The discovery of nuclear fission in the 1930s brought with it first the threat of nuclear annihilation by nuclear weapons in the 1940s, followed by the promise of clean, plentiful power in the 1950s courtesy of nuclear power plants. These would replace other types of thermal plants with one that would produce no exhaust gases, no fly ash and require only occasional refueling using uranium and other fissile fuels that can be found practically everywhere.
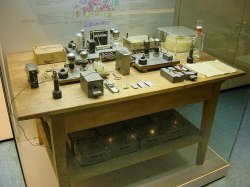
As nuclear reactors popped up ever faster during the 1950s and 1960s, the worry about running out of uranium fuel became ever more present, which led to increased R&D in so-called fast reactors, which in the fast-breeder reactor (FBR) configuration can use uranium fuel significantly more efficiently by using fast neutrons to change (‘breed’) 238U into 239Pu, which can then be mixed with uranium fuel to create (MOX) fuel for slow-neutron reactors, allowing not 1% but up to 60% of the energy in uranium to be used in a once-through cycle.
The boom in uranium supplies discovered during the 1970s mostly put a stop to these R&D efforts, with some nations like France still going through its Rapsodie, Phénix and SuperPhénix designs until recently finally canceling the Generation IV ASTRID demonstrator design after years of trying to get the project off the ground.
This is not the end of fast reactors, however. In this article we’ll look at how these marvels of engineering work and the various fast reactor types in use and under development by nations like Russia, China and India.
The ‘fast’ part of fast reactors
As alluded to in the introduction, the speed of the neutrons in their fission process is what makes a “fast” reactor fast. Whereas light-water reactors (LWR: including PWR, BWR and SCWR) employ regular water as a neutron moderator, fast reactors do not. The neutrons that are emitted by 235U and other isotopes when they are subjected to a nuclear chain reaction normally travel at a significant speed. Interestingly enough, the speed at which a neutron travels determines the likelihood of it interacting with a specific nucleus.
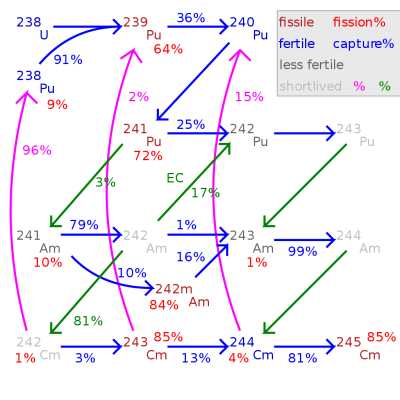
This neutron cross-section property is used to categorize nuclides. When a nucleus absorbs a neutron and either keeps it or decays, it is said to have a capture cross section. Nuclides that fission (shatter) have a fission cross section. Other nuclides will simply scatter the neutron and are said to have a scatter cross section. Nuclides with large absorption cross sections are called neutron poisons, as they will simply absorb neutrons without decaying, essentially starving the nuclear reaction of neutrons.
A nuclide like that of 238U is interesting in that has a non-zero rating in each of those three cross-section categories, which at least partially explains why it makes for such a poor fuel for a LWR. This is quite unlike 235U, which has a solid fission cross section, but only at neutron speeds which are significantly lower than those of freshly emitted neutrons during the nuclear chain reaction. This means that the neutrons in a LWR have to be slowed down (reduced to ‘thermal’ speeds) for a fission process to be sustained.
Here the water finds itself amidst the fuel rods, with neutrons flying everywhere as the fission process has been kick-started by the startup neutron source. These fast neutrons readily collide with the hydrogen atoms in a water molecule, which causes the former to lose kinetic energy and as a result slow down. This allows them to then careen straight into another (or the same) fuel rod and successfully fission another 235U nuclide.
This property of water as moderator also acts as a safety feature. If the temperature in the core increases, the water will end up boiling, which causes it to turn into a gas, meaning fewer water molecules per volume and thus less moderating of neutrons, effectively reducing the rate of the nuclear chain reaction. This negative void coefficient is a common feature of all commercial reactors in use today, with noticeable exceptions being the infamous RBMK design and the heavy water-based Canadian CANDU reactors.
Breeding plutonium for fun and profit
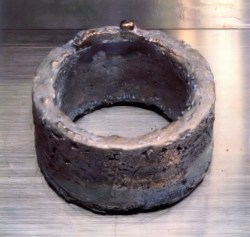
As mentioned earlier, 238U is a bit of an odd one when it comes to its neutron cross-section. Its triple-dipping means that it both absorbs and scatters neutrons in addition to the occasional fission event, with the former being significantly more prevalent. Upon capturing a neutron by a 238U nuclide, it transforms (transmutates) into 239Pu (and some 239Pu into 240Pu). This process also happens in an LWR reactor, but is done on purpose in a fast breeder reactor (FBR) to create plutonium.
A fast reactor omits the neutron moderator completely, as it requires the fast neutrons in order to convert as much of the 238U to 239Pu. In the FBR, an enriched 235U core is covered with a mantel of mostly 238U, which then slowly transmutates into mostly 239Pu and 240Pu, for use in MOX fuel. This means that the FBR is a relatively simple design, using either a cooling loop or pool design. Coolants used are generally a liquid metal or sodium-based coolant, as these are weak neutron moderators, while still possessing excellent heat transfer properties.
France’s fast reactors have been used to both generate electricity just like any other thermal plant, while also providing the plutonium needed for creating MOX fuel that can be used in its LWRs. A major reason for this process was energy independence, as France does not have significant uranium resources, this would have allowed it to obtain up to sixty times more energy out of the uranium it imports, allowing every kilogram of uranium to last sixty times as long.
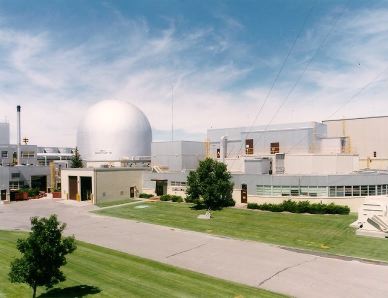
Other recent efforts involving fast reactors include the Integral Fast Reactor in the USA and Japan’s Monju (succeeded by the FNR Jouyou sodium-cooled fast reactor). A nice side-effect of breeding uranium fuel is that it significantly reduces the volume of the spent fuel at the end of a once-through fuel cycle, as much of the original 238U will have been burned as 239Pu fuel in the LWR. The spent fuel from LWRs can then be passed through an FBR again, to burn up ‘waste’ isotopes which LWRs cannot use, as well as to create more fuel for LWRs.
Unfortunately, fast reactors have the disadvantages of being more expensive than LWRs and the challenges of sodium-based cooling (mainly avoiding contact with water) have meant that since the 1970s crash in uranium prices, it’s generally more economically viable to create new fuel out of uranium ore and store or dump the spent fuel after a once-through run in an LWR.
Despite an LWR doing some breeding of its own, converting some of the 238U to plutonium, an LWR’s spent fuel still contains about 96% of the original uranium along with 3% of ‘waste’ isotopes and about 1% of plutonium isotopes.
