Doing something for the first time is tough. Yet to replicate the nuclear fusion process that powers the very stars, and do it right here on Earth in a controlled and sustained fashion is decidedly at the top of the list of ‘tough’ first times. What further complicates matters is when in order to even get to this ‘first’ you also add in a massive, international construction project and a heaping of geopolitics, all of which is a far cry from past nuclear fusion experiments.
With the International Thermonuclear Experimental Reactor (ITER) as the most visible part of nuclear fusion research, it is perhaps little wonder that the recent string of delays and budget increases is leading some to proclaim doom and gloom over the entire sector. This ironically in contrast with the recent news from the US’s NIF and its laser-based inertial confinement fusion, which is both state-funded and will never produce commercial power.
In light of this, it feels pertinent to ask the question of whether ITER is the proverbial white elephant, or even the mausoleum of international science that a recent article in Scientific American makes it out to be. Is fusion research truly doomed to peter out amidst the seemingly never-ending work on ITER?
History Sometimes Rhymes
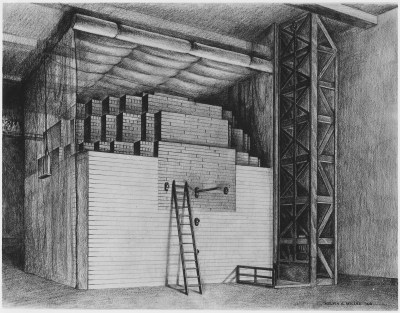
What sometimes trips people up is that although both nuclear fission and nuclear fusion pertain to energy obtained from interactions between atoms, only one of these is exceedingly easy to make happen. All that it takes for nuclear fission to occur is for enough fissile material (such as uranium-235) to exist in the same general location, and enough neutrons to zip about that allow for a nuclear chain reaction to begin and sustain itself. This is why natural nuclear fission reactors existed during the formative years of the Earth, when amounts of fissile material in the Earth’s crust was still relatively high.
It is little wonder then that replicating this process during the 1940s in graphite pile reactors was a relatively straightforward process: the graphite moderates the emitted neutrons, which are thus slowed down into thermal neutrons which cause more fission events, which produce more neutrons, which are moderated, etc. Although this obviously took some engineering to get all the details right, the first artificial nuclear fission reactor became a fact on December 2nd, 1942 when Chicago Pile-1 (CP1) sustained a nuclear chain reaction.
Although CP1 had no cooling, shielding or other amenities, CP2 and derived reactors were already usable as crude predecessors to the commercial power reactors that would form the backbone of the nuclear fleet build-outs in countries like Canada, the US, France and Japan during the 1970s, most of which reactors are still operating and producing power today. With this success in mind, one might be forgiven for being optimistic about the chances of also tackling nuclear fusion.
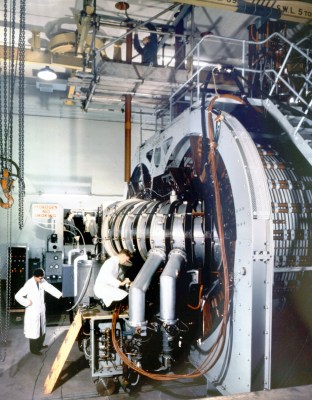
During the 1950s, the UK had become one of the leading forces behind nuclear fusion plants, with the research up till that point indicating that the Z-pinch (zeta pinch) method of containing deuterium-tritium plasma within a magnetic field was all that would be needed to create a commercial fusion power plant. Unfortunately, it was discovered that in the larger prototype Z-pinch reactors the plasma was not as well-behaved as originally assumed. Instead plasma instabilities would occur seemingly randomly within the reactor, which led to the near-demise of fusion research, until the invention of the tokamak breathed new life into the field.
These first tokamak designs with their donut-shaped field proved to be significantly more capable of dealing with the whims of the highly dynamic and very hot plasma as it churned within the confinement of the field lines. Ultimately, the demise of commercial Z-pinch-based fusion reactors proved to be not the end of the dream of commercial fusion, but rather the beginning of a much better understanding of plasma physics.
Many Eggs, Many Baskets
Of the things which have changed significantly over the past seventy-odd years, advances in computer technologies and materials science have allowed us to create reactor vessels and magnets that would have been deemed near-magical in those early days. In addition, using supercomputers we can simulate plasma fields in great detail, simulations which are continuously improved by experimental data obtained from the many active fusion research reactors around the world.
