Of all the elements that make up the Earth’s crust, uranium is reasonably abundant, coming in at 49th place, ahead of elements such as tin, tungsten and silver. Ever since humankind began to exploit uranium for its fissile properties in energy production, this abundance has also translated into widespread availability for mining. As of 2019, Kazakhstan, Canada and Australia formed the world’s main producers, accounting for about 68% of output.
Considering the enormous energy density of uranium when used as fuel in a nuclear fission reactor, the demand for uranium is relatively low, especially combined with the long (two years on average) refueling cycles of commercial reactors. The effect is that even with the very inefficient once-through fuel cycle – which only uses a fraction of the uranium fuel’s potential energy – uranium market prices have remained relatively low and stable even amidst geopolitical crises.
Despite this, the gradual rise in uranium market prices ($10/lb in 2003, $49/lb in 2022), as well as the rapid construction of new reactors is driving new exploration. Here recent innovations may make uranium fuel even more accessible to all nations, by unlocking the billions of tons of uranium found in plain seawater as well as the many tons of fly ash produced by coal plants every single day.
It’s The Economy, Silly
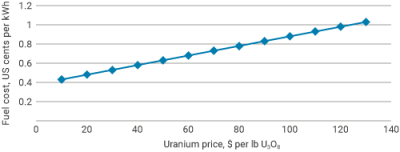
The primary reason why most nuclear plant operators opt for a once-through fuel cycle that only uses a fraction of the fissile U-235, is that the fuel costs for a nuclear plant are so incredibly low. While the up-front costs for a new, GW-level nuclear power plant are considerable, the operating costs over its 40-100 year lifespan are very low, which is where the added cost that reprocessing spent fuel, to remove the newly formed actinides and transuranics, makes little economic sense.
This economic angle is also one of the factors that has made the development of fast neutron reactors (FNRs) unattractive. While these FNRs can breed their own fuel from fertile isotopes through neutron capture, they are more complicated and expensive than a light water reactor — virtually all commercial nuclear plants in operation today. These factors all play a role in whether certain uranium resources are economical to extract from the ground or other sources, while making it quite obvious why extracting uranium from fly ash and seawater wasn’t economical before.
Even though the Earth’s seas and oceans contain an estimated 4.6 billion tons of uranium, the dilution of it into these vast waters means that that you have to filter out a substance of which there are only a few molecules per billion. Similarly for fly ash, separating the uranium from the other components in the ash has to be done in a way that is efficient enough to compete with existing mining methods, such as in-situ leaching (ISL).
However, another potential benefit of extracting uranium from seawater and fly ash is that it can fully avoid the environmental impact of traditional mining, while potentially helping with addressing the environmental hazard posed by fly ash.
Coal Waste Problem
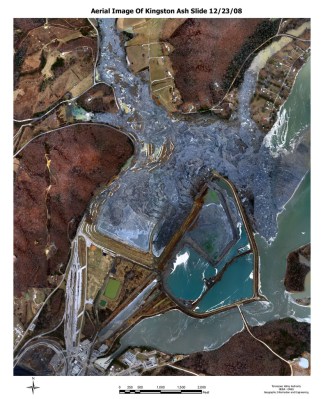
Coal ash consists out of the ash that remains at the bottom of the boiler along with the fine ash, or fly ash, that is captured by electrostatic precipitators or similar equipment installed in or near the coal plant’s chimneys. It contains some left-over carbon, along with large amounts of silicon dioxide, aluminium oxide and calcium dioxide. In addition, it contains trace elements of many heavy metals and similarly problematic substances, including arsenic, cadmium, hexavalent chromium, lead and mercury.
Finding a way to recycle or process fly ash has been an increasingly problematic topic, especially now that fly ash is generally not released into the atmosphere any more, but instead stored in massive coal ash ponds. These ponds form a potential hazard, as illustrated by the 2008 Kingston Fossil Fuel Plant slurry spill, that released 4.2 million cubic meters (1.1 billion US gallons) of fly ash mixed with water into the adjacent Emory River. The subsequent clean-up cost the lives of approximately 40 workers from exposure to the hazardous chemicals.