Burn, baby, burn
While most fast reactors are used to breed fuel for LWRs, another type aims to use all of the fuel locally. This type of fast reactor is called a Fast-Neutron Reactor (FNR) and is essentially a different core configuration of the FBR design, with no fundamental differences. Any fast reactor can in theory be used to breed fuel and burn it.
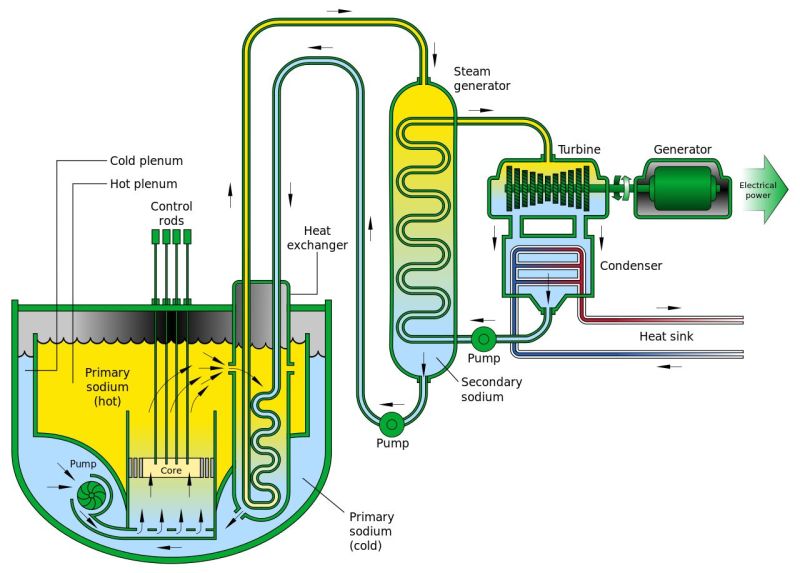
Changing an FBR design to FNR involves removing the 238U blanket and installing stainless steel (or equivalent) neutron reflectors. In the resulting reactor, the produced neutrons are kept inside the core, keeping them available for new interactions with nuclides and continuing the fission process.
As a result, an FNR can effectively fission and transmutate the nuclides in the fuel until no significant amounts of actinides (which includes uranium and plutonium) remain. This can be combined with pyroprocessing, which can reprocess today’s spent fuel from LWRs for burn-up in FNRs, effectively closing the nuclear fuel cycle.
French Resistance
Not only cold economics have played a role in stifling fast reactor development in the West. Fast reactors have caught the attention of terrorists and politicians alike. The former is illustrated by the 1982 rocket attack by Chaïm Nissim on the Superphénix FBR with five RPG-7 shoulder-fired rocket-propelled grenades, as he believed that an FBR “can explode with their fast neutrons”. This particular FBR was a joint project between France, Italy and Germany, with originally the goal to build FBRs based on the Superphénix design in both France and Germany.
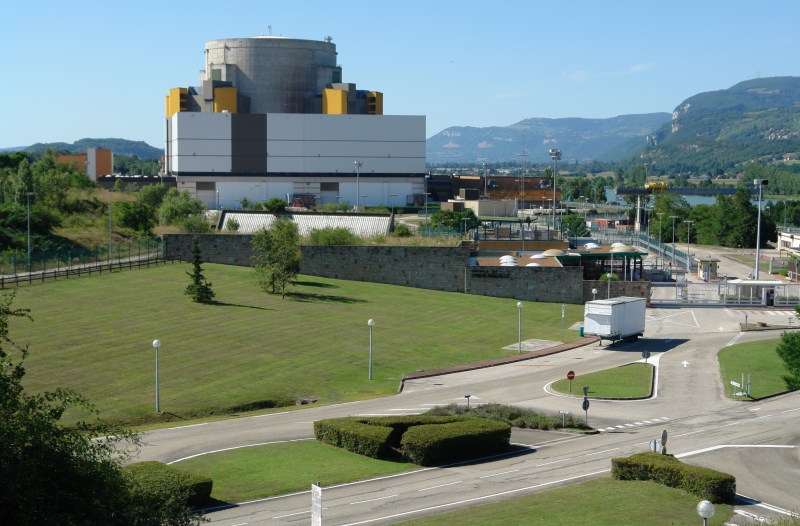
From the beginning the Superphénix faced strong political resistance by anti-nuclear groups, with the closure of this prototype reactor in 1998, at a time when anti-nuclear Green ministers were in charge of the French government. The only reason given was that the project wasn’t viable due to its ‘excessive costs’, being 9.1 billion Euro since 1976, or about 430 million Euro a year. This despite the reactor’s issues with the sodium loop having been resolved in 1996 and the reactor having made money by producing electricity during most of its operational lifespan.
Current development
The situation in the US, France and other Western countries contrasts sharply with that in the Soviet Union, China and India. Starting in 1973, the BN-350 FNR on the shores of the Caspian Sea in what is now Kazakhstan provided 135 MW of electricity and desalinated water to the nearby city of Aktau. It only shut down in 1994 because the operator had run out of funds to purchase more fuel. In 1999 the reactor was fully retired, after 26 years of service.
The BN-series of FNRs continued with the BN-600, which was constructed at Beloyarsk Nuclear Power Station in Russia. This uses a sodium pool-based design and has been in operation since 1980, providing 600 MW of power to the local grid. Despite suffering a few dozen minor issues mostly related to leaks in the sodium tubing, its operational history has been largely trouble-free despite being the second prototype in the BN-series.
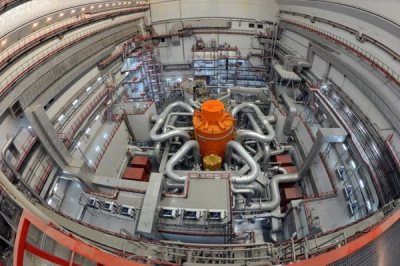
The BN-800 reactor, built at the same Beloyarsk site, is the final prototype in the BN-series, providing 85% reduction in operating costs over the LWR VVER-1200 reactor, with the BN-1200 intended to be the first mass-produced fast reactor. Construction of the first BN-1200 reactors is currently pending. China’s experimental CEFR FNR and CFR-600 pilot FNR are based on Russian BN-reactor technology. Russia is also working on a lead-cooled fast reactor, called BREST.
India has found itself with abundant thorium (232Th) resources, which has led to it focusing on an ambitious thorium-based development program alongside uranium reactors. The thorium program consists out of three parts. First, they produce plutonium from uranium using LWRs. Then a FNR creates 233U from 232Th while burning the plutonium. Finally, advanced heavy water reactors would use the resulting thorium as fuel, and the 233U and plutonium as driver fuels.
Other Generation IV FNR designs are also under development, such as the helium gas-cooled fast reactor (GFR).
Closing the fuel cycle
As mentioned earlier, FNRs are capable of using all of today’s spent fuel (often referred to as ‘nuclear waste’) as fuel. Combined with pyropocessing, this would allow for nuclear fission reactors to operate with practically zero waste, using up all uranium fuel, minor actinides and so on. This has been a major goal of Russia’s nuclear program, and is one of China’s, Japan’s and South Korea’s nuclear programs as well.
