of solar power.
Nuclear power is a drop-in solution to the existing system. Solar is not – as of yet it only works up to a point where it’s still small enough to not cause problems. It took a hundred years to build the power grid as it is, and now you’re looking at building networks of superconducting power lines extending from Algeria to Norway in order to actually make use of renewable power on the same scale.
> No, it’s really not… It’s more than twice as expensive as solar and wind.
Nuclear used to be a lot cheaper. Ideally, we could find out what is causing the seemingly exponential rise in cost and work to solve it.
> It’s less modular.
Than solar, yes. Than wind, not really. Wind turbines need big cement bases.
> Less safe.
Than 50+ year old designs, certainly! But then even digital multimeters are less safe than modern CAT rated designs. For an example of a reactor that can’t melt down see. [1][2]
> Has waste disposal issues.
So does solar, wind, and the Li-Ion battery units they employ — at least in the USA. You see, we don’t have or have very few of the recycling plants capable of handling all of the above.
> And is subject to e.g. earthquakes and terrorism and extreme weather…
Solar and wind are subject to the same as even the most forgiving outlets will point out.[3]
> and say lack of water in an increasingly drought ridden world…
Umm, we live in a closed system. The water is still on planet earth… somewhere.
But perhaps you mean that we’re not replenishing our aquifers in particular?
Then I should point out that datacenters basically flush water down the sewers instead of using a CLC system like our PC water coolers use.[4] Nuclear isn’t the only water hog currently. Another good example of a water hog is Mosaic, who strip mine phosphate and, in the process, both use huge amounts of water and destroy the Florida aquifer system.
But even if you argue that nuclear should cut waaaay back on water usage. As pointed out above, as a result of advances in technology, this is possible.[2]
> Meanwhile there’s very very good reason to believe climate change is going to knock the world off its rocker… e.g. 2040 is also the year when the pH of the ocean falls to a point where the bottom gets ripped out if the marine food chain, the SML on the ocean’s surface disappears and we have uncontrolled evaporation leading to steadily mounting vapor in the atmosphere which is a more potent GHG than CO2…
Umm, maybe you don’t recall, but the earth used to have much much higher levels of CO2 and the ecological system survived and worked around the supposed problem just fine.[5]
> “Agriculture is the Achilles Heel of humanity.”
In keeping with your line of reasoning, if we really are at the brink of collapsing ecosystem, then we wouldn’t keep destroying our farmland (here in the USA).[6][7]
[1]: https://x-energy.com/reactors/xe-100
[2]: https://www.energy.gov/ne/articles/x-energy-developing-pebble-bed-reactor-they-say-cant-melt-down
[3]: https://www.npr.org/sections/live-updates-winter-storms-2021/2021/02/18/968967137/no-the-blackouts-in-texas-werent-caused-by-renewables-heres-what-really-happened
[4]: https://www.techradar.com/news/googles-data-center-water-usage-is-revealed-and-its-a-lot
[5]: https://www.nature.com/articles/s41598-017-08234-0
[6]: https://theworld.org/stories/2020/07/31/farmland-losing-development
[7]: https://farmlandinfo.org/statistics/farms-under-threat/
Fusion has always been “20 years away” for the whole of my life. In June, I am pleased to say I will be starting a new job working as part of the UK’s Fusion program. I genuinly believe it will be a possibility within the remaining years of my career, and I really am looking forward to making it a reality. It’s a matter of “when”, not “if”, and there is still a long way to go before it’s on the same scale as Fission.
When you look at how quickly we have adopted Fission, I do not doubt that once it’s proven stable and possible it’ll accelerate adoption in the coming decades.
Exciting times!
Genuine question – where is the tritium going to come from to fuel the reactor?
Don’t ask your boss as you will probably get fired.
https://www.iter.org/newsline/-/3852
>a simple physics principle: when a neutron impacts a lithium nucleus, the reaction produces one atom of tritium
Problem with fusion is that the teller
ulam and the ways it currently can be achieved needs a high security clearance
You cant just walk in and get all of that thermonuclear bombs research papers and try to port the design to a reactor
No. While there is some overlap, creating a thermonuclear bomb and a fusion reactor are two unrelated tasks.
As someone who worked briefly on Fusion in the 1980’s, I’ve always taken an interest in the progress. I have to admit I had assumed we would be closer to full fusion power by now, driven by the optimism of those I worked with (most who have probably retired without fulfilling their goal. )
However looking back, it was never the physics is holding us back. Yes there are some areas like plasma physics that needed extending (many people forget that in trying to achieve fusion, many other areas of physics have benefited), but the main obstacle was in engineering.
