It’s hardly a secret that nuclear fusion has had a rough time when it comes to its image in the media: the miracle power source that is always ‘just ten years away’. Even if no self-respecting physicist would ever make such a statement, the arrival of commercial nuclear fusion power cannot come quickly enough for many. With the promise of virtually endless, clean energy with no waste, it does truly sound like something from a science-fiction story.
Meanwhile, in the world of non-fiction, generations of scientists have dedicated their careers to understanding better how plasma in a reactor behaves, how to contain it and what types of fuels would work best for a fusion reactor, especially one that has to run continuously, with a net positive energy output. In this regard, 2020 is an exciting year, with the German Wendelstein 7-X stellarator reaching its final configuration, and the Chinese HL-2M tokamak about to fire up.
Join me after the break as I look into what a century of progress in fusion research has brought us and where it will take us next.
Previously in Pursuit of Nuclear Fusion
The discovery that the total mass equivalent of four hydrogen atoms is more than that of a single helium (4He) atom was made in 1920 by British physicist Francis William Aston. This observation led to the conclusion that net energy can be released when one fuses hydrogen cores together, for example in the common deuterium (2D) tritium (3T) reaction:
Deuterium (2H, or D) is a common, stable isotope of hydrogen that occurs naturally in abundance, to the rate of 0.02% of the hydrogen in Earth’s oceans. Tritium (3H, or T) is an unstable, radioactive (beta emitter) isotope of hydrogen, with a half-life of 12.32 years. Tritium is formed naturally by the interaction with cosmic rays, but can be easily bred from lithium metal, either in a breeder blanket in a fusion reactor, or from a fission reactor that uses heavy water (deuterium), like Canada’s CANDU reactors.
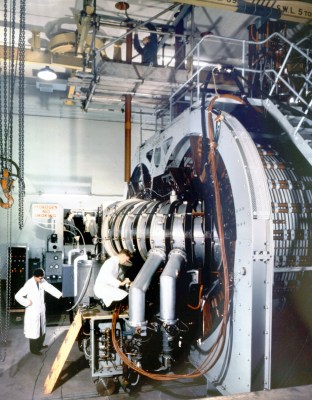
The first neutrons from nuclear fusion were detected in 1933 by staff members of Ernest Rutherfords’ at the University of Cambridge. This involved the acceleration of protons towards a target with energies of up to 600 keV. Research on the topic during the 1930s would lay the foundation for the development of the first concepts for fusion reactors, initially involving the Z-pinch concept which uses the Lorentz force to contain the plasma.
Teams around the world worked in absolute secrecy, with all fusion-related research being classified. The British created the ZETA stabilized pinch fusion reactor, with the hope that this would prove to be a viable blueprint for commercial fusion reactors. Unfortunately, ZETA proved that the Z-pinch design would always suffer from instabilities, and by 1961 the Z-pinch concept was abandoned.
Meanwhile, the Russians had developed the tokamak reactor concept during the 1950s, partially based on the Z-pinch design. The tokamak design proved to be able to suppress the instabilities that had plagued Z-pinch reactors, as well as early stellerator designs. These days, most fusion reactors in operation are of the tokamak design, though the stellarator has seen a resurgence recently, especially in the form of the Wendelstein 7-X project.