Along with efforts in the US (mostly Argonne National Laboratory and its IFR pyroprocessing), South Korea’s KAERI is actively working on closing South Korea’s fuel cycle. The goal is to separate the spent fuel from everything that is still viable as fuel, meaning everything that is still radioactive. Unfortunately cooperation between Russia and nations other than China, as well as between South Korea and Japan or China has been very limited on this type of research on mostly political grounds.
Despite this, it seems that efforts are well underway to make Generation IV FNRs the reactor of choice for new plants, not only allowing for spent fuel to be used up fully and closing the fuel cycle, but also increasing the energy we can obtain from uranium (and conceivably thorium) by many times, increasing even the pessimistic estimate of about 100 years of uranium fuel to a comfortable few-thousand years, while not leaving the world a legacy of spent uranium fuel.
That would explain why China bought the triSodium process from Dupont. Only problem the only place in the word that the process works great is in NY usa.
What would make only one geographic region suitable for a particular type of chemical processing?
Attempting to google “TriSodium” leads to absolutely nothing of relevance for nuclear fuels, only lots of information about the rather common cleaner Trisodium Phosphate.
As far as I can tell, the ‘unique’ situation is that electricity is very cheap, and transport links are very good. I suppose this might make it possible to use a process that is not economically viable anywhere else.
Sodium production in Niagara finished in 2015.
I’m not sure what’s so special about liquid sodium. It melts at 97C so if you had little regard for personal safety, you could melt some in a water bath (just don’t try it anywhere near me).
Or they simply perform the processing on-site, which satisfies those requirements.
What people dont get when I said TriSodium Process, that is the LIQUID Metal (Used in the first Breeder Reactor here in the US, just north of the Craters of the Moon, Idaho). That process is a result of making chlorine gas from table Salt (or Sea Salt), the side product being Sodium. And because where the process happens (Niagara Falls NY) it naturally makes TriSodium (it has to do with atmospheric conditions that happens in Niagara Falls, which is also funny because the process cant be duplicated anywhere else from what I was told in the world. So Selling it to the process to the Chinese is going to be well… ). But still that will make it easier for them to make weapons grade Pu, if they work out how to keep their heat exchanger from corroding, like what happened with the EBR1. I still think it was a mistake to sell our process to them.
RE Chernobyl- what is left out of a lot of talks about design of that plant… It was designed to make 2+ pounds of Pu per day. Something that was left out of the knowledge of the people who were running it during the accident, do to that knowledge was classified as a military secrets (along with that, that the reactor was a breeder reactor- not some symple LWR). That is like driving a tank, with a fully loaded muzzle down a city street, and not telling the driver which button could set off the cannon.
It is amazing that we did not blow ourselves up during WW2 in the Manhattan project, because we were using graphite reactors to make our own Pu, but we were not making steam (Which Chernobyl was making steam for making electricity- their accident was really a steam explosion {and maybe chemical explosion of hydrogen and O2 because of the reactor being over 7000 deg F out of specs- Same worry about 3 mile island about the hydrogen and O2 bubble during that accident.} do to overheating of the Chernobyl reactor. The grafite catching of fire was secondary to the explosion, along with expelson of radioactive by products. The pipes were too hot to cool it and failed, to name one of many reason. Even though they had Pu in the reactor it was NOT a nuclear bomb explosion. ), we used the water to just cool our WW2 reactors (It blows my mind that we could take 35 Deg F water from the columbia river and bring it to boiling under 2 seconds through 50 feet of pipe in our reactors – yes, tons of water moving through that reactor). And unlike the British, who try using air to cool their Graphite reactor during WW2 and which caught fire because of mishandeling of their reactor process, and spewing radioactive elements {iodine being one of them) all over the countryside {despite having carbon filters scrubbing in the stack for the radioactive particles}, we again used water to just cool our reactor(s).
You must be thinking of the windscale accident when mentioning the brits. That was in 1957.
It is known for quite some time, that Chernobyl was designed without an outer containment to simplify the production and removal of the produced plutonium. The main reason for the explosion was an experiment which was done too fast under political pressure by an inexperienced crew.
Almost all sodium is produced from NaCl, normally the Na or NaOH is considered the main product and the chlorine a byproduct. Some people go even that far to say that PVC was invented to get rid of vast amounts of surplus chlorine.
I doubt very much that sodium production is limited to Niagara falls. I am sure the Russians did not buy the Sodium for their reactors from the US. Surely with the properties of Sodium you want to exclude the atmosphere as much as possible from the production process. The reason Sodium is used in this type of reactor is, as the article says “Coolants used are generally a liquid metal or sodium-based coolant, as these are weak neutron moderators, while still possessing excellent heat transfer properties”. The low melting point of sodium is of course also an advantage. Another reason it is popular with fast reactors is that it is very compatible with steels commonly used in the industry, as long as it is free of Oxygen. I could not find any records of corroding heat exchangers on the EBR 1, which used NaK as a coolant.
From what I read about about fast molten salt reactors, even oxygen free sodium chloride is not very corrosive.
Economic sodium production is easier when you’re close to a hydroelectric source. You can make sodium at home. I’ve done it. Mix table salt and baking soda, melt in a crucible over a bunsen burner (the mix melts at a lower temp than either alone) and stick in two electrodes with a couple of volts between them. But if you want to make a lot, you need a lot of cheap electricity. It’s also nice if you have lots of cheap sodium ores nearby. Upstate New York got a head start on many manufacturing processes because they had a well-developed infrastructure for moving bulk materials by canal, and once that was established, later development in railroads and hydroelectric was concentrated there, maintaining their manufacturing strength.
“cheap sodium ores” that could be any place at the sea :-) At least where it is warm enough to produce sea salt by evaporation.
While I am not opposed to nuclear power as such, the phrase “generates huge amounts of plutonium” is not the sort of thing which calms the mind of any rational person.
Also, as far as I can tell, all of these reactor designs are still “fail bad” designs in which problems must be actively addressed by knowledgeable people making informed decisions under stress. This means that sooner or later there will be a significant failure and in nucspeak “significant” often means depopulating more than a few square miles. Thorium molten salt and most renewable generation methods on the other hand “fail safe”. If the crew walks away the thing eventually shuts down in a way that doesn’t endanger anyone outside the immediate area. I like that design philosophy a lot.
The functioning of an FNR is dependent on the presence of fast neutrons. Remove the neutron reflectors and the reaction effectively stops. LWRs with a negative void coefficient also fail safe, as the article points out. The CANDU and today’s RBMK reactors do not have Chernobyl’s issue with positive void coefficient by avoiding the ‘SCRAM makes stuff go boom’ issue, which those hapless folk found out with the #4 reactor in 1986.