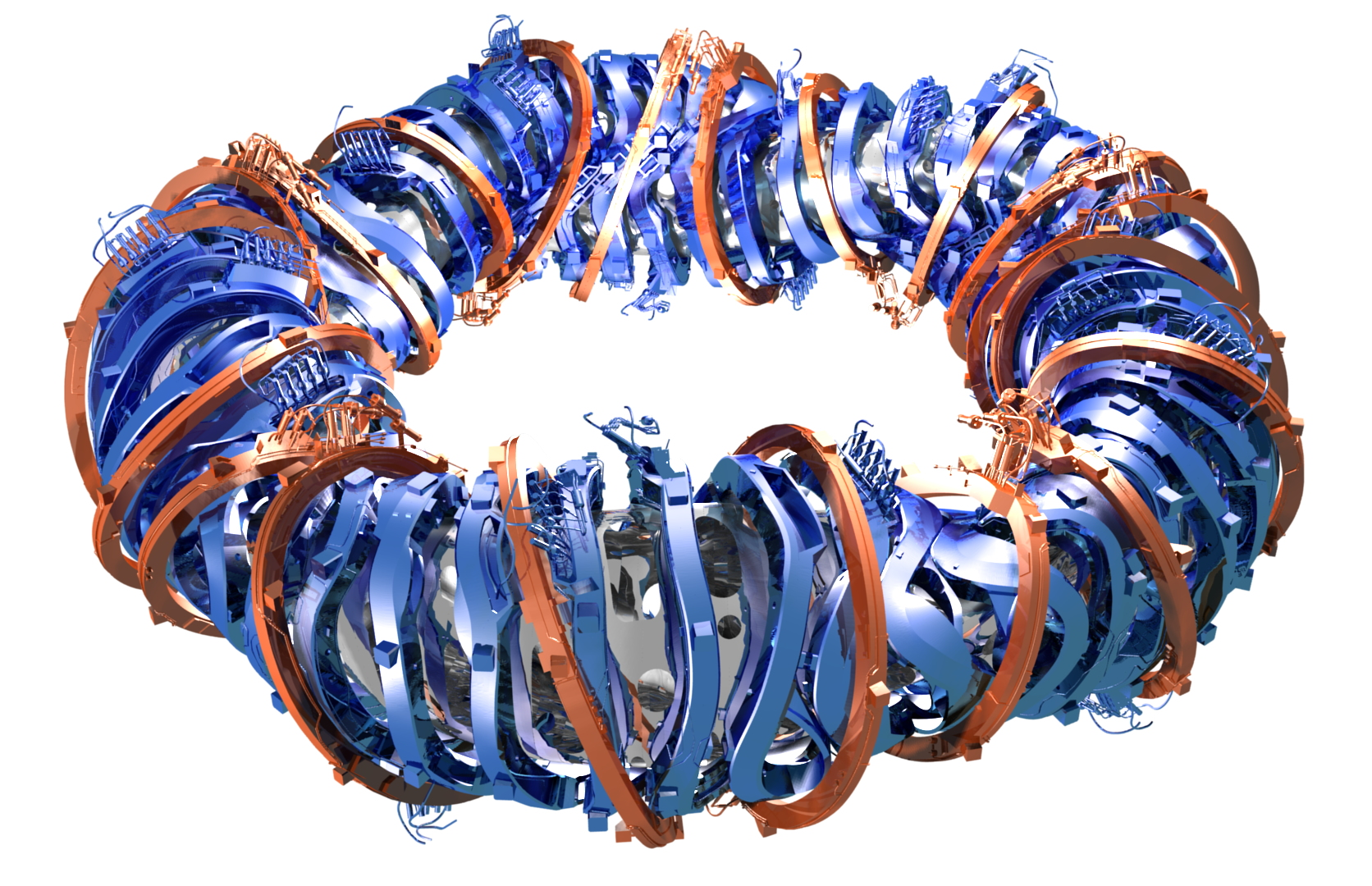
Perhaps most fascinating of these reactors are the stellerators, which are not a new concept, but which require a level of understanding of plasma field dynamics that was beyond the grasp of our understanding and simulation tools until a few decades ago. Since then, this type of fusion reactor has rapidly caught up on tokamak designs, with the Wendelstein 7-X being the most well-known. Using its oddly shaped electromagnets, it creates a magnetic field that doesn’t just trap and constrain the plasma as in a tokamak, but instead seeks to ‘flow’ with the plasma. Theoretically this will increase efficiency, and allow for continuous operation to demonstrate the feasibility of stellerators for use in commercial power plants. This particular stellerator has most recently been updated to its final configuration with cooled diverters, which should allow it to demonstrate a continuous run with hot plasma for up to half an hour.
