The lead-acid rechargeable battery is a not-quite-modern marvel. Super reliable and easy to use, charging it is just a matter of applying a fixed voltage to it and waiting a while; eventually the battery is charged and stays topped off, and that’s it. Their ease is countered by their size, weight, energy density, and toxic materials.
The lithium battery is the new hotness, but their high energy density means a pretty small package that can get very angry and dangerous when mishandled. Academics have been searching for safer batteries, better charge management systems, and longer lasting battery formulations that can be recharged thousands of times, and a recent publication is generating a lot of excitement about it.
Consider the requirements for a battery cell in an electric car:
- High energy density (Lots of power stored in a small size)
- Quick charge ability
- High discharge ability
- MANY recharge cycles
- Low self-discharge
- Safe
Lithium ion batteries are the best option we have right now, but there are a variety of Li-ion chemistries, and depending on the expected use and balancing and charging, different chemistries can be optimized for different performance characteristics. There’s no perfect battery yet, and conflicting requirements mean that the battery market will likely always have some options.
How a Li+ion Works
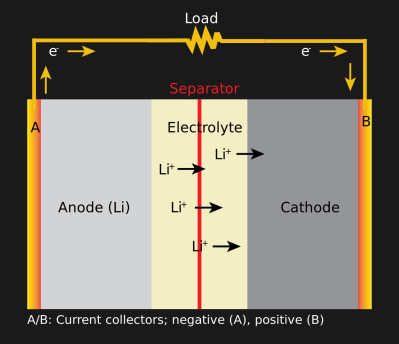
All batteries work the same way. There are 3 components: an anode, a cathode, and an electrolyte. A chemical reaction between the electrolyte and the electrodes (the anode and cathode) creates ions near one part of an electrode and electrons on the other, giving the two terminals a difference in potential. The two electrodes are made of different materials. The anode is graphite bound to copper, and the cathode is some lithium crystal bound to aluminum. The electrolyte is a sort of insulator, so the electrons are better off going through the circuit from one electrode to the other than to make an internal short. Once the reaction is complete, the battery is dead, and the reaction won’t happen unless there’s a path for the electrons to move (aka a closed circuit). To charge a battery, the process is reversed, and power applied to the electrolyte undoes the chemical reaction. Not all electrolytes are created the same, however, and the chemistry of a non-rechargeable battery means that it can store more energy, but applying power in reverse doesn’t undo the chemical reaction.
It’s best to maximize the battery by taking advantage of surface area, so the anode/electrolyte/cathode sandwich benefits from being as thin as possible with lots of area touching. Also, the sandwich has a few more slices of materials with porous layers between them to allow ion transfer without allowing material migration. Now take your battery sandwich and put a bunch of them together in a stack with separators, and you have either a pouch battery (cheap cell in a silver casing), a prismatic battery (fancy cell that you’d find on a laptop), or roll it into a small tube and you have a cylindrical battery (like the 18650 or AA).
The Million Mile Battery
You may have seen the news recently about Tesla’s Million Mile battery. It was actually a group of researchers from Dalhousie University in Halifax, Canada under contract with Tesla, but they did a LOT of testing of a variety of Li-ion batteries to find the best chemistries and use profiles and charging profiles. The million mile battery is just a good PR term to describe the research that optimized some battery formulations and resulted in much longer lifetimes. The full paper is filled with technical jargon, so I spent the weekend learning all about batteries in order to distill it here. You’re welcome.
The first thing to note about their million mile formula is that it doesn’t represent most current drivers that identify as average commuters. Rather, they’re targeting the use cases that will use the vehicle almost constantly and charge when the battery is empty. This would be for long-haul semi trucks, taxis, and buses. Their term was 100% DOD, or Depth of Discharge, where they run the battery all the way to empty before charging it back up again, unlike a cell phone which is usually plugged in every night no matter the state of charge.
Findings: Batteries Like it Cool; Hot New Chemical Formulations
They found that temperature matters a lot. A battery that spent most of its life at 20ºC resulted in a longer lasting battery than at 40ºC, but a battery that spent time at a high temperature and then went to a lower temperature then lost its capacity at the same rate as other low-temperature samples. In other words, a cell at higher temperatures loses capacity faster, and a cell at lower temperature still loses capacity over time but not as fast, and a single battery can move anywhere on that line without memory. The lower temperature meant less degradation at a molecular level, with fewer cracks, dendrites, gas pockets, etc. They couldn’t stress enough how important it was to keep everything cool.
The research team spent a lot of time in previous studies looking at other chemistries, but had primarily settled on NMC532/graphite as their electrodes (as has most of the research community). In chemistry terms, NMC532 is another name for LiNi0.5Mn0.3Co0.2O2, and in lay-speak it means the cathode is mostly lithium crystals, with a sprinkling of nickel, manganese, cobalt, and oxygen, and the anode is graphite (though research into graphene is promising).