As for Fukushima, that was the hydrogen explosion from the hydrogen produced by zirkonium cladding being exposed to the air in the spent fuel pools. If TEPCO had installed hydrogen venting mechanisms (highly advisable since TMI’s incident, but completely ignored by TEPCO, among every other safety feature), the Fukushima Daiichi buildings would have remained intact, with no material spread around. The reactors likely would have melted down (safely), because of the other string of f*ckups (incompatible connectors, submerged generators in basement, tiny flood wall, etc.), but it’d have been significantly less messy.
So no, these new designs are not ‘fail bad’ designs. Even today’s Gen II LWRs aren’t ‘fail bad’, let alone Gen III+ plants going into operation today, courtesy of passive safeties and more. Thorium MSRs would also not be magically different from FNRs using sodium (or liquid metal).
But that’s a whole other article, most of it unrelated to fast reactors.
Fukushima might not have even gone into meltdown at a all if the moved the generators out of the basement and didn’t shave down the island to make the cooling plumbing easier.
TEPCO was criminally negligent in the decisions they made.
Indeed. The Japanese Diet’s report on the accident pretty much completely destroys TEPCO on every single point. It’s no wonder that TEPCO no longer exists as a company.
GE had warned them about everything that went wrong. They told them to raise the tsunami wall and to elevate the generators and their fuel tanks. How incredibly stupid to keep the generators where they’d easily be flooded. If they had not been, there would have been no problems. For the idiots who made those choices should have been sent to prison.
I had seen some reports implying that these reactors had at some point been retrofitted with isolation condensers (part of the passive safety features in newer reactor designs), but they hadn’t been installed correctly?
Not sure about that one… Either way, lesson learned is “build modern plants, don’t service life extend some of the oldest operating reactors on the planet”.
“Remove the neutron reflectors and the reaction effectively stops.”
Not really. Doing a full stop on a reactor still leaves about 10-15% of residual thermal power with the core that takes hours and days to die down, which is why large reactor cores don’t just stop when you pull the emergency lever, and why you have to keep providing the cooling. If all goes well and all the safety systems and mechanisms are working correctly, it’s theoretically a walk-away situation, but so much can still go wrong especially with reactors that are otherwise damaged, with the potential for steam explosions or generating hydrogen and oxygen with reactions between superheated water and materials in the reactor core.
This is a problem for all reactors which are too large to shed heat passively. Not so much for small modular reactors.
With molten metal cooled reactors you can design it so you can make where it get away with passive radiators to deal with the latent heat since the boiling temp of the coolant is much higher.
They did a shut down like Fukushima on a metal cooled reactor in the 1970s and it stayed safe.
Much of the dame to an improperly shut down reactor happens when the pressure vessel fails and the coolant gets boiled away leaving nothing to remove the heat.
That too depends on the size of the reactor. There are critical dimensions to the device where the heat output of the core will surpass its ability to expel heat passively, especially in a loss-of-coolant (leak) event.
About Fukushima, I knew about the below-ocean-level generators (BIG facepalm) but never heard/read anything about mismatched connectors though… Thank you for an highly informative comment all-around.
Reactors like the Fukushima Daiichi ones have an external power feed-in connector so that electrical power can be supplied in emergencies when its backup power fails (as had happened in the case of Fukushima Daiichi). Unfortunately the emergency vehicles that were on-site did not have the right connector available that would have fit, and thus they could not provide emergency power.
It was one of the many points where Fukushima Daiichi’s reactors melting down could have been easily prevented.
Then best design it so, that in an emergency jumper cables with clamps could be used. :-) Not very nice, not perfectly safe and to be replaced with proper connectors as soon as possible, but perhaps it helps.
Or even better: keep some adapter cables on site.
The problem is not the particular path to failure, but rather the unstable nature of the process. A self-amplifying process is started, and then controlled by another process, but you need a backup process in case the second process is interrupted and some way of monitoring all three of these processes, plus backups for the monitoring process and the resulting time lags and multiple feedback loops and systems that need to work but can’t be tested in a real-life situation and given enough operating hours something goes wrong. Read “How complex Systems Fail” by Richard Cook.
What’s extra crazy is why was a nuclear power plant built to be absolutely dependent on outside power, or generators when outside power was interrupted? It’s a *nuclear power plant*, when up and running it should be able to tap the power it produces to operate all its own pumps, lights etc. Off-site power should only be required to do a startup when all the on-site reactors are ‘shut down’ and the turbines aren’t spinning.
Yes. Should. That’s what they were testing at Cherobyl.
When the turbogenerators are cut off from the main grid, they lose the load and the turbines would overspeed in a matter of seconds because there’s a gigawatt of power going nowhere except to spool up the generator faster and faster. When they do an emergency shutdown, they have to bypass the turbine as well or else all hell breaks loose.
With the generator no longer receiving steam, it can only provide power for some few minutes before it slows down too much and the voltage and frequency go all over the place.
Why bypass it completely? run most of the steam in a condensor but keep control the turbines at the proper speed. If it’s a minimum load problem then you could even install an electrical dummy load.
The issue is what is called “decay heat”. Even if you SCRAM a reactor, it still generates somewhere around 10-15% of its operating heat initially. (This decays fairly rapidly, but is still not insignificant after 72 hours – but getting past the first 72 hours is the real danger zone).
On older designs, getting rid of this decay heat required external power.
On newer designs, the backup generators are not safety critical and decay heat can be dissipated passively. (IIRC, in some Gen3 designs, human intervention is eventually required after 72 hours by topping off a pool if it boils off – but this pool is isolated using what are effectively heatpipes so can be topped off by any old fire truck.)
I’m not sure I agree that there would have been no release if not for the hydrogen explosions. After all, there was still a full meltdown. Are you saying that the containment would have prevented release entirely?
In any event, scientific consensus is that Fukushima caused no deaths and will have no measurable public health impacts. As to the earlier reference about large areas “needing” to be evacuated for long time periods (with such “fail bad” reactors), analyses show that no areas around Fukushima were as unhealthy a place to live as most of the world’s large cities. Thus, the long-term relocations in Japan were unjustified.
http://www.bristolpost.co.uk/news/bristol-news/people-near-nuclear-power-station-853901
https://www.sciencedaily.com/releases/2017/11/171120085453.htm
I agree with you that LWRs are not necessarily “fail bad”. This is especially true of small modular LWR reactors like NuScale. Simply due to their small size (low radionuclide inventory and larger surface to volume ratio), they are incapable of creating radiation levels above the natural range anywhere outside the plant site boundary. That is, even after a meltdown, or even after all components fail. MSRs may be great, but they are not necessary in this regard.
any rational person would look up how actual plutonium 239 production works and realize that apart from specialised breeding reactors (or the RBMK…), FAST power generation reactors are not suitable for this purpose, especially if you’d want to do it covertly, because there are international treaties with inspections regarding anything that can produce plutonium.
Exposing U238 to neutrons will breed not only Pu239 (which you’d want for a weapon), but also Pu240, which has a relatively short half life (=very radioactive), so apart the highly active material handling problems which are “fun” in their own ways, you’d have to perform isotope separation (=difficult, expensive and hard to hide).