What We Can Expect to See in 2020
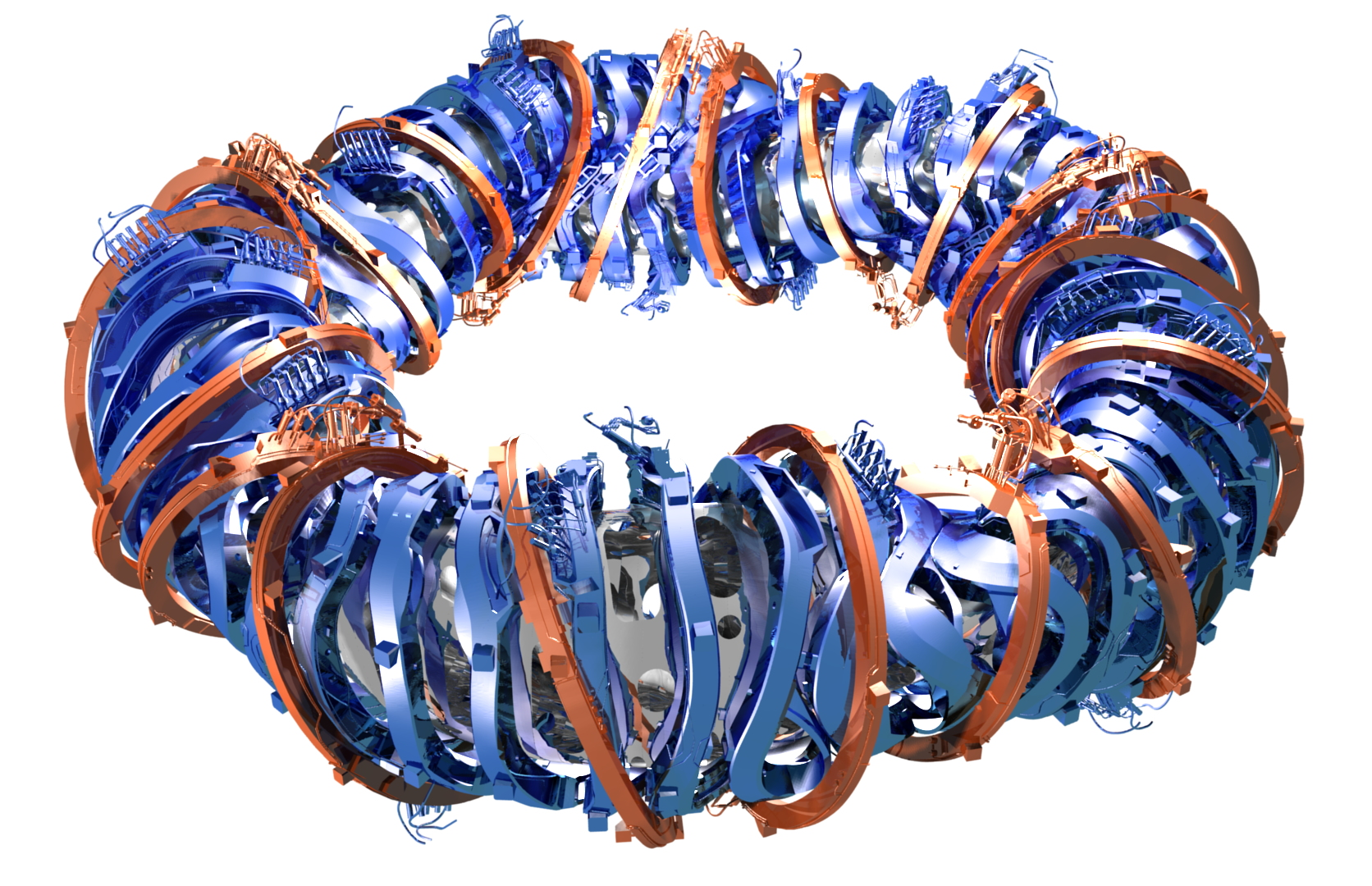
As mentioned in the article introduction, Wendelstein 7-X has reached an important milestone. Since we first wrote about this project back in 2015, the project has worked through all of its targets except the final one: cooled divertor operation. The reactor is currently being upgraded with these divertors which should theoretically allow for steady-state operation, allowing for impurities to be removed from the plasma during operation.
Installing the new divertors and cryopumps will continue into much of 2020, involving the running of a 55 meter long transfer line to the cryoplant, along with the installation of new storage tanks for helium gas and liquid nitrogen. With some luck we’ll see the first tests of the new system this year, but most likely the first continuous operations of WX-7 will take place in 2021.
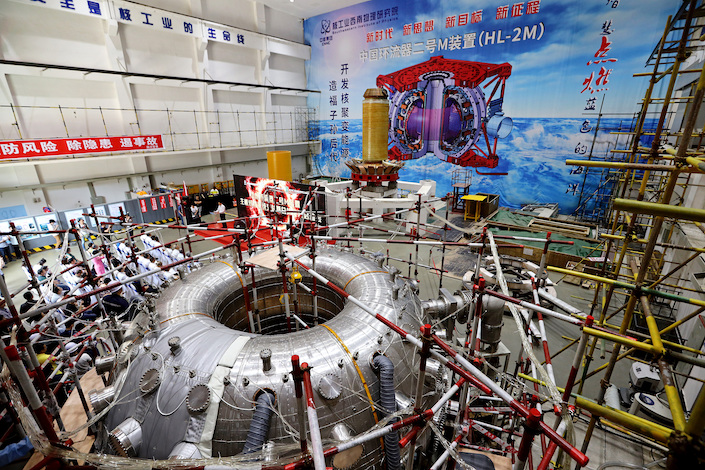
Over in China, the final touches have been put on its HL-2M tokamak, which is the latest in a range of tokamak designs since the 1960s. The HL-2M is the new configuration of the HL-2A tokamak, one of three tokamaks currently in use in China (EAST and J-TEXT being the other two). HL-2M has seen big changes to its coil configuration that allow for the creation of many types of plasma, along with the testing of various types of divertor configurations. This year HL-2M will see its first plasma.