Meanwhile, a mixture of both state-financed and private fusion reactors are vying for the spotlight, with the UK’s JET tokamak and ST40 spherical tokamak getting headlines, and the private Commonwealth company putting decades of US national laboratory fusion research to good use in its innovative ARC and SPARC reactor designs that employ high-temperature, superconducting electromagnets. Of the remaining research reactors, the Chinese fusion program is probably the most ambitious, using their medium-size HL-2M tokamak in particular to not only provide experimental validation for ITER’s design, but also for the China Fusion Engineering Test Reactor (CFETR).
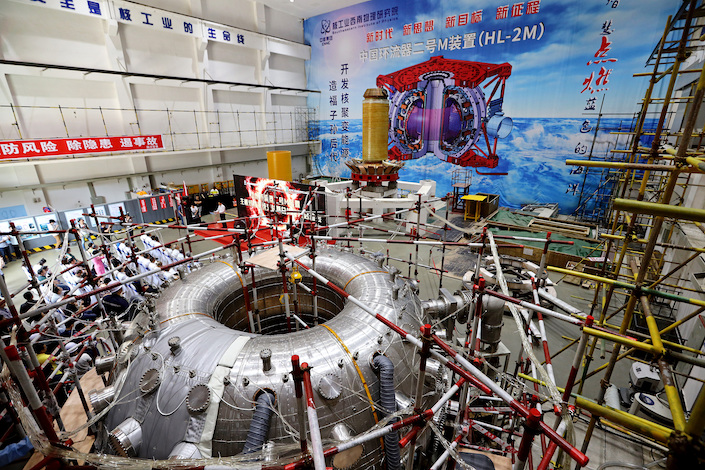
Whereas the HL-2M tokamak has a major radius of a mere 1.78 m, the CFETR as it is currently scheduled to be constructed this decade will feature a major radius of 7.2 m, which would make it larger than ITER at 6.2 m. Essentially, CFETR will fall between ITER and its intended DEMO successor, allowing it to validate DEMO principles, such as breeding tritium fuel from a lithium blanket inside the reactor vessel to sustain continuous operation.
Interestingly enough, according to the current schedule, the CFETR should begin operation around the same time that ITER is projected to begin fusion with deuterium-tritium fuel, around 2035. Despite the experimental nature of CFETR, China is looking at using the approximately 2 GW of thermal energy for electricity production, with molten salt as buffer. As these early fusion reactors are likely to need frequent breaks to cool down and for maintenance, having an intermediate buffer might already make fusion reactors viable power plants by next century.
Engineering Chops
As an international undertaking, ITER relies on the nations involved in its construction to provide the components for the tokamak, which appears to cause issues that mirror the troubles that plagued Boeing’s 787 airliner, with many subcontractors around the world being provided with incomplete specifications, along with quality assurance troubles. Whether it concerns an airplane or fusion reactor, smaller issues can quickly snowball into worse issues, especially when they’re not tackled early on. Add to this the massively disrupted supply chains in 2020 and beyond due to the pandemic, and it would have been more amazing if things had continued without delay.
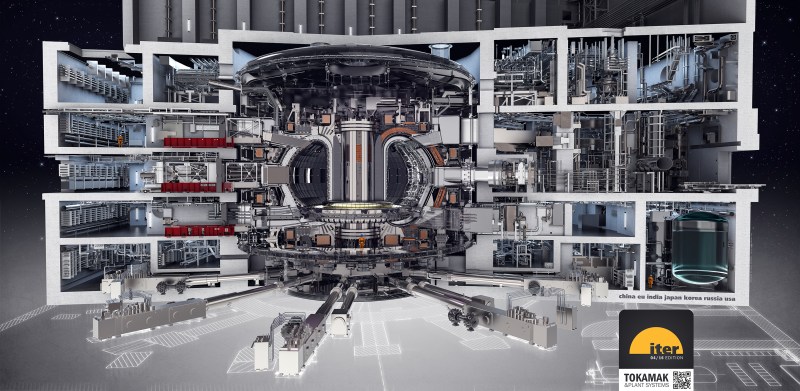
Although the experimental validation for aspects of ITER’s design continues using existing tokamaks, the actual assembly of the physical reactor has to be performed by skilled workers, who are provided with quality components, materials, and tooling. Reportedly, this is where ITER does have some major issues, with defective components arriving at the build site, and some workers claiming qualifications that they do not have. These are unfortunate project management aspects that are not exclusive to the construction of fusion plants.