While fly ash is increasingly mixed into everything from concrete to tarmac and toothpaste, the sheer amount produced means that a significant amount of the approximately 34.7×106 tons of fly ash produced by coal plants in the US alone each year ends up in landfills. Mining fly ash for useful elements like uranium could help with reducing its total volume, while potentially making it easier to use the left-over material.
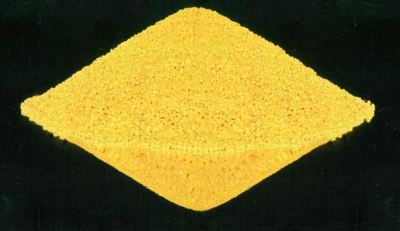
As early as 2007, Sparton Resources from Canada reported producing yellowcake (mostly U3O8) from fly ash from a Chinese coal-fired plant. Uranium levels were found to be about 160 ppm, corresponding to about 0.2 kg of yellowcake from a ton of ash. As noted by The Economist in a 2010 article, this compares to the 1,000 ppm or more in uranium ore. Using a process involving sulfuric and hydrochloric acid that is reminiscent of in-situ leeching, the uranium and other dissolved elements are then filtered out and precipitated using ammonium carbonate.
At that point in 2010, Sparton claimed to be able to extract a kilogram of uranium this way for $77, with the uranium spot price market then being $90. Most of the R&D on this topic appears to occur in China, where it is considered a viable source of uranium fuel, especially in light of the more than hundred new nuclear reactors China has planned or is in the process of constructing, and its focus on self-reliance.
Sun et al. (2016) describe the extraction of uranium from bottom ash, and the high concentration of uranium (374 mg/kg) in the bottom ash remaining after high-germanium containing coal was burned. Although uranium mined from CFA isn’t a big industry yet, ongoing research and small-scale trials as described show the viability of this approach.
Mining The Oceans
The concept of filtering uranium out of sea- and ocean waters is a simple one: essentially it comes down to pumping as much water as possible through a filter system that will retain the elements we are interested in, after which the uranyl molecules can be further processed into reactor fuel. Where things get tricky is that although the oceans contain hundreds of times more easily accessible uranium than dry land, the low density of a few particles per billion (ppb) makes it essential to have a highly efficient filtering method.
As noted by a 2017 Stanford article, the approach used at that point involved sticking plastic fibers containing a compound called amidoxime into the water and waiting for the fibers to become saturated with uranyl, which can then be processed. In 2018, PNNL reported recovering a whole gram of yellowcake from seawater this way.
In a study published last year in Nature, Yang et al. (2021), demonstrate an alternative approach where seawater is led through a hierarchical porous membrane, not unlike the branching network of a lung or blood vessels. By coating the insides of the thus formed channels with amidoxime and forcing uranium-containing water through them, they demonstrated a significantly improved extraction rate over previous attempts, mostly due to the massively increased surface area, and the increased flow of water across the amidoxime-coated material.
Once saturation has been reached, the uranium can be dissolved using hydrochloric acid, following the usual processing approach into fuel for reactors. The thus cleaned membrane can then be reused many times, making it a potentially economical option, and a way to extract uranium for one’s own needs for any nation that has access to a body of sea- or ocean water.
Not Running Out Soon
Unlike fossil fuels, which are beginning to see some significant production drops as many of the best oil, coal and gas fields have been exploited to the point of exhaustion, fission reactors using only uranium as fuel can sustain human society at its current energy levels for thousands of years, even without significant investment in the reprocessing of spent fuel, or in fast neutron reactors. Perhaps even more tantalizing is that it adds the cleaning up of the toxic waste created by coal plants as a feature of this transition.
