By the end of World War II the world had changed forever, as nuclear weapons were used for the first and – to this date – only time in anger. Although the use of these weapons was barely avoided during the Korean War in the early 1950s, the dawning of the Atomic Age had come in the form of obliterated cities and an increasing number of these weapons being test fired around the world. It was against this background that on December 8, 1953, US President Dwight D. Eisenhower held his ‘Atoms for Peace’ speech, during which he would not only promote the peaceful use of nuclear technologies but also lay the groundwork for what would become the International Atomic Energy Agency (IAEA), as announced in the full speech.
Under the Eisenhower administration the US became one of the world’s nuclear power pioneers, as it competed with the UK and later others in establishing world’s firsts in commercial nuclear power. Dresden Generating Station would become the first purely commercial boiling water reactor (BWR) in 1960 and Yankee-Rowe, the first pressurized water reactor (PWR) in 1961. Following these, the number of new reactors planned and constructed kept increasing year over year, setting the trend for the few decades of the US nuclear power industry.
Today the US operates 94 reactors, which generate nearly 20% of the country’s electricity. Exactly how did the US build so many reactors before 1990, and how does this compare to the recent revival with both new builds and retired plants being put back into service?
From Graphite Pile to Light Water Reactor
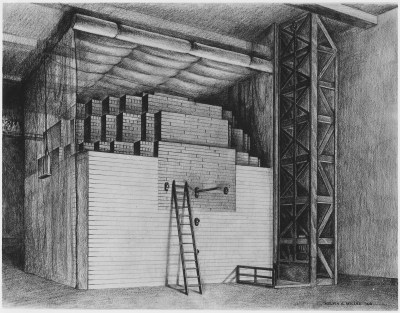
The first artificial nuclear reactor was the Chicago Pile-1, demonstrating the first human-made, self-sustaining nuclear fission reaction. This laid the groundwork for the second generation of nuclear fission reactors with commercial aspirations, the overwhelming majority of which are light water reactors (LWRs). These use water as both coolant and neutron moderator. When artificial fission reactors were being developed, it had already been established that in order to fission uranium-235 (U-235), moderated (thermal) neutrons were needed.
Moderating neutrons effectively means slowing them down using a material that interacts with but doesn’t capture neutrons. Heavy water (deuterium, or D2O) was a known moderator in the 1940s, as was graphite, but it was easiest and fastest to assemble a graphite pile reactor, with the uranium interspersed throughout the graphite blocks. Naturally, this meant that CP-1 had no means of cooling itself and only low power output, but it sufficed to test many theoretical assumptions experimentally.
Interestingly, the US and Canadian reactor designs diverge here. Canadian engineers, in their Generation II reactor designs, went with heavy water in the pressurized heavy water CANDU reactor (PHWR), as this allowed for the use of unenriched uranium as its fuel. While light water (H2O) is also a neutron moderator, it absorbs a significant number of neutrons, which requires enriched uranium (<5% U-235) and the resulting increase in neutrons to compensate for so that a chain reaction can even commence. Even so, US engineers opted for the much cheaper ultra-pure light water as an acceptable trade-off.
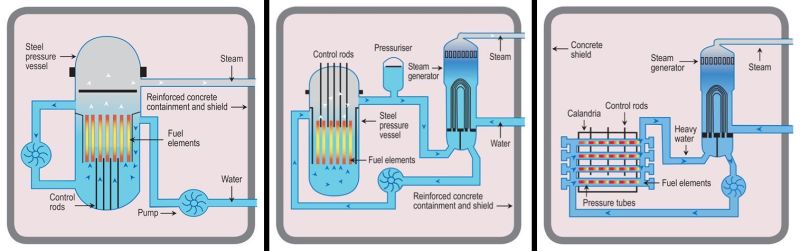
The two basic types of LWR that still form the backbone of the US nuclear fleet today are BWRs and PWRs, which differ primarily in their complexity. In a BWR the steam is generated in the reactor pressure vessel, from where it travels to the steam turbine before hitting the condenser and returning to the pressure vessel. A PWR separates this into two loops: the (high-pressure) primary loop where the water (heavy water in the case of a PWHR) is heated but not allowed to boil, and a secondary loop which the thermal energy is transferred into by the steam generator before heading to the steam turbine.
Unsurprisingly, a PWR is more efficient than a BWR due to the high-pressure primary loop, but BWRs have one advantage in that there’s less latency between the heat production and the conversion into electrical power. This makes BWRs better at load-following when integrated into a local grid, though this advantage has become less pronounced over the years with newer PWR designs and more efficient grid-storage solutions.
The reactivity of the reactor core is regulated with control rods, which are made out of a material with a large neutron absorption cross section, the ideal type of which depends on the design of the reactor core, but both cadmium (Cd-113) and hafnium (various isotopes) are popular options here. With all of these in place and a control scheme developed to keep the chain reaction occurring at an optimal pace, the next step is to start the reactor. Since the fresh reactor fuel won’t do this by itself, a startup neutron source is required, such as californium-252 or plutonium-238. This source is inserted at the beginning of startup and removed after successful commencing of the reaction.