In essence, then, it could be said that the ITER project has major issues that need to be addressed. Yet to mark ITER as somehow a monument to the folly of pursuing nuclear fusion power, or the final resting place of international scientific cooperation would be an offensively poor hot take on the state of the field. After all, the collective fields of plasma physics, fusion research and adjacent are cooperating every day for many decades now towards the singular goal of achieving sustainable nuclear fusion.
To then characterize ITER – with its roots in 1970s geopolitics – as somehow the end-all, be-all of fusion research is nothing if not dishonest. Whether or not ITER is ultimately finished next century and starts operating is more a question of sunk cost, but it is far from being humanity’s sole hope of unlocking fusion power, let alone the linchpin for international cooperation on the topic. Ideally the current issues with ITER will be resolved sooner rather than later, but it should not reflect on the field as a whole.
After all, ITER is just part of the puzzle, with private companies around the world investing in their own designs, multiple designs by nations like China with a strong nuclear industry courtesy of its ongoing fission plant build-out, and even Germany with its so far incredibly successful Wendelstein 7-X stellerator. All of whom are whittling down the last remaining issues towards making commercial fusion plants a reality.
(Heading image: Aerial view of the ITER site in 2020. (Credit: Macskelek) )
How much energy is lost due to bremsstrahlung, or braking radiation, as the charged particles rotate. I would imagine that it is a lot.
I’m guessing mostly hard X-Rays and Gamma rays. I wonder how much shielding is required.
This article completely overlooks many promising commercial ventures which are neither tokamaks or stellarators, such as Helion and Zap Energy.
I found the Helion videos (YouTube / RealEngineering?) highly compelling, and apparently Microsoft agrees, as it’s planning to buy at least one of the reactors. I am also confused as to why these “alternative” ventures are rarely mentioned in many of these kinds of articles – not just HAD.
And the Lockheed High Beta reactor.
Most of these startups are only a traps for greedy investors. Their plans are as realistic as tokamak plans for fusion 50 years ago. They might succeed, but not with reactor which fits on truck (Lockheed, etc) and not in 10 years.
Even if the reactors don’t work out, the IP and patent development possibilities still exist. Commercial reactors could be close enough to construction that patents now might still be valid.
This is pedantic, but that’d be synchrotron radiation, not bremsstrahlung. Bremsstrahlung occurs when charged particles interact with material, or each other (“beamsstrahlung”).
Agreed! It looks like @Truth is trying to pull a whataboutism for obvious reasons to negate the possibility of fusion becoming real
Actually, I think it is a mixture of both synchroton and bremsstrahlung. Accounts for about 6% of the losses in a D-T Tokamak. The charged particles in the bulk plasma are interacting with each other all the time. It’s basically a Maxwellian system.
Not much, perhaps 10%. We will have to add impurities to increase radiative looses at the edge. Plan is to radiate up to 95% of power. This will reduce particle heat load in the most exposed regions of the tokamak divertor.
What they are trying to do in these facilities is not duplicating “the process that powers the very stars.” That reaction is the proton cycle, and while it is aneutronic which is good it also requires ridiculously high unattainable temperatures and pressures and doesn’t yield very much energy. Stars aren’t hot because they are like hydrogen bombs, they’re hot because they’re so big it takes millennia for the energy created to get out of the core, so it all piles up.
What every single one of these reactors are trying to duplicate is the reaction that does power the second stage of a hydrogen bomb, but slower and less violently. And that reaction generates more neutrons than you can even imagine. For reference, most of the energy from a hydrogen bomb doesn’t come from the fusion reaction. It comes from the fast neutrons from the fusion reaction slamming into depleted (normally non-fissile) Uranium and making it fission. That’s where 80% of the energy comes from. Neutrons are not a nitpicky side issue, they’re the main way energy exits the reactor. (If you don’t use the neutrons from the D-T fusion reaction to induce fission, for example by using an inert tamper, you get a device called a neutron bomb. It kills people very effectively despite not being as boomy.)