In addition to exploiting fly ash for uranium, some researchers are trying to determine whether rare-earth elements can perhaps also be economically recovered from these ashes. In this way the pollution of yesterday may conceivably help power the future, in an ironic twist.
Banner image: Chien Wai of PNNL holding up vial with 5 grams of yellowcake recovered from seawater.
I’m more surprised that they’re not using reverse osmosis and the like to filter out Lithium. It is said that there is more lithium In the the sea than on land. So why not go after both lithium and Uranium from sea waterwater? Right now Israel is thinking And doing Desalination for drinking water Using the mediterranean sea As it source. They have to dump the salt water back into the mediterranean after harvisting the water to drink from it, Which has a high salt content, Which also contains probably uranium and Lithium, and gold too. Here in the US we also have that same problem with high Salt content When processing sea water For drinking water, such as what we are doing in southern California. We are pouring the Higher density salt water back into the sea, Which has it’s own issues when not done correctly. Mexico already harvest sea salt from the sea on it’s west coast. Makes me wonder how much uranium, lithium and gold is being missed from that process? And how we could do that here in the US?
It’s because reverse osmosis still uses so much energy to produce the required pressure and flow rates, and what you mostly get out of it is just table salt.
I ran a reverse osmosis machine up in Alaska, so I know how much energy it takes to produce Potable Water for a town of 1000 people. It does not take that much energy. And that left over “Table Salt” as you call it, has a lot of minerals besides just sodium chloride (NaCl). When They mine Silver/Zink/Lead out of the Lucky Friday Mine in Idaho, gold and other precious metals does not just fall out in your hands, they have to process it through chemical flotation. And the Lucky Friday Mine does not report how much gold and other precious metals they produce, but they do produce enough that it majorly off sets their operating cost (Hence why they have not shut down like the Sunshine mine has.) So the bottom line really is if you can concentrate what your looking for at the high concentration so you can float it out of the other solution, then maybe you can extract it. There is a lake at a really high altitude in Chile with lots of Lithium (Li), the only reason they quit processing it there was because to get at the Li they had to evaporate the water. And getting fuel there, and having your equipment survive the harsh enviroment, and find people that can live in a lower O2 enviroment, was almost impossible. This was already Tried back in the early 1960’s. But we have better Technology that maybe when the price for Li is high enough then they will go back and try it. But again the Ocean has more Li then that lake. The other side of the coin is Israel has come up with a process to ‘mine’ gold out of salt water back in the early 2000’s, they just didn’t blow their horn about it.
The mention of aluminum oxide caught my eye — that’s the ceramet used in spark plug bodies, the sort of porcelain white bit. One wonders if an economical way of retrieving that material from coal ash might also be a matter worth pursuing.
There is so much aluminum oxide ore. It’s called bauxite, usually, and it’s everywhere. Oxygen is the most common element in the earth’s crust, and aluminum is the third most common, so to a rough approximation, the earth’s crust is about 1/2 silicon dioxide, 1/6 aluminum oxide, and everything else fits in the remaining 1/3.
I have to admit, I didn’t know that that’s what bauxite was… or just how abundant aluminum oxide.
In my defense, I’m neither a geologist nor a metallurgist — although clearly I need to look into hiring a *cranial* geologist, as I’ve obviously got a head full of rocks! :P
Apologies.
Isn’t that just one allotrope?
Awesome, but also worrying. It’s like every nation with a dump of coal ash or coal mines can now get their hands on uranium… which is great if it’s used cleanly for power… not so great if used for other reasons.
It takes a lot more refinement to get weapons grade U-235 vs power plant fuel.
Uranium has a way too inefficient critical mass. If you are not a developing nuclear power anymore, the real powerful nations have breeder reactors for plutonium. Miniaturise stuff, there are nuclear artillery shells. And I am not talking armour piercing but real 15kT nukes like the W48 155-millimeter.
The nukes of highly educated and developed people aren’t usually the concern.