Depending on the performance of HL-2M, it will allow for the CFETR (China Fusion Engineering Test Reactor) project to start its construction phase in the 2020s. In its first phase, the CFETR tokamak would demonstrate steady-state operation and tritium breeding. In its second phase CFETR would be updated to allow for a power output of 1 GW (compared to ITER’s 500 MW) and a fusion gain (Q) higher than 12.
Fusion is Hard
The main reason why nuclear fusion is taking so long compared to nuclear fission is that the former requires the ideal conditions to even occur, let alone persist. This means high temperatures, high pressures, high currents, or a combination thereof. Even then plasma containment is notoriously complicated, as the plasma isn’t a nice, calm gaseous state that’s just kinda floating there. Rather it’s a seething collection of plasma at high pressures and many millions of degrees Celsius that’ll breach through the magnetic confinement any chance it gets. Although significant progress has been made here, the coming years will tell whether we’ll avoid a Z-pinch-like dead-end with the tokamak and stellarator designs.
Many aspects of running a nuclear fusion reactor remain somewhat of a mystery, much as it was with the first fission reactors, where early generation II designs saw countless changes to materials used, along with fundamental changes to the overall design to improve performance and safety. Although fusion reactors are less challenging in this regard, they do have to deal with the neutron bombardment of materials in the core, which can weaken them. This also leads to the only waste that is produced by fusion reactors: the reactor core itself.
A combination of activation through this exposure to neutrons, along with contamination with tritium will render core materials radioactive, requiring handling of the resulting low- to intermediate- level radioactive waste like steel and other building materials at the end of the reactor’s lifespan. Fortunately, studies have shown that intermediate storage of up to 100 years is sufficient to render these materials safe.
Regardless, the neutron exposure is one aspect which can likely be dealt with in a more direct way through material selection or through neutron capture mechanisms in future reactor designs. As China’s program evolves through HL-2M into CFETR and ITER into DEMO, the hope is that we can catch any issues and make improvements before fusion hits prime time.
Low Energy Nuclear Reactions
Tangentially related to fusion, LENR is what used to be called ‘Cold Fusion’. Marred by decades of ridicule, a number of scientists have nonetheless persisted in examining the phenomenon that lit up the world with promises of fusion power at room temperature. Dismissing the original theory of hydrogen atoms fusing, the current theory is that protons and electrons can be merged to form neutrons. For a detailed overview, see for example this video presentation by Prof. Peter Hagelstein, an MIT associate professor.
According to the Widom-Larsen theory, the reason why the original 1989 experiment was so hard to recreate is because it relies on hydrogen atoms settling on active sites on the palladium (or equivalent) layer. This means that one needs to create a suitable surface on a nano level, something which was not realistic in the 1980s.
Even if LENR never turns into anything more than a curiosity, it does give us a glimpse in another way that atoms seem to behave. Best case it might provide us with ways to improve fusion reactors in ways that we cannot fathom today, by lowering pressure and temperature requirements.
Energy Dominance is the Game
A commercial fusion reactor would be essentially the pinnacle of energy generation, barring the development of anti-matter reactors or such. As an energy source, its fuel is practically unlimited, with the deuterium-tritium type having enough fuel on Earth for millions of years, and the deuterium process version offering billions of years of energy production using just the deuterium available on Earth. The first nation to master this capability stands to gain a lot.
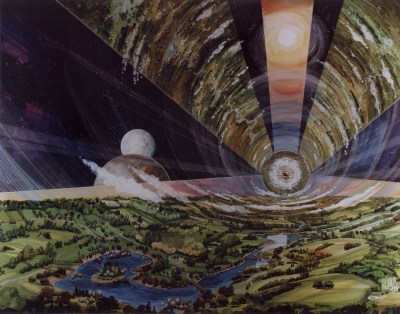
Fusion reactors are inherently safe, due to the complexity of maintaining the proper conditions for the fusion process to take place. During operation, no waste is produced, but instead very useful helium (4He) is created which has countless applications in everything from industry to running MRI scanners, to filling up party balloons. While nuclear fusion will very likely co-exist with nuclear fission (possibly using FNRs) in a complementary fashion, the former being ideally suited to handle electricity and heat generation for all population centers on Earth.