Even small amounts of Pu240 contamination make the the remaining Pu239 unsuitable for weapons, as it becomes difficult to prevent triggering fission before the right moment, which would result in a nuclear fizzle.
Plutonium is a severely hazardous material even if you don’t make a bomb out of it. It is flammable, poisonous, carcinogenic and all in invisibly tiny amounts. Perhaps we can put enough controls on the fuel cycle that there will be no accidental or intentional releases of Plutonium over the next several thousand years while hundreds of tons of it are created, transported and subjected to various chemical and mechanical manufacturing processes, but I doubt it. I’d rather just not create any more if we don’t have to.
” and all in invisibly tiny amounts”
Except not. Plutonium is technically hazardous in small amounts, but only if you actually get it into your system. It’s actually surprisingly difficult to absorb. Because it’s so reactive, especially when it’s made into fine dust, it almost always becomes an oxide before you are exposed to it, which makes it difficult to dissolve. It serves no biological function, nor does it closely resemble anything that does, so it is not actively taken in by the body.
If you eat a gobful of plutonium oxide, only 0.04% of it ends up being absorbed into the body – mainly by diffusing through the gut lining and cell membranes – the rest simply passes through without lasting harm. Of course that amount of pure plutonium oxide would kill anyone, but the point is clear – if you get microgram amounts of it through contaminated food for example, nanograms will be absorbed.
Plutonium is more dangerous when inhaled, because the dust gets stuck in your lungs – but the lungs are also removing particle matter all the time, so it doesn’t stay with you unless your lungs are already irreparably damaged by something like smoking cigarettes for half a lifetime.
There’s even 25 workers from Los Alamos who inhaled plutonium dust while working there, and according to the old “hot particle” theory, all of them should have died of lung cancer. None of them were. No human is known to have died as a result of plutonium inhalation despite many people being exposed to it. The dangers of plutonium are massively exaggerated.
It’s estimated that 200 micrograms of Plutonium is enough to cause you cancer.
If so, then you’d actually have to eat half a kilogram of Plutonium – much more if you count it as plutonium oxides – in order to absorb enough to eventually cause you cancer.
Sorry, that’s half a gram, not half a kilogram. In any case, a perfectly visible amount of Pu.
PuO2 has a density of 11.5 g cm−3 and assuming most of the mass is Plutonium, the required particle size is 43 cubic millimeters, or roughly the size of an airgun pellet.
from the Plutonium MSDS:
Plutonium is one of the most dangerous substances known. The metal and it’s salts are all highly toxic. Its ionizing radiation can cause cancer. The metal can incorporate with bone marrow forming insoluble plutonium (IV) phosphate. The metal only leaves the body very slowly. All operations must be carried out by remote control devices with proper shields. In production, processing, handling, and storage of large quantities of plutonium or its compounds one must bear in mind its critical mass, which can vary with the shape and the specific solid form or the quantities of plutonium contained in solutions.
>”The metal and it’s salts are all highly toxic.”
Yes. The metal and its salts. Plutonium however does not exists as plain metal in the environment because it’s so reactive it oxidizes rapidly. The fuel in a nuclear reactor is also oxides – it’s made into ceramic pellets. PuO2 has extremely low solubility in water, or even stomach acid.
In order to have plutonium salts, you must first dissolve it into a strong acid or base, like hexafluorosilicic acid.
Nice writeup. We need more nuclear power stations. The Gen IV stuff answers waste concerns. They emit no CO2! Why aren’t the people who run around wanting green energy looking at this and welcoming the idea? For the cost of a windfarm we can have much more reliable power.
Because they aren’t about the realz, they are about the feelz. “I DON’T CARE ABOUT YOUR DATA AND SOLUTIONS, I JUST WANT TO BE ANGRY!!!”
Actually the folks who want green energy are not interested in nuclear power for two reasons:
(1) They want green power soon, like lots of it in the next decade (or two at most). Solar cells can be massed produced in many factories and an installation takes months from idea to production. Same with wind power. Nuclear plants take at least a decade to build, even without legal battles over permitting and location and there are only a couple companies capable of actually building such projects. Nuclear is a 22nd century solution to a 20th century problem we are trying to fix in the 21st.
(2) Gen IV power is science fiction, Gen III is prototype, Gen II never made it to mass production. Every nuclear plant that exists has been designed fresh from the ground up. The BN-1200 is supposed to be mass produced but is still on the drawing boards, all the current fast/breeder reactors are test units which only incidentally produce power. All the other stuff, kilopower, pebblebed, powerwave, etc all exist as simulations or straight up imaginations, not a prototype among them all at least 25 years away from producing a single watt.
Nuclear power has been so slow in development because of people with attitudes like yours.
Actually, all the fastest transitions away from fossil fuels in the power sector have been nuclear (and hydro) based. We achieved that decades ago, in France and Sweden, etc.. Solar and wind have yet to come close to that rate of decarbonization. Germany *hopes* to do in 50 years (2000 – 2050) with renewables what France *did* do in ~15 years with nuclear. And Germany is already running into trouble. Solar and wind have limitations (intermittentcy, land use) that nuclear simply doesn’t have. The growth rate of solar and wind has never equaled the peak nuclear growth rate that occurred in the ’70s and ’80s.
It’s false to say that nuclear is not capable of decarbonizing the grid as fast as solar and wind can. It can do it faster (as previous experience shows). All it requires is political will. On the other hand, there are serious questions as to whether solar and wind will be able to provide most, let alone all, of our power, since that would rely on massive-scale electricity storage tech that is still nowhere on the horizon. Here’s an MIT analysis which states that, even assuming battery costs fall by a factor of several, a grid which uses renewables for more than 50% of overall power would result in power costs over $1/kW-hr!!
https://www.technologyreview.com/s/611683/the-25-trillion-reason-we-cant-rely-on-batteries-to-clean-up-the-grid/
You are aware of the fact that virtually all nuclear plants producing power today are Gen II plants, right? Like, they started building them in the late 60s. New plants being built right now are mostly Gen III+ LWRs, with some Gen IV designs up and running (mostly MSRs). Russia’s BN-series has been running uneventfully.
So, why say things which have no base in truth? You have been spamming this conversation with lots of claims which only have cursory contact with reality. Why do this?
I know Gen II plants have been around since the 60’s, I also know that they are each designed for a specific site and set of regulations, they are artisanal products, not mass produced items. Newer LWR’s could be called Gen II+, they function exactly like the old versions, just with new safety attachments. FNR’s like the BN series are Gen III plants and all the existing ones are considered prototypes. As far as I can find there are no currently operating MSR’s (since the thorium project(also a prototype reactor) was shut down). All the “super safe, ultra clean” Gen IV reactors(pebble bed, traveling wave, etc) are concepts and simulations. If you can point to an operating model I will be glad to accept it.
BN-series fast reactors, the currently active fast reactor in Japan and the two fast reactors in Chinese (CEFR, CFR) are all Gen IV MSR designs.Just because you think that you’re right doesn’t mean that you get to disagree with nuclear physicists.