Umm… breeder reactors are not weapons production reactors. They typically have fuel cycles longer than 90 days that breed pu240 which taints the plutonium and prevents it from being used in weapons. Weapons production reactors by treaty are specific use. They are optimized for pu239 production.
https://en.wikipedia.org/wiki/Weapons-grade_nuclear_material
239 is made in breeders and not cyclotrons
i’m sure you confused your isotopes there mate
239 is produced in all reactors.
But 240 is also produced, which puts you back at isotope separation.
To make weapons grade plutonium you run fresh Uranium fuel full tilt for a very short time. Which gets you near pure 239 that can just be chemically separated.
Any reactor can make weapons grade Pu, but not while being run in an economic way for power.
Its really easy to obtain uranium. Thats why the monitoring and regulation of nuclear weapon proliferation Focuses on isotopic enrichment and nuclear fuel reprocessing. These things are much
More difficult to do candidly.
Right because the US didn’t have a $1.7 Trillion shit fit over Iraq possibly having yellowcake and doing something with it, from 2003-2011 causing further issues that ain’t all dealt with yet.
While we can all agree radioactive contamination from coal is far worse than even the old nuclear power generation facilities. I think we differ in opinion on estimating the total cost of ownership.
Most modern geopolitical weapons proliferation problems originate from 1950s scientific hubris, and a cavalier policy towards emerging economies. Additionally, even our own disposal sites are constantly leaking radioactive runoff into rivers and coastal areas. Note, no community wants spent fuel and nuclear waste in their backyards, so it just gets shuffled around to various cooling ponds to sit (no one ever admits this issue exists).
Nuclear power plants require Billions in tax subsidies to build, constant armed security, and a massive post-service containment cost lasting 10 thousand years. Additionally, those giant 3” thick stainless steel tanks of Uranium hexafluoride from refinement are not something that will last forever. Likely, the worst waste the “greatest generation” left behind for their kids besides the standard cognitive dissonance.
In summary, I think several Billion dollars of sustainable and renewable distributed-infrastructure is a wiser investment. As this article has proven once again people tend to ignore or forget the lessons of the past, and fails to acknowledge all human-beings are fallible given enough time.
> even our own disposal sites are constantly leaking radioactive runoff
These are not disposal sites, but simply places where the waste is left to wait for disposal which never came.
>it just gets shuffled around to various cooling ponds to sit (no one ever admits this issue exists).
In reality, after it has spent a time in cooling ponds, it is transferred to dry cask storage which is above-ground concrete silos at the nuclear power plant site. This is how most commercial nuclear waste is stored these days.
>Nuclear power plants require Billions in tax subsidies to build
Even if they did, nuclear power makes so much energy that it makes the investment cheap. See e.g Olkiluoto 3 which went three times over-budget and cost 11 billion Euros, and it still makes energy at 42 EUR/MWh which is cheaper than wind power. The major issues regarding modern nuclear power seem to be all connected to buying it from the French – not in the technology itself.
>constant armed security, and a massive post-service containment cost lasting 10 thousand years. (…) tanks of Uranium hexafluoride…
Most of the problems you’re talking about relate to places like Hanford which were nuclear weapons producing operations. That’s about 2/3rds of all nuclear waste in the US. Commercial reactor wastes are not that messy, and the “massive post-service containment” issue is caused by politics: all attempts and even research have been defunded and protested, which is why the spent fuel is sitting in ponds and silos and not re-processed or five miles underground where it’s safe. The nuclear waste problem persists simply because we’re not allowed to do anything about it – by the very same people who protest against it. One necessarily thinks that the point is to keep on protesting, because it’s profitable.
>I think several Billion dollars of sustainable and renewable distributed-infrastructure is a wiser investment
Nuclear subsidies mostly work in the form of loan and insurance guarantees, which are not paid unless the company defaults. Nuclear power is actually taxed on a production basis, so the government eventually makes (and has made) any direct subsidies back – which is how an investment works. There is no “investment” in renewables, because the renewable subsidies are production based – the more they generate, the more you pay. In short, nuclear power works by “subsidize and then tax”, while renewable power is “subsidize and then continue to subsidize”.