Exactly when we’ll see the first commercially viable fusion reactor appear is hard to tell at this point. With ITER not projected to see first plasma until 2035, we might see China with its CFETR and successor reactors reach the point of commercial viability by the 2030s or 2040s. Yet as the UK learned with ZETA, in plasma physics nothing is certain.
[Main image: Experimental visualization of the field line on a magnetic surface in Wendelstein 7-X. CC-BY 4.0, Wendelstein 7-X team]
Nice article!
It would be fun with a follow up regarding the large undercurrent of more or less wonky fusion projects that’s floating around out there.
+1
Even better, let’s propose a new concept: The “Fisi-Fusi Oscillo-Reactor”.
Use fission to break the nucleus, then glue the fragments back together using fusion, then break it again, and so on. Unlimited energy from a single atom!
:o)
Unfortunately, the atoms in fission output and and fusion input are not the same.
However the perpetual movement exists with other ingredients: the more beer you drink, the more peanuts you need, and the more peanuts you eat, the more beer you need (scientist: J.-C. Vandamme :o).
Thats not how it works, not that it will work but only heavy elements can fission and only light elements can fuse.
Some waste is produced from fusion, even D-D, when neutrons activate materials in the reactor.
Try reading the whole article, not just the first paragraph…
“Although fusion reactors are less challenging in this regard, they do have to deal with the neutron bombardment of materials in the core, which can weaken them. This also leads to the only waste that is produced by fusion reactors: the reactor core itself.”
“the miracle power source that is always ‘just ten years away’”
I thought the most practical nuclear fusion power source is 8 minutes and 20 seconds away!
+1
Sure! got a power cord 92 million miles long?
No need.
We already figured out wireless power transmission with a currently available efficiency of up to 23% (and climbing).
Ever seen a solar panel?
which translates into 230 watts per square meter in optimal situations. In sub-optimal, you are lucky to reach 100W/SqM. In subarctic regions sunlight is non-existant in the 4 winter months, incidentally when the electricity demand is the highest. Not to mention solar panels lose efficiency with age. So yeah, solar panels work, might even be viable for a part of the population, but by no means is it viable for the whole population.
INVENTION
by Shel Silverstein
I’ve done it, I’ve done it!
Guess what I’ve done!
Invented a light that plugs into the sun.
The sun is bright enough,
The bulb is strong enough,
But, oh, there’s only one thing wrong…
The cord ain’t long enough.
Only if you can travel at lightspeed.
But of course that’s not the problem: Energy transfer with EM waves is quite efficient. But it is only available half the time at max. less in bad weather periods.
Even if nuclear fusion becomes technically possible, it may not be commercially viable. We have already seen with nuclear fission plants that builders want guaranteed electricity prices in order to safeguard their large investment. Fusion plants work better at larger scale, so building small ones is not an attractive proposition.
Of course, if there was a source of “cheap, unlimited and clean” energy it would be a disaster for the planet. We currently are using messy and inefficient fossil fuels to destroy the planet, just think how much worse it would be with even cheaper energy.
Well by the time we fiiiinally figure out how to sustain a reaction that produces an energy surplus we might have a slightly different business culture and economy than we do now. Much in the way that automation is eventually going to make work unnecessary for a large portion of the populace due to no fault of their own, we will have an impetus to generate power in a way that may not necessarily be commercially lucrative. We might even want to—gasp—nationalize it. Imagine that.
Not following you at all about how it would be a disaster for the planet. It would ideally get rid of all the messy fossil fuels. Do you think that the energy generation itself is what harms the planet? What’s your reasoning there?
I can imagine there’s an environmental effect from the energy that’s released as heat from vehicles, machinery, poorly insulated buildings, etc. I’m sure it’s well below the noise floor caused our greenhouse gasses though, and probably only directly observable in cities. Not sure if that’s what bleepblorpthesnake was talking about.
“automation is eventually going to make work unnecessary for a large portion of the populace”
That’s just totes adorbs!
Tokamaks work better at larger scale – especially when they’re being subsidized by the state.
The W7X is actually pretty tiny.
you are comparing apples to oranges here… a fusion plant can’t have a catastrophic meltdown, it will not need a large reinforced concrete dome with meter thick walls, it will not need a crapton of reserve power generation just to keep pumps going and given that the trithium amounts present on site will be small, there is going to be a LOT less red tape that makes a fission plant so expensive and slow to build.
A far less expensive powerplant with the promise of actually clean energy and a reasonable ROI (when compared to fission) has a good chance of getting enough private investments to not need state intervention.