The timescale for advances in those areas were probably underestimated. Things like material science, magnets etc needed improvements, but those are never quick. However the technology has come a long way in a slow incremental fashion. We have capabilities that we could only dream of in the 80s. As an example the computers we worked on were PDP-11’s which had the kind of processing power which a mobile phone user would sneer at. One of my jobs was to simulate a magnetic confinement using banks of 555 timers, today the same job could be done on a pi pico. The ability to model and simulate is light years ahead of where we were, meaning less need to build physical devices
Things like superconducting cables, high magnetic fields, alloys and composites again are today the kind of things that I read in science fiction during the day
Therefore I am confident we are almost there. The hard yards in places like JET have been done. The models are available, what is required is that one more push. But engineering like this is a challenge and time consuming, and will take time. However like most things, once it has been proved it can be done, it is easy to repeat
The question is often asked why fusion, not fission? I have no problem with either, but fusion will always have one big advantage over fission in that it cannot be used in the secondary role of making bomb material. If we are to solve climate change, it is not enough for a few countries to have access to such technology, it needs to be used worldwide. This is something that Fission reactors will never be suitable for. Therefore to ensure we have a baseload to support renewables, for me Fusion is the only real long term solution
Less of a waste problem as well.
This kind of research is extremely valuable, and always worth it, no matter how long it takes, because, as you mentioned, it benefits many other areas of physics, but also of science in general.
Fusion has a *huge* proliferation problem. It will require enormous amounts of Li-6 in order to produce the tritium fuel, both of which are highly strategic materials.
This is also the reason tokamaks will never be the basis of commercial power production, there is simply no way the required amount of fuel can ever be produced economically.
Sounds like you know details I don’t. Tell me more. What I’ve been reading is that deuterium is available in sea water at 1.1 g/m^3 and tritium will be breed and reused on site without a waste product. I understand there are e.g. only 36 kg of tritium in the world right now but I believe several outfits have plans for making it in situ. I think e.g. Helion if I’m not mistaken. Not every reactor even uses tritium of course… but it sounds like the majority do. Sounds like you’re talking about an isotope of lithium as a necessary catalyst? I don’t know much about it… Have you seen though, that e.g. there are large lithium deposits recently identified in Pennsylvania? Also I hear there’s a lot of lithium in coal ash or something like that. I read it a few days ago…
Nice post thanks for it. We see the situation alike.
>The ability to model and simulate is light years ahead of where we were, meaning less need to build physical devices
The W-7X is based on physics modeled on relatively modest computer hardware in the 90’s. That’s 30 years ago. The predecessor W7-AS was completed in 1988 and that was the last design they really did “by hand” without the help of computer simulations.
The W 7-X was supposed to be completed in 2006 but it slipped a further 9 years due to the meager funding and slow work. The inefficiency of having basically no money, and the misdirection of most of the available funds into political “superstar” projects like the ITER, is what has caused decades of delay in the field even as we have gained the tools actually pull it off. We could already be on the third generation of stellarators right now, trying to burn actual fuel plasma.
I look forward to a time when people finally wake up and see the fusion reactor Game for the bureaucratic con it really is.
Billions have been siphoned off into dead end projects that could have gone into genuine plasma physics resesrch.
ITER will cost 60 billion euros over its lifetime and will never produce a watt of net power by design.
The simple question is: where is all the tritium going to come from? Or even the Li-6 required to manufacture that tritium?
ITER management have dropped the plan to test out tritium breeding, the only part of the project which could have advanced fusion power generation research. This is because they tacitly admit it is not feasible.
Stop allowing this charade to contiune.
100 million degrees C? That’s impressive! … Are you sure it isn’t merely 100 million degrees K? 100 million degrees K obviously wouldn’t be nearly as impressive. :)
Are you serious? 100 million degrees K is simply 100 million degrees C minus (roughy) 273 =roughly 100million degrees K
The reason the sun can contain a continuous fusion reaction is due to a magic force called: GRAVITY!
I have doubts that magnetism can achieve the same results as gravity. There are no confinement walls around the sun to damage from instabilities in the plasma field. I think the solar plasma instabilities are called “Solar Flares”, and they too are reasonably controlled by the wonderful magic of: GRAVITY!
Find a way to artificially generate gravity adequate to contain the reaction and you might have something.
It’s weird to me that this article doesn’t mention well funded startups like Commonwealth Fusion (started by really good people from MIT), Helion, or any of the others (quite a few) currently working on commercialization. I think they’re all very serious companies. The biggest breakthrough in recent decades in the field has been the development of very high field magnets using high temperature superconductors, which is what most of these startups are exploiting. Commonwealth’s SPARC design, for example, is based on a tokamak using extremely high fields.
I work for one of the startups. It appears there is actually quite a lot of interesting engineering space that hasn’t been very well explored, with a lot of positive hints about future directions to take.
The good news amongst all us engineers and scientists in the fusion field, is that when one of our teams cracks the code to plasma stability problems, it’ll likely enable all the other team’s machines to function, albeit at different efficiencies due to engineering/topology choices.
We humans still need to consume less, abundant power is only one part of the puzzle.
I wonder why no one ever seems to know about Helion…