This👆!!
Like all AstroTurf campaigns they present straw-man arguments to convince the gullible they addressed the criticisms. The reality is nuclear power is not cheaper, and is a loss-leader sales pitch that costs billions to clean up a location. Note, we actually process your decommissioned nuclear warheads to heat our homes, and know all the dirty secrets you think are hidden away with google maps stitching..
Billions of construction dollars encourages some to gamble with other peoples safety.
The continuously decreasing cost of solar panels means that they will soon completely dominate the energy market and leave all other tech in the dust. We will adapt our strategies for storing and using energy to adapt to crazy cheap solar power.
The nuclear folks touted that their energy would be “too cheap to meter” but solar will actually achieve that goal.
Based on what? Do you even know the unsubsidized cost of solar panels?
Based on nothing.
https://reshapingenergy.files.wordpress.com/2021/02/1608187202503.jpg
New nuclear is about level for cost with utility scale solar power (not counting storage), for the investment payback time. For long term operation (LTO) nuclear is way below anything else on the market. Residential solar and biomass are not cost-effective at all.
The reason why residential and commercial solar do so poorly is because they’re highly distributed, so the labor and material costs per unit are high. No matter how cheap the panels become, it’s never going to get that much cheaper because the panels are already under a third of the full price.
More… This 👆!
When markets are rapidly changing, it’s important to use up-to-date numbers. This is as true in the energy market as the stock market.
The graph you link to is based old data from the EIA. I’m not sure which particular report that is from, as the person didn’t bother to include a reference on it, but it’s definitely the EIA (google the “note” text). I believe it is either the 2018 or 2019 report.
Here is the latest edition of the same report:
https://www.eia.gov/outlooks/aeo/pdf/electricity_generation.pdf
If you look on page 5, you can see that the current average price for new-build PV is 2.5 cents, one half that of the value shown in the link above.
The numbers in the graph came from an older version of the table seen on page 22. As you can see, the EIA’s (always outdated, NREL is quicker) estimates for LCOE on new nuclear are 80/MWh while they put PV at 31 and PV+storage (“hybrid”) at 45.
New nuclear is simply not even remotely competitive.
> Residential solar and biomass are not cost-effective at all.
Utility systems have to compete with other utility systems on the wholesale side of the meter. Residential systems have to compete with LDC pricing. Residential systems in the US currently average about 13 cents/kWh, which may or may not be competitive depending on where you are.
The vast majority of that cost is permitting and inspections, which are soft costs. These costs in the US are twice that of similar permitting in Europe, which suggests there is room for movement here if anyone wants to bother.
I was incorrect, the graph is from the IAE, not the EIA, they just happen to use near-identical language on their charts!
The graph appears to be from data collected in 2015, but I don’t believe it is the 2015 report, still looking.
> Based on what?
Can’t speak for the poster named “X” you replied to, but I will base my numbers on being a PV wholesaler in a previous life.
> Do you even know the unsubsidized cost of solar panels?
Yes.
Currently when purchased in container-sided lots, the price is approximately 18 to 23 cents per watt for “bankable” panels. “Bankable” is a PV term that is effectively “the bank will let you buy it”, which in other industries might call “A-grade” or “OEM”.
In the US, the EIA and NREL track these prices (and the IEA internationally), although their publication times are on the order of a year, so here is the last version of NRELs report from Q4/2021:
https://www.nrel.gov/docs/fy22osti/80694.pdf
Utility-scale inverters are currently running about 15 cents/W. Installation represents the majority of the cost, and soft-costs almost all of the rest (permits, land, fencing, etc.). Currently, all-in costs from paper napkin plan to spinning the meter in the US costs about 83 cents/W (see page v of the report above), and about 5 to 6 cents more for a one-axis tracker, which improves capacity factor (CF) about 15%.