With these designs designed and prototyped, all US engineers had to do now was to build them in large quantities to power the rapidly increasing electrical demand of the 1960s United States.
Mass-Produce It
How do you scale up building nuclear reactors from a few handcrafted prototypes and demonstrators to hundreds of units? Perhaps unsurprisingly this is done in much the same way as any other large-scale infrastructure project. Many parts of a nuclear power plant (NPP) are the same or similar as in a coal- or gas-fired plant, as they are after all still thermal plants, just with different ways to create the hot steam or air that makes the turbines spin. This made things quite easy for US nuclear engineers who could mostly focus on the part that makes a nuclear plant different from a coal-fired power plant: the reactor and steam generator instead of the boiler. As a bonus, an NPP doesn’t need a smokestack, coal or ash hopper, coal mill, exhaust scrubber and countless other parts that come with burning tons of coal every single day.
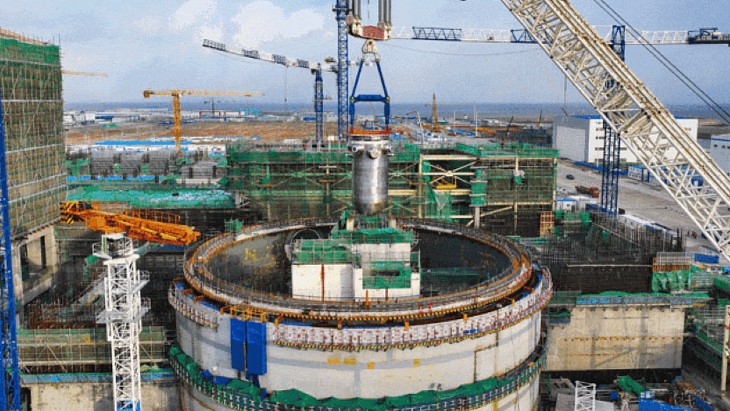
The beating heart of an NPP is the reactor pressure vessel and its control mechanisms. These parts have to be of certain alloys and of a high quality level so that they are resistant to e.g. neutron flux and corrosion while inside the reactor for decades. With the research and development finished, once the production lines are in place and the workers hired and trained, all one has to do is to simply keep producing the steel and other components, while continuously transporting new pressure vessels, steam generators and everything else to the sites of new NPPs.
Having as few distinct reactor designs is incredibly helpful here, with General Electric’s BWR line of reactors taking the lion’s share of BWR construction in the US and around the globe. For PWRs, Babcock & Wilcox provided many of the designs (e.g. LLP, WH 4LP), along with Westinghouse.
Much as we can see today with the nuclear fleet build-out in China with the Westinghouse AP1000 and derived designs (CAP1000 and Hualong One), strong political and financial backing combined with a mature nuclear power supply chain means that the time from first concrete to grid connection can be as little as four to five years. After all, the components are made in parallel, so that no single step in the construction is blocked until final on-site assembly occurs. Meanwhile, experience is collected with each newly constructed reactor that helps to speed up and improve subsequent builds.
As a demonstration of this, a recently released report by the US Department of Energy (DoE) shows the massive time improvement for key milestones between Vogtle units 3 & 4:
Vogtle unit 3 was the first time the new AP1000 reactor was built in the US, completing its construction long after the Chinese nuclear sector had not only built a number of licensed AP1000 units, but also designed their own version that better fit the Chinese market and built a number of these both in China and abroad. The difference is that the US is now cobbling its nuclear industry back together after a decades-long hiatus – much like it did in the 1950s and 1960s – while China began building nuclear reactors in the 1990s and never quit building them.
The problem with institutional knowledge is that it’s costly to obtain and basically impossible to retain if you do not actively maintain it. When the US began building its nuclear fleet last century, this knowledge was strong and supply chains robust. During the 1980s and until recently, both were allowed to degrade, as engineers and workers retired or died, suppliers changed markets or went bankrupt, documentation was lost and tooling was scrapped.
Back From The Dead
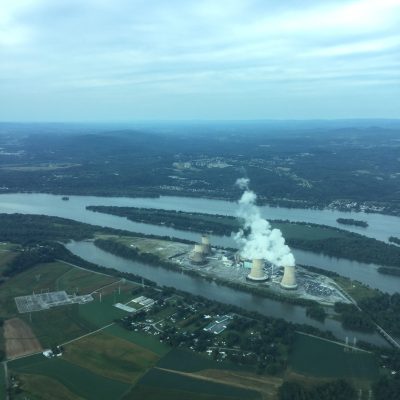
The current revival of the US and effectively the Western nuclear power industry poses many challenges, as much of this institutional knowledge has to be relearned and rediscovered. As the economics of the energy market change, and financing options become available with the scrapping of various anti-nuclear power regulations, there’s now a surge in interest among companies and investors in not only new builds, but also in reviving units that were already turned off and put into decommissioning status.