It may also interest you that both France and Sweden did do mass-produce and build Gen II reactors, when they decarbonised their economies in the span of about a decade, many years ago. They picked a couple of standard designs and built lots of them.
The Russian BNR and the Chinese C*R series are not MSR’s they are FNR’s and are considered, even by their owners as prototypes, although the Russians are working on a production design for their series. Just because you think that you’re right doesn’t mean that you get to disagree with nuclear physicists.
I was not aware of the high level of standardization in the French system, I mainly study US stuff; but France has not decarbonized their grid, they still use about 15% hydrocarbons and possibly more if one includes power imports. Also, France is currently shutting down plants, looking to decrease nuclear to 50% of it’s electrical output over the coming decades and has had several accidents and safety related shutdowns. But yes, there have been instances of “mass produced” Gen II reactors.
There is no MSR – molten salt fueled of cooled reactor running, maybe a very small experimental one in China. The Russian BN reactors, are metal – Sodium – cooled.
>”even without legal battles over permitting and location and there are only a couple companies capable of actually building such projects”
That’s a false argument. The very reason there are only a couple companies like that is because building nuclear powerplants has been made so difficult that only a handful of big energy corporations can sustain this line of business.
Have you ever seen a nuclear power plant? The reason they take years to build is that they are HUGE and they involve huge structures made of heavy duty steel and the construction tolerances are incredibly fine. You can’t pull some random kid out of tech school and send him to weld up a few hundred feet of two inch thick stainless steel and demand no voids or burns, this stuff requires expert welders like the Met requires expert singers, and there are about as many of both. And they take as long to train. The size and complexity of these projects are the reason that they take so long and that there are few contractors who can handle them.
And while you may disagree with the people who don’t want to live near a nuclear plant you cannot wish them away, in the US they have the right to go to court and calling them NIMBY’s, while true, does not change anything. Similarly, you can complain about red tape and certifications, but do you really want them to build less safe reactors?
You’re still begging the question.
The reason why nuclear plants are huge is because it takes years and years to get a permit to build one, which means years and years of lost profits that have to be made up once the plant is in operation.
When you’re only ALLOWED to build one per decade at best, presuming you’re then actually allowed to start it, or run it for any number of years, you HAVE to make it big in order to make any money over it. There’s no economic sense in building smaller ones because you’ve just spent the last decade wasting money to keep your business up while you’re unable to build anything or sell anything, and you have to spend the next decade cutting through red tape and fighting off protestors and political saboteurs to get something built.
There’s a reason why the new EPR in Olkiluoto Finland has taken 20 years from planning to completion and still they’re not giving it the green light to start, while the EPR in Taishan China took 10 years and went online in 2018. Same reactor, same supplier, different regulatory environment.
>”you can complain about red tape and certifications, but do you really want them to build less safe reactors?”
You’re making a false dilemma. The red tape and certifications don’t make the reactors any safer than they are designed to be. If anything, the opposite is true because these artificial hurdles and general lack of demand create an economic situation that favors reactors that are too large to be passively safe.
The more expensive and difficult you make it to actually build the plants, the less competition you get, and the higher your chances of corruption and collusion. See TEPCO.
>”The reason they take years to build is that they are HUGE ”
The reason they are HUGE is because it takes so many years to plan and clear political hurdles that a smaller reactor wouldn’t make the money back in a reasonable time.
A naval type small nuclear reactor used in submarines and carriers costs about $100-200 million to build because they’re pretty much copies of a standard type and they don’t need so much active safety because they’re small. Also, because they’re used by the military so they don’t need extensive permits and insurances and financing costs and ten years of lobbying to build a new one.
They come in 50-150 MW sizes for output power, which puts the cost around 1-2 million per megawatt. Coincidentally, that’s about same as wind turbines, except of course the nuclear reactor can run almost 100% of the time while the wind turbine can’t.
NO, Nuclear plants are huge because they need to be to produce megawatts for grid base load, they cannot be used in a disbursed setting like solar because of security concerns regarding the fuel/wastes, they need to be huge because the investment in any centralized power plant is huge and making the plant bigger improves the cost/benefit ratio.
You say the problem is red tape yet in France and China where red tape is minimized it still takes a decade to build a plant and more to bring it fully on line. The French made nuclear power their Apollo program, planning to build 170 reactors in 15 years, they managed 57 and found out that many of those had fraudulent safety certifications.
Red tape does not improve the safety of a design, it ensures that the final product is as safe as the design says it should be.
Similarly the small reactors built for the military are small and cheap partly because the military can throw money and men at them to keep them that way. Military reactor crews stand 2-4 hour watches, meaning the staff is 2 to 4 times as large, and by the way is forced to sleep just yards from the reactor so they are handy for emergencies. Also military reactors are treated like fighter jets, anything that gets measurably out of whack gets replaced, no error tolerance or re-calibration. Nobody else can afford to run a reactor like that.
” Nuclear plants are huge because they need to be to produce megawatts for grid base load”
A Megawatt is small, one thousand megawatts is big. Nuclear plants used to be in the low hundreds of megawatts, but then they gradually crept up to 1000 MW and beyond because the economic limitations imposed on nuclear power favored larger and larger reactors, resulting in decades of lead time. This has nothing to do with providing megawatts of base load power. Many small reactors will do just the same as one large.
“yet in France and China where red tape is minimized”
Relatively speaking. It is not at its absolute minimum.Your argument is based on false premises.
“because the military can throw money and men at them to keep them that way”
That’s called automation and walk-away safety. These reactors are too small to melt down out of their containment, making them inherently passively safe – by design. They’re also designed to operate for 20-30 years without refueling to reduce the maintenance cost.
The military has 5,400 reactor-years of safe operation without a single accident, which is highly unlikely if it was down to some bored recruits pushing buttons.
“Red tape does not improve the safety of a design, it ensures that the final product is as safe as the design says it should be.”
Again, see TEPCO, or your own argument: “…and found out that many of those had fraudulent safety certifications.”
All the red tape does not in the end ensure that the reactors are safe. They either are by design, or they are approved by someone who is willing to look past the faults and hope that nothing goes wrong. Making every single reactor go through the same song and dance every time merely costs everyone a lot of money and time.
Do I want less safe reactors? Frankly, yes. This whole zero risk for nuclear only thing has to stop. Given that nuclear’s risks/impacts are orders of magnitude smaller than those of fossil generation, the focus should be maximizing nuclear *deployment* as opposed to minimizing nuclear-specific risks further and further. It has gotten out of hand. Having nuclear’s risks be 1000 times lower than fossil, but also having nuclear be so expensive that it is not used (and fossil generation is used instead) would yield far greater *overall* risks/impacts than having nuclear’s risks be 100 times lower than fossil, but it being competitive so that it actually replaced fossil generation.
Fukushima was a worst-case meltdown of 3 large reactors and was the only significant release of pollution (outside the old Soviet Union, which is a special, non-applicable case), in nuclear’s entire 50+year history. It caused no deaths and will never have any measurable public health impact, thus essentially showing that even severe meltdowns are incapable of causing significant loss of life. In stark contrast, over that same (50+ year) time period, fossil power generation has caused on the order of 10 million deaths, along with global warming. Even the most pessimistic credible theoretical estimates of Fukushima’s (too small to measure) total eventual death toll are one tenth of the number of deaths caused *every day* by worldwide fossil power generation.