Calling the battery NMC532/graphite isn’t quite sufficient, though. You still need to specify an electrolyte. The electrolyte is a slurry of LiPF6 and solvents and additives with fun names to say out loud, like dimethyl carbonate and ethylene sulfate. In this study they tried out a few combinations of solvents. The additives can also change the performance of the cell, giving it a higher charge/discharge rating at the expense of overall lifetime, or vice versa. Based on previous studies, they were really excited about 2 different additive formulas (2%FEC+1%LFO, and 2%VC+1%DTD), though they discovered that the two had different performances at different temperatures, so they suggested that the choice of additives could be application-specific. In the production of cells, usually the dry packs are manufactured, and then the wet electrolyte slurry is injected. See Sparkfun’s tutorial tour through a manufacturing plant for a visual story of the process.
With this special formula, and maintaining a low temperature, they were able to minimize the two main things that cause degradation of a battery; lithium inventory loss, and increased impedance. Generally, over time the lithium ions move around and eventually get themselves into locations that aren’t helpful for the battery. They can get electrically isolated, they can group together into plates and dendrites and surface films, and they can react with other components of the cell and become unavailable for charging/discharging. The dendrites are particularly bad because these sharp little lithium needles can pierce the separators, causing a short circuit in the cell, which then heats up and causes a runaway reaction that ultimately explodes. Impedance is increased through electrode corrosion or just in general less surface area being available, either through reactions or cracking or resistive surface layers forming and blocking the electrode.
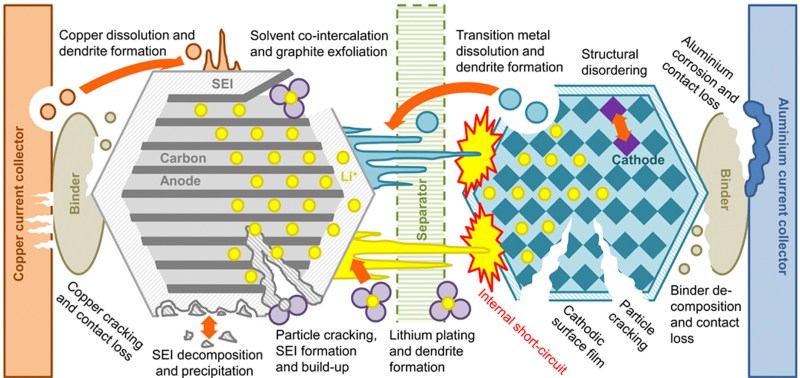
One of the reasons this study got so much attention is that is was thorough and entirely open. It took them 3 years, running thousands of cycles on each battery, with extremely accurate chargers and dischargers to record the capacity, to get the most complete data they could. Usually accelerated lifetime testing on batteries is difficult, as it’s still putting the battery at higher charge/discharge rates than expected in real life, with less recovery time, so the fact that they put so much time into this testing means the results are more realistic. They also specifically said:
Full details of these cells including electrode compositions, electrode loadings, electrolyte compositions, additives used, etc. have been provided in contrast to literature reports using commercial cells. This has been done so that others can re-create these cells and use them as benchmarks for their own R+D efforts be they in the spaces of Li-ion cells or “beyond Li-ion cells”.
It’s refreshing to see commercially funded research made publicly available, and Creative Commons no less.
Despite its openness, we probably won’t be seeing homebrew Li-ion batteries soon (but we’d love to cover it if any of you try). From the battery application community we may be seeing battery chemistry change towards the formulation suggested, and we’ll likely see a lot more attention paid to cooling, as that significantly improves their life. And we’re pretty certain that if you want a battery pack, Tesla will soon be happy to sell you one of theirs from their gigafactories.
Author’s Note: Some people pointed out that I used the term LiPo when I should have used Li-ion. They were, of course, correct, and I’ve updated the article accordingly.
“High energy density (Lots of power stored in a small size)”
Power and energy density are not the same thing, and the two break down further into volumetric and gravimetric energy density. LiPo-s are high in power density, but Li-ion packs more energy in a smaller volume and less mass.
The NMC cells tested by Tesla are not LiPo, but Li-ion.
The difference is that LiPo uses a solid polymer electrolyte, whereas Li-ion uses a liquid solvent electrolyte. Hybrid versions exist where the electrolyte is stabilized into a gel.
The point of the solid or gel electrolyte is the ability to enclose the battery in a pouch rather than a cylinder, because the electrolyte doesn’t run off and pool somewhere.