Such a system produces electricity at or under 2 cents/kWh. This makes it the cheapest form of electricity in history:
https://www.carbonbrief.org/solar-is-now-cheapest-electricity-in-history-confirms-iea/
In contrast, new nuclear plants in “the west” are currently averaging between 10 and 12 dollars per W, 12 to 15 times the price of utility-scale PV. This is why no one is building them – the banks simply won’t lend you the money. So things like the UKs Hinkley and Sizewell projects require government intervention, and I think we all share the opinion on how rapidly the government works on large capital projects of this sort.
When faced with this reality (and it’s been this way for years) the pro-nuclear internet know-nothings will invariably start talking about storage. Storage prices are falling much faster than PV prices. In the same NREL report, you can find that PV **with storage** is $1.67/W.
At that point, they will invariably raise the issue of lithium supply. This is always amusing, but especially in an article about extracting uranium from seawater. This is because lithium is 20 times more abundant in seawater than uranium. It also has fully developed commercial processes for extracting it. Current estimates are enough for 20 trillion (yes, *trillion*) Tesla-sided battery packs.
There is some hope for nuclear even in this competitive landscape. The problem with nuclear is not the technology, it’s the construction costs which have basically doubled in the last 15 years. This is not entirely surprising given the large construction market. If you’re a construction company, would you rather build a single reactor which might be cancelled in a five-year project, or a dozen high-rise condos? Don’t answer: the industry already did.
Thus all the hope being pinned on SMRs, which reduce the construction side to something more akin to a high-rise level of infrastructure, while the complex bits are taking place in a dedicated factory. Time will tell what sort of effect this will have, and I think we’ll be seeing FOAK systems in the next two years or so, so we’ll have real data.
It is worth pointing out that there is a well-known formula that suggests single larger plants are more economical than two smaller ones – the factor is about 1.4, so two 600 MW plants will cost about 1.4 times a single 1200 MW plant – which is why in the past all the designs kept scaling up. T
The barrier SMRs have to cross is enormous. A one-axis tracker system has a CF around 25%, and new nuclear around 90%, so on a Wh basis, you need 3.5 times as much PV as nuclear. At the current cost of ~85 cents, that puts us around $4, about 1/3rd the cost of current nuclear. This is going to be difficult! It is no better when you compare to PV+storage, which has a CF around 50%, so you need twice as much PV as nuclear, but that’s even *less* expensive.
Further, as you can see on page vi of the report, those prices continue to decline at ridiculous rates every year. The all-in cost declined 10% **in one year** between 2020 and 2021. So it’s not just that SMRs have to cross a gap, they have to cross a constantly widening gap. This will be difficult (ahem!) and it’s not clear why anyone should bother.
>energy would be “too cheap to meter”
It’s not too uncommon to have fixed utility rates, especially in apartments, because it’s too cheap to meter (meaning, they’d make less if they were charging you for the real amount instead of adding it to the rent).
On the historical point of it, that was just one guy, Lewis Strauss who said it and the press ran with it, while the U.S. Atomic Energy Commission replied that no, it’s not going to get that cheap, and the actual prediction was that it was going to become as cheap as conventional power.
“the actual prediction was that it was going to become as cheap as conventional power.”
That was also a lie. Nuclear will never be as cheap as solar, which is rapidly becoming the new “conventional” source of energy.
You can’t even put a price tag on all the river life that has been obliterated by the excess heat output from nuclear power plants. No amount of new technology is going to overcome the laws of thermodynamics. They try to dissipate the heat with cooling towers but the towers inevitably corrode and collapse, and they just dump the heat in the river instead. Apparently fixing the towers is too expensive…
>That was also a lie. Nuclear will never be as cheap as solar
It’s the other way around: solar is unlikely to become as cheap as nuclear.
Nuclear power LCOE is around 30-40 EUR/MWh with modern plants, even with the massive cost-overruns in building them. Solar may get below that in some places (e.g. around the equator) but that is already forgetting the cost of storage and other infrastructure which tips the whole thing over.
