The basic technology of radio hasn’t changed much since an Italian marquis first blasted telegraph messages across the Atlantic using a souped-up spark plug and a couple of coils of wire. Then as now, receiving radio waves relies on antennas of just the right shape and size to use the energy in the radio waves to induce a current that can be amplified, filtered, and demodulated, and changed into an audio waveform.
That basic equation may be set to change soon, though, as direct receivers made from an exotic phase of matter are developed and commercialized. Atomic radio, which does not rely on the trappings of traditional radio receivers, is poised to open a new window on the RF spectrum, one that is less subject to interference, takes up less space, and has much broader bandwidth than current receiver technologies. And surprisingly, it relies on just a small cloud of gas and a couple of lasers to work.
Quantum Music using Rydberg Atoms
The term atomic radio seems a bit confusing at first. After all, aren’t all radios made from atoms? But in the context of differentiating traditional radio technologies from the newer approach, use of the term atomic makes sense. Atomic radio relies on Rydberg atoms, which are atoms of elements such as cesium and rubidium that have had their outer electrons coaxed into much, much higher quantum states than normal matter, using either laser light at exactly the right wavelength or other electromagnetic methods. The electrons are so far from the nucleus in Rydberg atoms that they are barely held in orbit, the orbits are nearly circular, and the atom approaches macroscopic size.
Because of the highly excited outer electrons, Rydberg atoms have interesting and useful properties. The spacing between electron levels that far out from the nucleus is extremely narrow, making it very easy to perturb electrons and make them change states. If, say, a passing radio wave hits a Rydberg atom, its outer electrons can be nudged to another level. What’s more, Rydberg atoms show the nonlinear optical property of electromagnetically induced transparency, or EIT. That means that a laser tuned to a specific frequency can saturate a gas of Rydberg atoms, rendering them optically transparent in a narrow slice of the spectrum. A second laser tuned to that optical window will shine right through the gas with little loss of intensity.
Atomic radio combines these two properties: a small cell full of excited cesium vapor is rendered transparent with a laser tuned to 852 nm. The cell also has a laser at 510 nm passing through it to a photodiode. When a microwave signal is transmitted through the cell, the vapor’s transparency is reduced proportionally to the strength of the incident radio wave, which results in a signal from the photodiode that can be amplified. This means that unlike conventional radio antennas that operate electromagnetically, atomic radio directly detects radio waves optically.
No Static at All
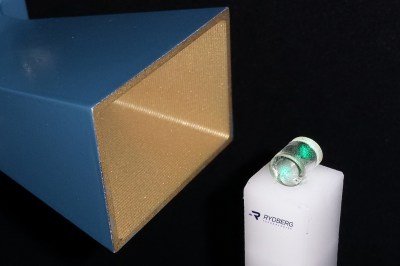
In a way, atomic radio hearkens back to the aforementioned days of spark gap radios. The earliest radio receivers used coherers to detect passing radio waves. Coherers were simple glass tubes filled with iron filings that had metal contacts at each end. Under normal conditions, the iron filings would not be very conductive, owing to insulating oxides creating a high-resistance path through the loosely packed particles. But a passing radio wave would cause the filings to clump together, reducing the resistance through the coherer and causing it to conduct. The radio wave’s passage could be indicated with a bell or a light.
Of course, coherers need a device called a decoherer, which was essentially a solenoid to gently tap the tube and reset the iron filings to a loose, non-conductive state. An atomic radio receiver needs no such resetting, and as recent work by David Anderson, Rachel Sapiro, and Georg Raithel shows, it is capable of receiving modulated signals, both AM and FM, which coherers were not. Still, the analogy is apt.
The simplicity of atomic radio is attractive. There are no tuned circuits, no intermediate amplifiers, no RF mixers, and critically, no antenna in the traditional sense. Radio waves are detected directly based on how they interact optically with the Rydberg atoms. This means that the stages of a traditional radio receiver that are subject to picking up interference are not present in an atomic radio, resulting in a much lower tendency to pick up noise. And because the Rydberg atom vapor is sensitive to a wide range of radio frequencies, an atomic radio is a broadband receiver — the one demonstrated in the above paper had a four-octave range, from the C-band to the Q-band, or 4 GHz to 50 GHz.
Living in Stereo
More recently, Christopher Holloway et al at the National Institute of Standards and Technology demonstrated an atomic radio that can receive two signals at once. The vapor cell in this radio contained a mix of cesium and rubidium, and each channel required two lasers — one to saturate each species to transparency, and one to probe for RF. Two guitars were played into audio amps (the authors took pains to note that the amps had gain knobs that go to 11) which were used to modulate two microwave signal generators at different frequencies in the 20 GHz range. The probing lasers were directed through the cell and into two separate photodiodes using optical splitters, resulting in a stereo atomic radio.