Prime examples of this are the Palisades NPP in Michigan and Three Mile Island unit 1, the former of which was originally transferred to Holtec for decommissioning, but which decided to refurbish this NPP instead. In the case of TMI-1, this reactor was still running until 2019, but is now being refurbished by the owner due to a much friendlier political and investment climate for nuclear power.
Returning a nuclear reactor from a decommissioning state back to an operational one is pretty much an abbreviated case of constructing one: every component has to be inspected, with missing, damaged or otherwise unsuitable components replaced. Following this the typical commissioning procedure has to be followed, with cold pressure tests, hot pressure tests, fuel loading and gradual increasing of the power output through a set testing protocol. Depending on the level of damage and number of systems that had to be upgraded to current standards, this could take around 1-2 years.
In the case of Palisades, the expectation is that it will resume operations by late 2025, and for TMI-1 it will likely take until 2028, mostly due to environmental reviews, emergency and security protocols and getting the appropriate permissions from the NRC. Meanwhile the Duane Arnold NPP in Iowa may also be restarting, per recent news, and the V.C. Summer NPP in South Carolina may see its partially built AP1000 reactors finished after all, assuming the necessary legal and regulatory hurdles can be cleared.
Although the US has in the past demonstrated that it can build many nuclear reactors fast and safely, it would appear that the biggest obstacles are primarily a lack of political willpower, an atrophied nuclear industry and an abundance of red tape. Whether these can be overcome remains to be seen, but the successfully running AP1000 GenIII+ PWRs in China, South Korea, and the US demonstrate that the problem never was US engineering chops.
RBMK is still 70% less expensive to build and operate than AP1000. Just don’t let scrum masters operate it.
Probably safer to let a SCRAM master operate them. :-)
There were so many stories in the news around here about cost overruns at Plant Vogtle that I kept confusing the name with a similar sounding German obscenity. Still, I think it was money well spent to get the US nuclear program back on track. Somebody had to go first and I wasn’t surprised that it cost more than they thought.
That one single…event…definitely put a major dent in the cost comparison…
Not really. It has great emotional weight, didn’t really do much overall damage compared to other industrial accidents. Total hippie death btw
Also watch out for the (now ubiquitous) H1B infiltration and hiring nepotism schemes. Wasn’t a problem the first time we developed this knowledge, back then it was mostly O1 visas
it is never engineering chops. but knowledge, risk assessment and focus scope need to be on par as well. technicall is is a solved puzzle, but the danger lies elsewhere: its society and the fragility of it. thats the elephant in the nucleair porcelain shop.
A fallen, degenerated society was always going to inherit all of our scary powerful stuff. Not a valid reason to refuse to do anything greater than tilling turnips as a domesticated ape.
We’ve seen the cycle of empires before and we honestly don’t have anything worthy of being called apocalyptic yet, despite the (historically constant) hysteria
Something else about the design of the CANDU reactors, the “core” is an a calandria (bunch of tubes) is because at the time Canada didn’t have the industrial capacity to build the massive containment vessel needed for American style reactors. It worked out alright in the end though because it let the CANDUs be refueled online, which most LWRs can’t do.
I was going to make a comment, but on a second thought I think I’ll better keep quiet about what I think about all of this.
Yes, probably best . . .
I’ll do the same!
The important part is that you told everybody about your restraint! Otherwise you’d get no credit
It’s quite important actually.
Voicing dissent is necessary.
Argument is not.
Furher, humans are greedy, nasy, violent goblins. We have to be taught to behave in a group(not everyone is taught, and some don’t learn.)
Doing good DESERVES praise.
Assuming people will just do he right thing is doing them a disservice.
They had to expend effort, even if it was trivial.
So what if someone did the right thing he last 100 times?
If the same person holds the door open for you every day when it rains, is there some cutoff point where you stop thanking them?
Celebrate when someone does a good.
It’s rare nowadays…
“… competed with the UK and later others in establishing world’s firsts”
weird way to spell “USSR” but OK
No mention of waste management – obviously not solved politically or socially, but are there proven stable long-term technical solutions?
Let’s better don’t talk about it, people with confirmation bias likely can’t be helped.
Smarmy, reddit, says essentially nothing while pretending to be wisdom, ignores that the sole purpose of a comments section is to get into it (why not simply delete the comments section? Is it kept around only for purposes of boosting engagement numbers? It would be more honest to come out and say this)
I just meant that such a discussion is likely futile.
I’ve tried to discuss such things objectively in my own family and it did cause nothing but dissonance.
So if the given topic itself has a somewhat positive rationale about something, then it’s hard to say anything else.
Finland has built a long-term nuclear repository. I don’t think anyone else has in the entire world.
Here in the US, we built Arizona and New Mexico for the same purpose.
Yes. You dig a really deep borehole in the middle of a tectonic plate and put it in there and cover it up.
Yes. Waste is a solved problem. Most waste is low level and will lose the majority of its radioactivity within 30 years. Some high level waste is able to be reprocessed back into usable fuel. The rest can be isolated in various ways. Some of which are retrievable while others are not. The main thing though is that new reactor types very much limit the amount of waste generated.
“Fleet” is not the right word. Sorry!