Also, one of the problems of the lifespan study is that they use linear extrapolation to predict the calendar life of the battery, assuming that both the cycle life loss and the calendar life loss are independent, while it is known that one affects the other: the side reactions in the battery increase the aging rate, and the impedance growth increases the side reactions. This results in a compounding effect where the battery wears out slowly at first, but then faster and faster as it gets older and is used up more.
So predicting a 20+ year lifespan is easy – if you use the battery very little or not at all. The earlier studies quoted in the paper had observed relatively little capacity loss over almost 12 years, but the battery was cycled only 750 times during those years – a very low stress of gradually charging and discharging the battery over several days.
Likewise, predicting a million mile cycle life (~3,500 cycles) is easy if you manage to use it all up in a short span of time (3 years). Achieving both a million miles and 20+ year calendar life – not so easy – even if you keep the temperatures low.
And that’s the main catch for all Li-ion batteries for automotive use. A car is expected to last 20+ years and a reasonable number of miles under temperatures that vary +-40 degrees at worst. So far nearly all Li-ion batteries succeed on the miles, but fail on the years, reaching their end of life around 10-12 years. Whether that can be extended to 12-15 years by incremental improvements doesn’t really matter, because you’re still left with a situation where you own an old EV with a dead battery, that could still have 5-10 years of use in it – and by all right should have – but it costs you half the price of a new car to fix it.
I don’t get why more people don’t give Lithium Titanate batteries a try… Given they are bigger and heavier than LiPo batteries per KWh but they are as forgiving (if not more) than Lead Acid and still significant improve on energy density. The Lithium Titanate batteries I use (Toshiba SCiB) are rated for between 14000 and 7000 recharge cycles depending on charge/discharge rate (the 7000 figure is when your charge rate is at or above 4C. The newer generation boasts 40K cycles at 10C charge/discharge rate before it’s capacity hits 80% of rated). These are worth a look for sure…
Likely because of ESR and round trip efficiency.
The ESR on my YinLong Lithium Titanate batteries is as low as as 1mOhm. Round trip efficiency as calculated by my solar charge controller is pretty good, 93 – 95% including the charger losses.
Overall they’re great batteries, only issue is the lower cell voltage.
“as low as as 1mOhm”
Over what capacity?
The energy and power density are the real killer. A 100 kWh LTO battery would literally weigh a short ton. Tesla NCA batteries weigh in at half a ton, and that’s just about the limit of doable before you have to start building them into SUVs.
Where can those cells be purchased? I found the usual “Go away mortals” Toshiba website for them. I also see that Yinlang cells are available from aliexpress. But Toshiba sounds more fun.
Try and go buy a Tesla pack not in a car or power wall. Good luck because they aren’t sharing
Good thing that other manufacturers are using the exact same chemistries (NMC and NCA) – but not the exact same compromises as Tesla.
buy a car or power wall and tear it down to harvest the cells
that is how rc’ers got the a123 cells from the dewalt nano packs
AFAIK you can already buy TESLA packs, used but still in very good condition, over ebay for a quite good price. They are probably not of best performance for a 500kW car any more, but very well suited for e.g. solar applications
I don’t understand why reverse current charging hasn’t become common for Li-ion cells. Ni-cad battery had (still have) this problem and it was addressed in a NASA article from the late ’60’s.
Dendrite formation in Li-ion cells should be able to be easily controlled in the same way.
Why don’t I see this technique being used?
Do you have a source for this? I’d love to read up on this.
Sounds like a very interesting read! Please share a link.
I’m looking but I’m afraid that my paper copy has been gone for some time.
I’ll check the patent office – there is a LOT of good stuff there. Including a (now expired) patent of mine.
If I can find the paper copy I’ll try and scan it and upload it somewhere, but I’m not too hopeful. It was a long time ago.
The gist of it is that to plate metal with a smooth layer you need to reverse the current now and then to knock off the points that form. DC current plating leads to a rough surface with small peaks. This is just what is forming dendrites in Nicad battery charging. Same technique works there.
Should probably work on Li-ion.
Note the implication here: working hard to make your charging current be very constant is probably a bad thing.
But I stopped working actively in the field 15 years ago. Maybe there is something better. But I haven’t seen anything.
A few links:
http://www.lightartvision.com/downloads/NASA-II.PDF
https://www.dnd.hu/letoltes/AdvanceTec_whitepaper.pdf
and a competing company, claiming it does work. But ignoring the dendrites:
http://www.batterypoweronline.com/images/PDFs_articles_whitepaper_appros/powerdesigners.pdf
What you want to look for seems to be called “burp charging” rather than reverse current charging. All these burp chargers seem to use similar pulses.