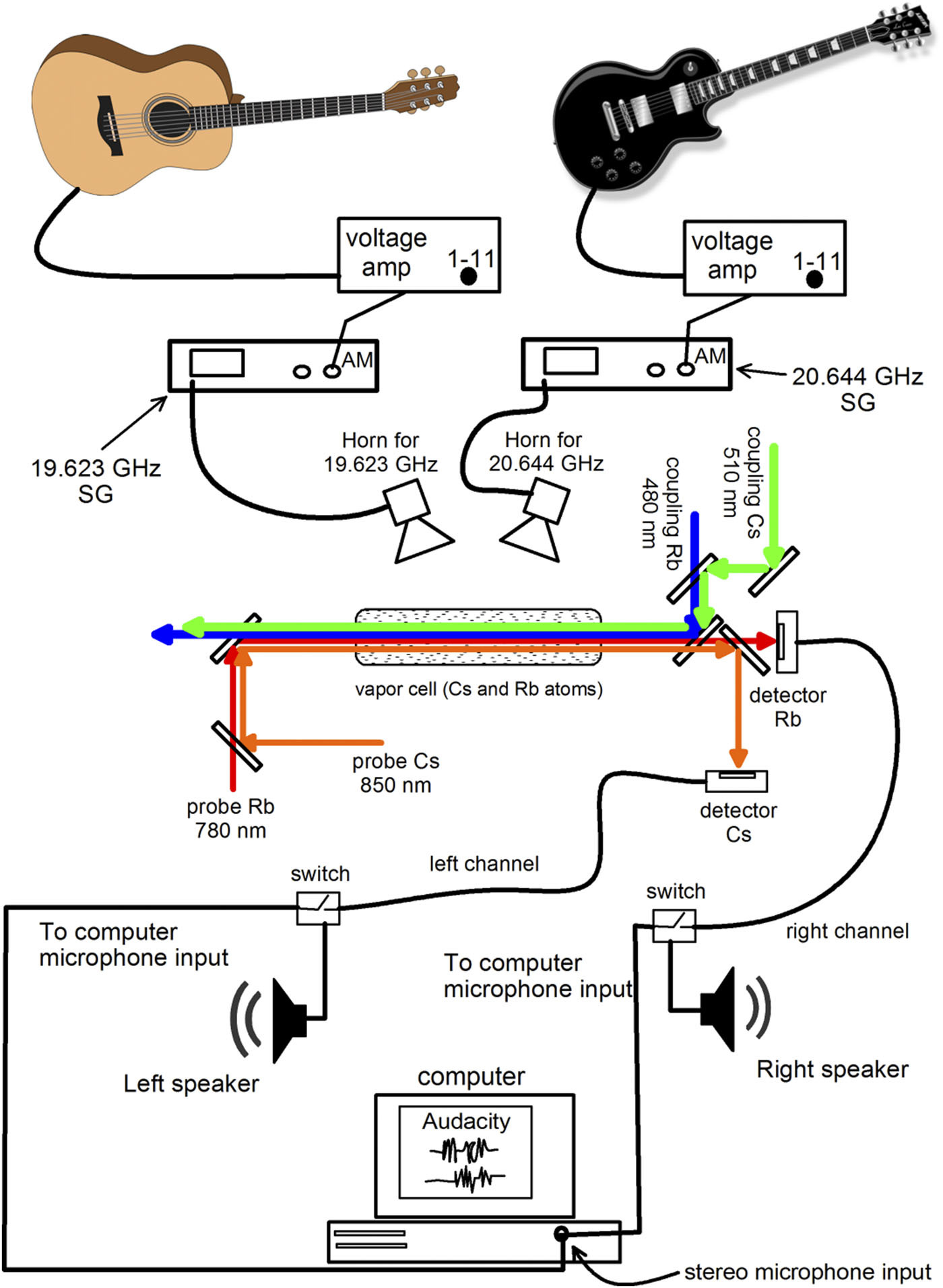
Atomic radio is at once both simple and complex — simple in that it bypasses most of the traditional circuitry of radio receivers and detects radio waves directly, but complex because it takes a physics lab full of lasers and optics to make it work. So it’s not likely that rank-and-file hobbyists will be building their own atomic radios anytime soon, and hams won’t be tearing down their half-wave dipole antennas in favor of cesium vapor cells. But atomic radio has a lot of potential, especially in deep-space communications applications, and if it can be miniaturized sufficiently, we just might see another commercialization of quantum theory.
“… but complex because it takes a physics lab full of lasers and optics to make it work.”
I suspect we’ll be able to shrink that down since it doesn’t take a lot of that cloud to work.
Oh, absolutely. I can see the potential for this being miniaturized down to the chip level at some point. That’ll open up some real Star Trek applications – I’m looking at you, commbadge…
So when can I but the new Motorola Lazr?
Right after Motorola starts building things again.
Interesting article, thanks Dan!
New stuff for me.
You’re welcome! I for one look forward to the day that old hams complain about them youngsters with their fancy atomic radios. “Back in my day we hung wires from trees and worked all continents, and we liked it that way!”