But right now we just need to get to a point when someone can actually be sure it would even work from the physics/engineering point of view. So far there’s only a good chance of that, no guarantee. THEN we can start worrying about the economical side of things…
I dare say the shield dome around the reactor will be a necessity also in the future unless mankind magically changes it´s inherently self destructive behaviour.
It is not the energy, what is considered to “destroy the planet” by the climate hysterics, it is the waste product, the CO2, what is considered harmful. You do not have this with fusion.
Generating power using fission still requires a source of cold, which I’m guessing is going to be mostly sea water, like other energy produced using steam turbines. Eventually dumping that much energy into the environment is going to increase the amount of water vapour in the atmosphere which is currently the highest greenhouse gas in terms of weight and by volume and it is the largest contributor to Earth’s greenhouse effect :)
Luckily it takes a hell of a lot of energy to increase the amount of water vapour in the atmosphere, but it is one of those things that you take for granted.
I don’t see how that’s different than how every power plant already works. They already emit that steam from their turbines. We’d be simply taking every other emission out of the equation. I don’t believe that water vapor due to running steam turbines is a significant factor in climate change. It can also be simply re-condensed, or some other Brayton cycle system could be used to run a generator.
heat pollution is a thing, its just not a thing we really have to worry about right now. when fusion enables the whole planet to use as much power as the west currently does, then it might be a problem.
I think you mean “The East” currently does. How many reactors is China building at the moment?
im talking per capita here. quality of life is certainly on the rise in the east, and the power bill with it.
Bingo.
No significant amounts of Helium will be generated by fusion. One reactor would make some kilograms per year. MRI machines worldwide consume about 7000 tons of it per year.
LENR is just pseudoscientists shifting the goalposts after it was shown time and time again that there’s not enough energy to fuse atoms in anything less than sun-like conditions. The whole “field” suffers from credibility issues because even tiny amounts of power generated by these devices would be immediately noticeable as fatal levels of neutrons coming off of them.
That’s wayyyy oversimplified. We aren’t talking about fusing the same particles that the sun does, which is enormously more difficult than many other far more accessible reactions. One of the reasons for this is the Lawson criterion:
https://en.wikipedia.org/wiki/Lawson_criterion
The primary reaction happening in the sun is proton-proton fusion. Basically, intense gravitational confinement manages to convince four protons to spontaneously fuse together into an atom of helium-4. The Lawson criterion of that reaction is way off the charts. Thousands of times higher than, for example, deuterium-tritium fusion, which has an L-C of 1. Nobody on earth is seriously attempting to accomplish proton-proton fusion like the sun uses. For that reaction, you’re probably right; nothing short of the center of a star would be adequate to create enough of those reactions to be worthwhile. Luckily there are reactions orders of magnitude easier than that one. Still hard, but we’re making progress.
Different reactions also have different levels of neutrons, and some are aneutronic. Plus, neutrons can be mitigated. The inside of most powerplants would be fatal to a human (even fossil fuel burners), but since fusion doesn’t create long-lasted radioisotopes you can just cover it with shielding and it’s pretty much fine. Eventually parts inside would suffer from neutron embrittlement and become lightly radioactive, but as the article says you’d be able to just bury those somewhere for less than a century and they’d be safe again. Then it’s just a question of using a waldo or a few for maintenance. Would certainly release a lot less radiation than oil and gas currently does.
“Then it’s just a question of using a waldo or a few for maintenance.”
So, where are we going to find a waldo?
B^)
FYI a Waldo is just the name for the crane-like remote manipulators used to handle radioactive materials.
https://en.wikipedia.org/wiki/Remote_manipulator
I noticed this misconception in the article too.
Helium -4 has TWO protons, and two neutrons.
So it’s 2 hydrogen atoms fusing to make helium, not 4.
It’s all fraud, of course, but a very useful and productive fraud indeed. The entire field of superconductive magnets was benefiting from the otherwise useless fusion research.
Dont forget for lenr, the the Widom-Larsen theory is… still just a theory. All we know is that something odd happen between metallic lattice (paladium, nickel) and hydrogen/deuterium. Phenomena causing thing such as additionnal heat and isotope change.
Your attitude is not helping and pretty un-scientific (although pretty much aligned with 99% of the scientific community).
This is science, you need to keep an open mind and that mean paying attention to facts. Sheeping around wont do any good.
The word “stellarator” is misspelled in the article.