As for reactors costing so much because they are so “large” and “complicated”, we built large reactors as less than 1/3 of today’s cost, back in the ’70s. Korea built them at low cost decades later. Thus, there are no such technical reasons why they have to be so expensive. The reason for the greatly increased costs is excessive and ever increasing regulations and QA requirements. These excessive requirements are not justified. In fact, as explained above, they have actually *increased* overall public health risk and environmental impact, by resulting in massive amounts of fossil fuel use in nuclear’s place. Analyses estimate that nuclear (used in place of fossil generation) has already saved ~2 million lives. It would have saved millions more if not for political opposition, and the excessive burdens that resulted.
Finally, you say that we can’t build small reactors, due to the need to “secure” the nuclear materials. Then why are so many companies and countries actively pursuing small modular reactors (SMRs) with power capacities of 50 MW or even less? You also described France as having lower levels of red tape (regulation). As if! Their nuclear regulator is even more out of control than NRC!
“they cannot be used in a disbursed setting like solar because of security concerns regarding the fuel/wastes”
Actually, they can. If you again take a note of the naval reactors, they have fuel cycles up to 30 years. They can be re-fueled, but that’s not strictly necessary because by the end of their life the entire reactor is lifted out and sent for de-fueling and de-commissioning. This means an entirely sealed unit that comes from the factory already welded shut. Rolls-Royce is currently developing small modular nuclear plants under UK funding, based on naval reactors, small enough to be carried by truck.
“anything that gets measurably out of whack gets replaced, no error tolerance or re-calibration. Nobody else can afford to run a reactor like that.”
Naval reactors are designed to run inside an ocean-going battleship which may get shot by missiles and torpedoes. The way the military runs them is to ensure they keep operating even when the ship is being thrown around in a storm and being fired at. There are couple cases of these ships being sunk with their reactors, and nothing happened because the reactors are by design walk-away safe. A land-based reactor of the same type has a vastly easier life and doesn’t require such babying – especially if you don’t build them on tectonic fault lines.
“The French made nuclear power their Apollo program, planning to build 170 reactors in 15 years, they managed 57”
That’s still vastly faster than one every 20 years like the EPR in Finland, or one every 10 years in China. It shows that if there is the will, and pre-approved designs and construction, you can build at an averaged rate of four conventional large reactors per year! A scale of 100x faster than what the current regulatory environments will permit.
The argument that nuclear power is too slow and expensive to build, because it must be built this and that way, is just begging the question. Real world examples to the contrary exist.
” And while you may disagree with the people who don’t want to live near a nuclear plant you cannot wish them away, ”
Most people don’t want to live close to windmills either. I used to live in Cape Town, and Cape Town was – is – rapidly expanding towards towards the only nuclear power plant in Africa. Amazingly some of the new arrivals in the area are protesting against the power station. I wish somebody would replace the power station with a coal fired one, that should get rid of a lot of poeple in the area.
I run around looking for green energy, and I welcome nuclear power! I hope that brightens your day :)
The only thing I don’t like about nuclear relative to e.g. solar is that it’s relatively difficult to put a nuclear reactor in my home, and if I did manage to, I imagine that would be a matter of some concern to my neighbours and municipal/national authorities. While everyone makes mistakes, I think it’s important that we make an honest effort to comply with non-proliferation treaties.
Solar is neat because I can have my own power plant. I really do look forward to cleaner and safer nuclear though.
I am not opposed to nuclear power as such, it is after all hard to run an aluminum smelter off windmills. But the idea that we should power the world off of fission reactors was bad in the 60’s and it’s bad now. Nuclear fission can be useful in situations where there is high density, continuous consumption of electrical power such as industrial parks and huge or isolated cities (NY and Hong Kong)but for the foreseeable future it needs to be a niche provider in situations where it’s virtues outweigh the very real dangers involved.
You mean the very real dangers of nuclear power being the safest form of energy production per TWh, even with the Chernobyl and Fukushima disasters being massive outliers in cultures that are non-representative of how most nations (Canada, US, France, South Korea) do safety?
Please quit it with the FUD.
The “safety” of nuclear power generally results from calculating in costs like lung disease from fly ash (which is legit) but not lung disease from Chernobyl, (which is also a thing). Lies, damn lies, etc
The simple fact is that no other energy source has to have it’s fuel/waste guarded to prevent people from turning it into WMD’s. No other power source, when shutdown, needs to have large parts of the plant entombed or disassembled by robots because it is to dangerous for people to be near. No other power source(except possibly hydro) has ever made city size areas uninhabitable for years/decades/who knows.
FUD is not a bad thing when confronted by the possibility of catastrophe. As for X disasters being massive outliers; I said elsewhere, read “How complex Systems Fail” by Richard Cook.
“The “safety” of nuclear power generally results from calculating in costs like lung disease from fly ash (which is legit) but not lung disease from Chernobyl, (which is also a thing). Lies, damn lies, etc”
No. Nuclear power compares favorably even in comparison to wind and hydroelectric power, which kill more people per TWh produced.
And the lung disease from Chernobyl is counted in. It’s just that the radioactive fallout outside of the immediate area was so diffuse and dilute that the incidence of cancer dissapears into the statistics. Using the LNT model in the comparison will also OVER-estimate the number of cases, so the Chernobyl accident is already treated as being worse than it actually was in the calculations.
>”No other power source(except possibly hydro) has ever made city size areas uninhabitable for years/decades/who knows.”
Ironically, mining operations for rare earth elements used in wind turbines, solar panels, and lithium batteries, electric cars, etc. have resulted in massive releases of radioactive pollution. See Mitsubishi and Bukit merah:
https://www.nytimes.com/2011/03/09/business/energy-environment/09rareside.html
Despite the cleaning operations, you wouldn’t want to live there.
>”FUD is not a bad thing when confronted by the possibility of catastrophe.”
Pascal’s Wager fallacy.
Also notice that FUD is inherently about lying and propaganda – about sowing distrust by making claims that are not proven even to the person making the claims.
When this is justified by the possibility of a catastrophe, it means you’re falling for your own FUD – because you don’t know about the catastrophe, you simply assume it’s going to happen, and that it’s going to be bad enough to warrant trying to avoid it in any case. That’s the Pascal’s Wager part: there’s an exceedingly small probability, but the outcomes are assumed to have infinite reward or infinite punishment, so the expected value of the choice always favors believing in exceedingly small probabilities that would otherwise be rejected as implausible or ridiculous.
In other words, it is a feedback loop where you’re amplifying your own hysteria by imagining worse outcomes and then telling yourself that because it might happen, it is justified to come up with worse scenarios and more FUD, which then paints the situation so dire that it justifies further exaggeration.