The original article I read was a pretty simple circuit – transformer secondary with one diode in the positive charging direction (with a current limit resistor) and a second diode in the opposite direction (with a larger resistor to make the reverse pulse be smaller). No smarts, stop when you see the battery charged or bad things could happen. This was the 60’s.
These papers are all about nickel based chemistries.
At least for li-ion – what this article doesn’t mention (partly because the goal was to evaluate abuse at 100% DoD) is that charging it to a termination voltage lower than the cell’s peak charge threshold generally reduces these effects by a significant amount.
For example, charging a chemistry rated at 4.2v/cell only up to 4.1v/cell leads to *significant* improvements in cell lifetime (at the cost of capacity per charge/discharge cycle obviously).
Which is why Teslas default to only charging to 80%, and many manufacturers of products where longevity is more valuable than per-cycle capacity will derate their batteries significantly.
Charging to 4.1v/cell instead of 4.2 is, for example, the difference between a DJI Phantom battery swelling within 4 months and a Yuneec Typhoon battery pack that’s still in great condition after 3+ years.
The LiIon battery has no free metallic Li and plating out Li is a major degradation process. So it has to be avoided anyway, be it rough or smooth. Ni and Li based chemistries are quite different.
BMS has advanced since then by quite a bit, so it helps keep the cells outside of conditions where they’re discharged enough for the dentrite to not get zapped away.
Lithium cells are too delicate to be used without a BMS, whereas on nickel chem it was quite rarely used (and the cells are far more prone to a “fatal” dendrite internal short circuit), so there’s no demand for innovative methods of caring for lithium cells…at least not yet…
As more and more very expensive lithium batteries roam around in cars, there is and will be more pressure to squeeze every bit of life in them.
Lithium cells can’t be reversed like NiCd can. If the cell voltage drops below about 2.5 Volts, some chemical reaction takes place which destroys the cell permanently.
I always thought reverse current charging (discharge in not sufficiently balanced packs) is the main reason for degradation or explosion of LiIon cells. Thats the reason a BMS is checking all cell voltages very precise.
Using Li-Po is WRONG:
LiPo means Lithium Polymer, and refers to a technique to make separators inside Li-ion cells that was proposed but was never achieved in production for Li-ion cells.
Unfortunately, that term was misunderstood by the non-technical users to mean ” 3.7 V Li-ion pouch cell”, and that error is here to stay.
Please say “pouch cell” when you’re referring to the format (shape) and please say “3.7 V” when you’re referring to the voltage. But try not to say “LiPo”.
You can’t say “LiPo vs Li-ion” because they are all Li-ion cells, regardless of voltage, format or chemistry.
Thanks. I’ve updated the article accordingly.
I always thought, the pouch cells are LiPo cells – with a polymeric electrolyte so they do not need the compression by the metallic case.
Lipo isn’t Lithium-polymer. It is Li-ion in a poly pouch,
Actual Lithium-polymer isn’t really a thing anymore.
Really?
https://www.motionrc.com/collections/lipo-batteries
Use them all the time to power my RC airplanes and jets :)
Those RC-batteries are also just Li-ion in a poly-pouch. Same misunderstanding as other people talk about
LiFePO4.
That is all.
A very good, safe and stable lithium chemistry but with less cell voltage and energy density than most other LiIon chemistries.
The reason for the 2.5V limit is copper stripping, Cu plates where it is not supposed to.
Actually I’ve run cells down to 1.5V without permanent damage but the capacity can be affected if left for more than 24 hours in this state. Seems that discharging under load is what ruins them, damage actually occurs when the cell experiences fast charging because the controller does not compensate for the lower than normal voltage properly eg E cig 18650 causing affected areas of the plates to heat up and he ethylene carbonate to decompose: see pouch cells for this effect, bloating = BAD.
If you charge a 2.2Ah cell at 50mA max from 1.5 – 2.5V then it recovers eventually but people don’t want to wait that long.
Also useful: laptop batteries degrade when unused because one cell takes all the load from the controller board and ends up becoming damaged, some better controllers do now compensate for this by drawing power from more than one cell.
Incidentally the team who invented the Li-ion got the Chemistry Nobel this year!!
I was stripping lithium cells from camera batteries for my RC plane before there were commercial lipo cells. Wasn’t using a bms or balancing them either. They did pretty good for my old slow stick with brushless. Even the first commercial lipo I got didn’t have a balance plug. I keep my lipo batts in a cooler when transporting them, people are like you don’t need to keep them cool. Then they wonder why theirs puff and mine are still good 3 years no problem. Better to start with batteries at 70* than 90* since as soon as you spin up your quad they are just getting hotter! Also letting them cool before charging will extend their life as well as charging at 1/2 C If you need to fast charge better to buy more batteries so you can charge slower versus fast charging to use again!