>You can’t even put a price tag on all the river life that has been obliterated by the excess heat output from nuclear power plants.
What about the massive hydroelectric dams built to buffer solar energy? The runoff of toxic chemicals from lithium and cobalt mines? The mountains or nuclear waste produced as mine tailings from rare-earth elements extraction? If you want to talk about minimizing environmental damage, nuclear power still comes out on top.
>They try to dissipate the heat with cooling towers but the towers inevitably corrode and collapse
You just made that up.
> Nuclear power LCOE is around 30-40 EUR/MWh with modern plants, even with the massive cost-overruns in building them
The average cost for new nuclear in Europe averages 10 cents USD/kWh. This is trivially easy to find.
The strike price for Hinkley C was set as 92.50 pounds, which is 12 cents USD.
The strike price for Flamanville 3 is 100 euro, which is currently 10 cents USD.
Olkiluoto 3 has no published strike that I can find, but given it is an EPR and roughly the same cost as Flamanville, we would expect roughly similar prices. Curiously, this will still lead to a *decrease* in Finnish wholesale prices due to the reduction in very expensive imports.
I am very curious where you get 30-40 EUR/MWh? Even the pro-nuclear IAE reports put it between 6 and 7.5 cents, and that’s *including* existing plants.
https://www.iea.org/reports/projected-costs-of-generating-electricity-2020
Technically, you could already make the existing system “too cheap to meter”, simply increase the minimum/base fee while reducing the per kw/h few, after doing this a few times, you push the idea that paying for meters on every customer is raising costs, so, removing the meters would save more than the lost revenue from the per kw/h charge (which has been suppressed). Similarly, if a local government wanted to pay for all the electrical generation and give it to residents for free, that would be possible (they’d still pay for it in taxes).
I doubt it will ever be so cheap that the ones with the means of production give away the product for free.
… until the sun goes down.
Solar doesn’t work at night.
And some people live in places where the winter nights are long, and daylight sun is weak.
Solar panels lose around 15% of their capacity in the first 5 years of use. Another 5% goes away over the next 5 to 10 years. The degradation continues at a much slower pace but never completely stops.
Any new PV installation should be made with at least 20% over-capacity to handle the capacity loss in its first decade.
Used panels that are 5 years old can be a better deal. Though of course they’ll be 5 years behind the bleeding edge of technology, the install will only need to be 5% to 10% oversized based on measuring the used panels’ actual output.
People will have to find someone who actually does windows. if you clean the panels regularly, maybe you might not have such a problem with the 5% loss of efficiency over the next few years. I had a window washer come over and do the plate glass window at my shop and it’s amazing all the detail I was missing and how bright the sunlit room has now become….
> Solar panels lose around 15% of their capacity in the first 5 years of use
I installed a dozen panels on my garage in 2010 and they are currently producing 88% of their original value (well, that was last year).
Current industry-wide averages are 0.5 to 0.8% degradation per year with a first-year drop (LID) as high as 1.5% but averaging less than that.
NREL has been carrying out these tests for decades:
“A review of the existing literature has identified 2,000 uniquely measured degradation rates. Median degradation rates for these modules are 0.5% per year. A long tail of lower-reliability products increases the average to 0.8% per year.”
Sandia got in on the act too:
https://pvpmc.sandia.gov/download/6888/
The problem with solar is it doesn’t produce 24h so you need some storage for night, then the production isn’t stable throughout year especially in higher latitudes so you need even more storage and when your country is flat as pancacke or you just don’t have space for pump hydro storage then you need to use batteries and that ain’t cheap nor long lasting. Nuclear is stable and long lasting. So the best is combine the two they fill in the gaps, and when they both are producing high you can use it for hydrogen generation – it looses energy but if the alternative is just letting it go by turning off then its not a bad choice.