That is quite common among people who oppose nuclear power. They seem to run under the theory of “any nuclear accident is by default a nuclear holocaust”. Assume the worst and then some, always. If this was applied consistently to all life, you wouldn’t leave the house.
Green energy is worthless as a source of baseline energy which is needed to power urban areas and industrial sites. Germany found that out the hard way when Merkel went nuts and shut down Germany’s nuke pl;ants and trying to rely on solar.
Worse, both solar and wind have to been taxpayer subsidized because they are not price competitive with fossil fuels. Wind is intermittent enough to be near useless. Take a drive past the Tehachapi wind farm and you’ll find half of them off-line at anyone time and that’s a windy area(some of the time).
Then there is mining of rare earth materials that go into solar panels and wind turbines which is very environmentally destructive. And don’t forget that most solar panels are made in China – a country with no pollution or environmental controls and in fact the world’s largest polluter.
Solar power is awesome where it works, alas, in the subarctic regions solar power is a joke for almost 6 months each year.
Stuff like this is what we really need to be putting research money into.
Nice article, but I think it fails to point out the more historically significant reason for breeding plutonium, and main motivation for building breeder reactors.
Actually no. The desired plutonium isotope one wants is the Pu-239, but keeping the U-238 too long exposed to the neutron flow means that Pu-240 and other undesired Pu isotopes are produced. One gets around this by brief exposure of the U-238 and regularly swapping in new batches of breeding material. This is not easily done in any FBR, barring the RBMK which had the option of swapping fuel rods while the reactor was active, to make it useful for producing plutonium if this were needed (Cold War turning hot).
An FBR is however complete overkill for this, and very clumsy. A simple and cheap graphite pile reactor would be more effective and far cheaper if one’s goal is to produce plutonium for weapons. The Pu-239 for RTGs is also produced in special breeder reactors, not commercial FBRs for this reason.
I *think* the first paragraph is about nuclear weapons, where Pu-239 is the desired isotope, but the mention of RTGs in the second paragaph should be Pu-238.
Yes, you’re right. I guess I have been typing ‘Pu-239’ too often lately :)
Generally, no you can’t have that, because nuclear power is and will be costly even with a closed fuel cycle.
And using nuclear power will generate radiactive waste(operational equipment, etc..), except the double burned and spent fuel will be decaying faster.
And the other question is, for how long will you have that kind of power? Also a wind farms are real, and are producing clean energy and yes they are a compromise, but all those engineer dreams of nuclear energy came with a big caveat, and costing huge amounts of money to develop is only one aspect. These reactors are somewhat prototypisch real.
Windfarms are no better if not worse in the long run when compared to better nuke plants… keep in mind that wind is not a very concentrated or stable power source, so you need a LOT of surface area (that needs wiring btw) covered with bird coppers AND reserve power stations, which are either gas or coal fired. Add to that a fuckton of additional power lines and the need to upgrade existing ones in order to safely deal with the unstable power output.
There’s a good reason wind farms are not financially feasible in most locations without subsidiaries and forcing the distributors into buing power from them.
There’s a fundamental limit of about 1 MW per square mile that can be extracted on the large scale with wind turbines. Adding more will cause the effective surface roughness of the land area to increase, which forms a new boundary layer over the turbines and lifts the faster winds higher up.
In many places there simply isn’t enough space to put enough wind turbines because of this. Hence why all the hoopla about off-shore wind, which is still many times more expensive.
I like this idea, so much safer, pull the power to the fusor and the fission side of the reaction stops cold.
https://www.researchgate.net/publication/255141641_Overview_of_Fusion-Fission_Hybrid_Reactor_Design_Study_in_China
” it’s generally more economically viable to create new fuel out of uranium ore and store or dump the spent fuel after a once-through run in an LWR.”
This isn’t a dig a nulcear as I’m pro nuclear.
But it’s a dig at society, companies, humanity really.
This one sentance explains why we are so fscked right now.
Why climate extremists are becoming a thing
The utter lack of responsibility for waste and waste being something which is cheap therefore preferable to do.
Companies taking no responsibility (suffering no cost!) for the end of life product cycle.
Fix that problem (taxes work better than asking nicely) and many problems we have today are fixed too.
On the bright side, we honestly don’t have a lot of spent fuel lying around at this point, so it’s an easily contained issue. I do agree that we should have done the R&D at least in the way that the Russians have done with the BN-series, as they have working FNR designs that are well past the prototyping and demonstration stage.
I guess that means that we can just buy those FNR plants from the Russians and Chinese (and South Koreans) soon. Anyone up for a few dozen BN-1200s in the US to start things off with? :)
LFTRs, please…
Informative impassioned illusions. We make judgement based not on facts, but on how we feel about those facts.
Solar sounds great until you stop thinking magic rectangles and start looking at the enormous energy required to grow, cut, etch, and deliver wafers that become the cells. Class10 cleanrooms for galiumarsenic ingots don’t come cheap…
20+ years to get net energy is not unrealistic for most install sites. And the panels last about 30 when cared for very well. Hope you got it shipped downhill on a skateboard and have a very short cable run or your instal takes even longer to get net energy.
Reality is rarely strightforward. It’s very simple, but not straightforward.
Spellcheck: lost an “r” in pyroprocessing in the first paragraph after the heading ‘closig the fuel cycle’
I know I cannot spell either, but I;m on mobile, doing it free, and runnjng on the average hacker mindset of 3hrs a night or it cuts into my reading time.
Oh! I’d love an article on radiochemistry if the Ministry of Truth would allow it.
All this closed fuel tech (and associated advanced reactors) is a solution in search of a problem. Uranium supplies are essentially infinite. Aside from better fuel utilization, the supposed benefits are reduced waste volume and longevity. But the volume of nuclear waste is already vanishingly small. As for longevity, it’s already the case that extremely strict regulators like NRC have concluded that repositories like Yucca will contain the wastes for as long as they remain hazardous, so that no significant environmental risk ever occurs. That being an impeccable standard that no other waste stream comes close to meeting. Many other, non-nuclear waste streams remain toxic *longer* than nuclear waste (forever in many cases) and no one seems to be concerned at all.
When will engineers, and people in the nuclear industry in general, understand that the nuclear waste “problem” is not technical, but purely political/sociological. Thus, new technology will not help resolve it. If technical parameters like waste volume and longevity mattered, people would not be concerned about nuclear waste already. So why does everyone think that improving those technical parameters will change things? Anyone who is concerned about nuclear waste now will still be concerned, regardless of how much waste volume or longevity is reduced. Nuclear waste is a *qualitative* concept in the public’s mind. As proof of this, research into deep borehole disposal of a tiny volume of 30-year half-life (fission product only) waste in North and South Dakota was politically opposed, every bit as strongly as Nevada opposed disposal of spent fuel assemblies at Yucca.
Here’s more on why uranium supplies are essentially infinite, as opposed to lasting for only 100 years, as the article suggests.
http://www.americanenergyindependence.com/uranium.aspx
https://www.forbes.com/sites/jamesconca/2016/07/01/uranium-seawater-extraction-makes-nuclear-power-completely-renewable/#5c5e3332159a