Working all continents between 4 and 50 GHz would be quite the feat!
Well, you would do it via satellite relays as those frequencies are strictly LOS.
I suspect you’ll still need those wires for transmitting. At least for a while…
How good is it really at receiving signals?
Very I would imagine. Rydberg atoms are very sensitive to radio waves disturbing their electrons, which is what makes this work. The SNR would be fantastic because there are no additional stages with traces or inductors to pick up any interference.
It still has that crucial source of noise, a diode and amplifier.
I suspect that Johnson–Nyquist noise would not be the limiting factor, so extremely sensitive would be my guess.
Although after speed reading their paper, I do hope things have progressed “While the achieved dynamic range between 20 dB and 30 dB falls slightly short of radio standards,we see considerable room for improvement of our demonstration unit.”
Because 20 to 30dB of a dynamic range, with extreme sensitivity would limit applications.
My question too. I mean, a paperclip is an antenna too.
My favorite paperclip LTE antenna: https://youtu.be/i2h2DbwIeRM
It would be better to call this a detector not a complete radio. What comes out of the diode is the same as any other radio. Now much more processing is needed to extract the desired signal.I would call it a microwave plasma detector without a horn antenna. Those IF strips do a lot of work. The stereo demo is redundant, as many channels of audio can be multiplexed in this wideband mode. More like polarized antennae waves.
“There are no tuned circuits, no intermediate amplifiers,”
“This means that the stages of a traditional radio receiver that are subject to picking up interference are not present in an atomic radio, resulting in a much lower tendency to pick up noise”
“an atomic radio is a broadband receiver — the one demonstrated in the above paper had a four-octave range, from the C-band to the Q-band, or 4 GHz to 50 GHz”
Yeah, so for a practical receiver, you’re still going to need those pesky tuned circuits and intermediate amplifiers to filter out all the signal in that range you DON’T want….
I agree wire Hiro. This doesn’t make a lot of sense, unless it’s explained in an erroneous and inexact way.
Tuning the laser tunes the atomic receiver. You don’t get all freqs at once, just what you tune for. It’s detailed better in the research paper.
Exactly. If an ultra-wideband receiver with no tuned circuits has a lower tendency to pick up noise because it doesn’t have all those pesky traditional stages, then why not just try a conventional radio receiver without all those extra tuned circuits and narrow filters and see how much noise you don’t pick up.
And I know just sdr architecture but you said traditional radio receivers. Sdr works better with bandpass filters and tuned circuits presumably picking up noise so how do you explain that?
The definition of noise is unwanted signal so unless the signal you’re after is really 46GHz wide and coming down a pipe so that you’re in control of external signals, there’s going to be a lot of noise in there.
Isn’t there?
I didn’t know Tesla was Italian!
Marconi invented wireless communication
If you thought the sdr nerds were intolerable before… great read tho. cheers
Just in time for 5G!
“Coherers were simple glass tubes filled with iron filings that had metal contacts at each end.”
I thought they were nickel, Wikipedia says a mixture of nickel and silver powder. Another source says the Marconi version was nickel powder with 4% silver powder and a trace of mercury between silver electrodes hermetically sealed in glass through platinum wires. Iron does work, so apparently do a lot of other metals, copper, aluminium, silver, brass, zinc, also graphite.
I think you need some new nomenclature. If you are writing about quantum processes, “detected optically” is a macro process and not very descriptive. The interaction of photons and electrons is Quantum Electrodynamics. Maybe call it Electrodynamic Detector? Or perhaps QED Radio? SuperFeynmandyne Receiver (my favorite)?
QED radio?
Quite Easily Done!
B^)
Yeah, I think its the Fabry-Pérot Interferometers that are on a chip now. Not sure about Michelson and other interferometer chips available main stream yet. There are definitely MIMS systems.
Kind of a tangent, though I’ve lately been wondering about why no one has made a wideband laser interferometer receiver yet. Seems simple and cost effective with either a high speed camera or even better higher refresh rate sensors in a Cartesian grid, especially with the last two posts regarding cost effective interferometers. The webcam will have a fps issue… so ideally the highest speed camera is best. Photosensors seam cheaper and obviously faster relaxation/excitation rates components to detect higher frequencies easier. Wondering what the highest frequency cost effective sensor component is? Thermal diodes can go to the THz range and thinking photodiodes might… though the more I think about it… they’re usually used in pulse modulation systems in the kHz range… so are most likely not the optimal candidate. Ideas?
Definitely more feasible than the Rydberg atom vapor cell for the laymen; since I’m not sure what the best isotope cesium and rubidium is used even (I guess stable common forms) and I’m not sure how many glass blowers would want to attempt. Then again, most skilled probably will with compensation from my experience so, may be easier than most realize.
Interesting, however in any scenario the detectors limitation on frequency detection capabilities.
No one else has said it, so let me be the one to officially call these this the “Atomic Coherer”
SuperFeynmandyne detector.
Neither was Alexander Popov.