My prediction is that fusion is doomed to be a niche power source, mainly used in space and other remote areas. The reason is simple. Assuming, optimistically, that fusion is commercially viable by 2040 that is 10 years after most people agree we need to have moved our electrical grid to renewable sources like wind, solar, etc. If there is a significant amount of fossil fuel generation to replace in 2040 it is unlikely human civilization will be able to support the technology for very long. If the grid is predominantly renewables and storage then it is inconceivable that early fusion plants will be cheaper per watt than solar cells, etc. Thus fusion becomes the choice for locations like Antarctica and the moon where harsh environments and high energy demands coexist. Fusion may also find a niche in providing industrial scale power for things like aluminium smelters and steel furnaces.
As weird as it seems, fusion would not be much usable in space…
Why? Heat management… Down here on Earth, we have massive thermal masses all around us. The atmosphere, the sea, lakes, rivers… There we can use heated gas, dump the heat, restart the cycle over and over. In space, there’s nothing.
Yes, I know space is cold, very cold, but you don’t just dump heat into the vacuum. There’s no convection, there’s no conduction, only radiation, and that is the least efficient heat transfer. That’s why the ISS have big, big radiators to get rid of heat.
Now imagine a spaceship having to get rid of megajoules of heat using just radiators, in the vacuum…
You’re assuming a space station or ship has to be a closed system.
If you’re willing to carry water, ammonia, or some other sufficiently thermally dense material as a consumable you can operate at higher thermal ratings. If we have fusion on Earth, fuel to get to space is much cheaper if not ‘free’ so why not send craft to europa or the asteroid belt for the sole purpose of bringing back ice / brine to dump overboard as thermal waste?
Basically Space steampunk.
If we are mining brine from Europa, why not mine methane? Keep the generator outside, feed methane and oxygen to it, let all the CO2 and heat be sent away…
It’s cheaper, easier, have less moving parts, and you don’t need to heat brine and jettison it outside.
Attempt no landings there…
Fission is *already* the preferred energy source in the Antarctic, has been for quite a while. If only there were light on the moon, we could use solar power. Oh, wait a minute…
Without a few yet unknown inventions in cheap energy storage technologies this scenario will probably stay a wet dream of the climate hysterics.
High temperature fusion will never work and the proponents of same are frauds and crooks!
Look up – it works rather well. It will never work on Earth in a stable way.
High temperature fusion has already worked. See Hydrogen bomb
If you are talking controlled high temperature fusion. well, that has worked too. Jet has achieved generating 16 MW of fusion power, however that required 24 MW of energy input. But JET was never designed as a functioning power station, but a test bed for fusion technologies. Enough progress was made to build ITER will will attempt to make the next step of power generation
The problem is not physics, but engineering, and huge progress has been made. That progress has also other applications outside fusion which makes the experiments worthwhile in itself, without the promise of almost limitless clean power source
“agree we need to have moved our electrical grid to renewable sources like wind, solar, ” Yeah, like that works …. Just a few days ago we had zero wind in the entire state (all wind turbines were actually drawing a bit of power) and since it was night, no solar…. and it was very cold…. Thank goodness for natural gas, coal and other reliable resources.
This is a problem with most “renewable” sources. (I think term is somewhat misleading “alternative” is much more accurate)
To a large extent alternative energy works well for a few people, off grid homes and small communities. But, when it is scaled up to meet the demands of the majority it suffers the same environmental impact of burning fossil fuels. There is the waste batteries (lead and lithium) the raw materials to construct them. The concrete production to build the wind turbines foundations (concrete production is one hell of a co2 emitter) the processing of the materials to make all the associated stuff.
I’m all for alternative fuels and ways of doing things, but while the population keeps increasing the problem will only get worse.
Thermal storage is very cheap, we just need more incentives to invest in it.
It may be relatively cheap, but it is not efficient, very lossy. You get about 20% efficiency with a PV panel. Which is not very much but good enough. And then you waste 2/3 of this energy with thermodynamics? Not a good idea.
oh look, another fusion article without any polywell love.
I loved Polly _Very_Well_ ;)
Happy now?
I was hoping to see more about that as well.
This company seemed promising a few years ago, but they went into limbo…
http://www.emc2fusion.org/
https://www.microsoft.com/en-us/research/video/polywell-fusion-electrostatic-fusion-in-a-magnetic-cusp/
The Z-pinch path has not entirely been abandoned. LPPFusion is still working on this approach.
https://lppfusion.com/
I can’t say if it is a viable path, but it is still around.
Ill just leave this here: https://www.helionenergy.com/
Practical nuclear fusion is only 10 years away, and always will be.