Solar energy will always be dilute and intermittend. Batteries on the scale needed to power a country simply do not exist. Nuclear is the cheapest, safest and most reliable source of power.
Solar power is dirt cheap, as long as you can use it while the sun is shining. If you need power storage, it gets much more expensive
The process of creating electrical energy from nuclear fuel is a horrible hack that always results in massive amounts of extra heat dissipated into the environment.
Compared to the heat from the sun, it’s a negligible amount.
At least if heat is in a concentrated area we can use that energy for other industrial purposes. Pre-heating processes to extract CO2 from the air and making synthetic petroleum, closing the cycle on fossil based energy methods.
Nature did it better already, we need to stop re-inventing the wheel. For mobile transportation petrochemistry is the best method. Now we just need to make the amount we need rather than drilling. Nuclear can desalinate water. Nuclear can provide energy for our homes. Waste heat can be used to remove CO2 from air and get it ready to re-form into a synthetic energy source.
We can close the cycle on energy if we put our minds to it and start learning how to use what nature has made into what we need, without continually damaging our world. But as long as drilling is cheaper we never will. Greed and Profit.
In the USA the single use fuel cycle is mandated by the Nuclear Waste Policy Act. It forbids reprocessing of commercial spent nuclear fuel and requires disposal of it.
Thorium
The yellow cake is a lie.
Yeah, it doesn’t taste like cake at all!
B^P
I think you will find that it was Japanese researchers who first proved that uranium could be extracted from sea water using resins, and it was much earlier than the dates listed in the article. Furthermore in Australia uranium is not necessarily mined using traditional methods either, there is very little on the surface to see outside of the processing facility and some pipes. Even coal is handled in a practical manner here, with my closest power station being located where the coal is extracted so that the ash that is not used for other products can go back where it came from. Then again I guess that is not hard to do when you have a deposit the size of Saudi Arabia that is expected to last for another 300 years based on current extraction rates.
The University of Tulsa and a university in Australia have been working on a similar concept to remove inorganic mercury from water while rejecting other inorganic metals that have a 2+ charge. A recent version of their material is so effective that it removed nearly 100% of the inorganic mercury in the sample within 5 minutes.
https://engineering.utulsa.edu/mercury-polymer/
I wonder if you could adapt this process using another compound than amidoxime so that Gold is recoverable instead. Could lead to a new era of gold mining xD
What the western hemisphere and Europe are going to have to do is develop much cleaner methods of extracting and refining “rare earth” metals that aren’t actually all that rare. It will also have to be cost competitive with the same elements from China, otherwise the manufacturers of batteries and super magnets will just keep buying from the filthy Chinese mines.
Demand for “rare earths” is only going to increase as electric vehicle production increases. Another possible demand may be mass transit with the demonstration of a permanent magnet levitated train in China. By using permanent magnets to levitate the suspended train, it only needs electricity for its linear induction motor. With a clean and low cost method of refining neodymium and other metals, the magnets to line the track should be affordable enough to make the maglev track a heck of a lot less expensive than one lined with electromagnets and their attendant high power supply and control systems. The permanent magnetic levitation just sits there, levitating, essentially forever, with no power input.
“Most of the R&D on this topic appears to occur in China… (due to) its focus on self-reliance.”
Unlike here in the stupidly dependent upon China Western nations.
Why are we still thinking about Uranium and it’s silly process to convert that substance into energy, leaving us with waste products that are to hot and radioactive that we have to safeguard the waste for the coming 100.000 years.
Invest your energy in Thorium and Molten Salt Nuclear Reactors : they don’t create waste-products like weapons-grade plutonium and weapons grade uranium, and other nasty products from the Actinium group.
Thorium reactors even can burn-up these nasty products and convert them into less radio-active products that only have to be supervised or stored away for 30 to 300 years ……
So “burning” radioactive waste AND getting more energy out of it ….
…..and then this scientist that is being quoted in the article is thinking about creating more Uranium waste products ????? Are we serious ???