I dismantled a Z pinch in 1987 as a summer job, so clearly it wasn’t entirely abandoned in the 60s.
But, away from facts and on to philosophy:
We need fusion. We need it badly.
It seems we can just about get by with renewables by backing back from where we are now.
But that’s not a long term answer. We need orders of magnitude more energy for when the mines run dry, to reprocess our waste dumps back to raw elements to re-use.
And to pull the CO2 back out of the atmosphere.
And to get ourselves and our eukyrotic friends off if of this doomed rock.
“We need fusion. We need it badly. ”
Anything worth doing, is worth doing badly.
-G.K. Chesterton
https://en.wikipedia.org/wiki/Muon-catalyzed_fusion
You just have to find a way to produce cheap muons… but then again, if you could do this, you could as well directly move on to producing cheap anti-protons and use anti-matter annihilation reactors instead of fusion…
“… its image in the media…” and only complete fools believe anything they get fed by the media who are both incompetent and dishonest.
A senior person in a UK firm, that just got an extra USD $100 million in funding, let slip that their commercialisation target is 2025. Then the video of that was taken off YouTube within 24 hrs, so either he made a mistake or let slip a very juicy secret.
I found another copy of the video, they really did cite 2025 as a target date, https://www.youtube.com/watch?v=Z_G_aodzVhs
YARG this again? Fusion is not going to be a practical power source any time soon, for values of “soon” comparable to a human lifetime.
The aforementioned neutrons are not just a detail, they are a major show-stopper. They are extremely plentiful, extremely difficult to mitigate because uncharged particles only interact with atomic nuclei, and aneutronic reactions are a pipe dream when we can barely break-even on D-T. It’s also very likely that if we ever did try for an aneutronic reaction it would be plagued by unstoppable side reactions that aren’t aneutronic, much as Castle Bravo ran away beyond its target yield because nobody realized that he harmless inert contaminant lithium-7 would fission to lithium-6 and participate in the active reaction. Because of, drumroll please, all the neutrons.
The first clue that Pons and Fleischman had not done what they thought they did was that they were still alive, since their kit was unshielded.
The real reason the fusion pipe dream gets watered so much is that at a high level, powerful but uneducated people are persuaded that the basic research might produce another breakthrough like the Manhattan Project did. But it won’t. We understand what happens at these energy levels pretty well now, and improving our control is just an incremental process. And we are many, many increments from being able to build a useful power plant of any scale.
Also, I didn’t see it mentioned this time but it bears repeating that fusion is NOT the power source behind hydrogen bombs. Those bombs USE a fusion reaction, which provides about 10% of the energy output, but on top of that and 10% or so from the fission trigger most of the bomb’s energy comes from the fissioning of the bomb’s casing of cheap depleted uranium by, drumroll please, all the neutrons from the fusion reaction. If you build a bomb that starts the fusion reaction but doesn’t use the neutrons to make more energy through fission, you still have a real weapon; look up “neutron bomb.” A fusion reactor, if we could build one, would basically be a continually running neutron bomb whose own hardware would be at ground zero.
Bollocks! https://lppfusion.com/
What did you smoke?!?
Why don’t the super cool the materials and then compress them throw a Neutron at the mix and see what happens!
That is how laser fusion targets are handled, frozen.
They could have skipped all that investment and just asked me.
It looks like you are a danger to others and yourself.
The reason the cold fusion result was unreplicatable is … do I really have to spell it out?
“of Rutherford’s”? Of Rutherford’s what?
The difficulty of making fusion work at all is why all examples in Science Fiction of fusion reactors exploding are silly. So what if the space warship takes damage to the power room? All that needs to be done to keep things from going really bad is to cut off the fuel feed to the reactor and it *stops*.
A properly designed fusion reactor, for any use, will have several fail safes that cut off the fuel feed.
The only fictional style of fusion reactor that could possibly have a catastrophic failure mode is one using artificial gravity to compress the fuel gas. If the gravity field failed, that would trigger a fuel cutoff. How big the boom could be would depned don how much gas is compressed inside the reactor at any instant. So build the room around the reactor extra strong, with some blowout panels or vents, with everything covered with heat resistant, smooth panels so the escaping gas can quickly get out. Such a design would have multiply redundant containment with power storage right at the reactor to maintain containment for the very short time fusion would continue after damage or other emergency caused the fuel to be shut off.
In other words, the fusion engineers in David Weber’s Honor Harrington books are a bunch of crazy loons who deliberately designed their fusion reactors to extremely unsafe.
Where’s the excitement in not ejecting the “Warp Core”?
OK, so it would fictionally explode, if the fictional anti-gravity field breaks down. :-)
In reality, the energy density of gravitation is much lower than that of the electromagnetic force. So I expect no higher energy release from such a confinement system in case of a breakdown than from magnetic confinement. Apart from the fact, that we do not have any idea about artificial gravity anyway. Luckily magnets work and are well understood.
Judging from the levels of ignorance on display in these comments, save perhaps ‘localroger’, it is a very good thing that fusion will always be 10 years away.
To cite only the simplest example, there are no “sources” of energy. Energy is conserved, always and absolutlely. What we humans value is power – the transformation or movement of energy. In particular we value the release of energy from a bound form (chemical or nuclear bonds) to a free form (electromagnetic radiation or particle kinetic energy).
Apart from the purely technical ignorance, there is social ignorance. Nuclear reactors of any kind are no solution to global warming. Global warming is the obvious and inevitable consequence of two immutable physical laws and one inescapable human trait.
The two laws are the law of conservation of energy and the black body radiation law. The human trait is the insatiable desire for power. The latter has been satisfied during the last two centuries through the release of solar energy stored in fossil fuels. Yes! fossil fuels are a form of renewable solar energy. It just takes many millenia to renew, that’s all. Obviously when you release millenia of stored energy in a little over a century, things are going to warm up. The attendant water vapor and carbon dioxide certainly helps impede the inevitable black body radiation of that energy into space, but the prime mover is the rate of release.
If you continue that same high rate of release, or worse, increase it, using nuclear processes, you have solved nothing. The only workable solution is clear. Each individual needs to throttle their desire for power to that which is necessary to be comfortable and the total human population residing on earth needs to be limited or even reduced.
I don’t see any of that happening in my lifetime. Finally, to go out on the precarious limb of prophecy, I do not believe either the tokomak or the stellarator can ever work.
By the way, the primary process in the sun is the carbon-nitrogen-oxygen cycle, not hydrogen-hydrogen, which occurs with very low probability, although the latter is necessary to create the precursors to the predominant cycle. The minor reaction occurs because there is a very very large amount of hydrogen in the sun.
And bye the bye, nuclear reactions are not restricted to the exothermic reactions that myopic humans so idolize. In addition to low energy nuclear reactions, there are, in fact, endothermic nuclear reactions. The Pons-Fleischmann “excess” energy, if real, was probably a consequence of the deuterium combining with the palladium followed by a sequence of alpha and beta emissions until a stable isotope was reached. Palladium occupies a uniquely suitable position on the knee in the isotope chart for this, and like platinum, it can absorb nearly one hydrogen for every palladium atom in the lattice, so the probability of a reaction occuring becomes non-negligable. It is a useless reaction for power production. You could fit the global supply of palladium in a good sized garage. The reaction is, nevertheless interesting in its own right and worthy of level headed investigation.
TL;DR: Humans are somehow generating the heat that’s responsible for global warming, and any further power generation will make it worse. But something about cold fusion…
Our current problem isn’t so much the release of “millenia of stored energy in a little over a century”, it’s the release of millenia of stored carbon over approximately two centuries. And the attendant messes from finding, extracting, transporting and burning that much fossil fuel.
Great article. I was especially grateful for the update on Wendelstein. A small point- the Iter website predicts first plasma in 2026; the article gives 2035 – please correct or clarify.
As an aside…
There are many types of incinerator, for disposing of waste. Some require extreme temperatures to eliminate hazardous chemicals. I’m thinking of Poly Chlorinated Biphenyls or PCBs as an example.
I’m sure plasma would destroy any harmful chemicals. I’m just wondering what impact those impurities would have on a fusion reaction.
Could we have a similar article on the latest fission technologies? It really does seem as if nuclear is a viable contender for our increasing electrical power needs.
It would seem that inertial confinement is a less complicated approach to achieving the conditions for fusion, and several indies are quietly pursuing that approach, although on miniscule budgets compared to the big international projects.
https://www.youtube.com/watch?v=k3zcmPmW6dE
https://thebulletin.org/2017/04/fusion-reactors-not-what-theyre-cracked-up-to-be/
Exactly
IIRC didn’t Teller propose a simpler (relatively) design for a linear fusion reactor in his later years, not as fancy and not providing as many years (decades) of lucrative research grants…. so not popular(?)
Grants are not the sole province of research, if there was workable Teller idea, it has been or is being investigated. Probably another dead end as fusion all are.