That kind of neutron flux quickly changes non-radioactive elements into dangerously radioactive isotopes not found in nature, knocks atoms out of their positions in crystal lattices which can cause metal fatigue and distortion, and a number of other unhealthy phenomena. When you bring this up about fusion power people like to mutter that there are aneutronic reactions, but most of these still produce enough neutrons to be dangerous (especially if you’re talking about power reactor levels of activity) and while they’re not as unattainable as the proton cycle they are all a lot harder to sustain than D-T fusion, which we can still barely demonstrate.
If we ever manage to sustain D-T fusion the next technical challenge will be to find a target for the neutrons that a) can get hot so we can use it to make elecricity, b) doesn’t fail mechanically due to the neutron flux knocking its atoms out of position, and c) doesn’t turn into dangerous radioactive waste. I am not aware of anybody who is doing any serious work on that necessary next step, because it’s a complete waste of time until we can sustain D-T fusion, but it’s at least as big a problem.
“I am not aware of anybody who is doing any serious work on that necessary next step”
What you’re looking for is the design and development of thermal blanket modules and lithium breeding blankets. In the ITER program those are called TBMs (test blanket modules).
Because, as you note, no one’s actually *done* this yet (because… we don’t have DT fusion), there are several different variants currently being studied and tested. I’m not exactly sure what you mean by “doing serious work” – most of the designs are either at the preliminary design review or are building scaled mockups.
It’s also worth noting that the world’s supply of tritium’s small enough that it makes sense that you only test at one reactor in a coordinated program, even if you don’t plan on using it for power. It’s been noted elsewhere that the tritium output of D-T reactors might actually be more valuable than the electricity itself.
“I am not aware of anybody who is doing any serious work on that necessary next step”
I guess doing exactly this kind of research ist the purpose of IFMIF : https://en.wikipedia.org/wiki/International_Fusion_Materials_Irradiation_Facility
But indeed it is a overlooked issue, and tbh this project is probably totally underfunded.
“Stars aren’t hot because they are like hydrogen bombs, they’re hot because they’re so big it takes millennia for the energy created to get out of the core, so it all piles up.”
Wot, I think you went too far in the comparison and somehow lost the reason you started that sentence
Exactly
https://thebulletin.org/2017/04/fusion-reactors-not-what-theyre-cracked-up-to-be/
D-T fusion is not at all ‘clean’, even if it ever does become practical and economical, which is unlikely.
“Scientific American” – the word Scientific no longer has a place in the name of that politicized rag of a publication.
Agreed. I stopped reading it shortly after they canned the “Amateur Scientist” column. The rest consisted of too little hard science info and way too many (woke) opinion pieces. At this point, I wouldn’t use their pages to paper the bottom of a bird cage.
@Observer ++, yeah – it’s amazing how far “Scientific American” has fallen into woke-land :-(
Try this instead for now (until the Marxists catch up with them and cancel), pretty good stuff:
https://en.wikipedia.org/wiki/Quanta_Magazine
Ha wait until you see the universities… We aren’t getting fusion anytime soon. We broke something back in the 1970s
Try Science Magazine Published Weekly by The American Association for the Advancement of Science (AAAS) https://www.science.org/
More science than this human can absorb, and only one of their journals.
It’s not free of political bias, but then no science is. You need to learn to separate wheat from chaff to properly nourish your mind.
I’m not a paid shill LOL
I’ve recently shredded some pop-science books which I got from my parents in 2001. Hydrogen and electric cars were just around the corner, Internet was supposed to make us all happier and more educated, fusion power plants were to replace coal and nuclear reactors. It all aged like milk.
Forget which book but it was about N rays and other radiation schennanigans. It said energy density of the sun is roughly equivalent to a box turtle or pile of compost. If anyone here can explain to me how, under the enormous pressure and temperature of the sun that is the energy density (I believe this part- the sun is incomprehensibly huge) how do any physics support productive fusion on earth without the reactor being, like, the size of the entire earth or something.
Because the Sun’s making power from pure hydrogen, which is a fundamentally terrible way to make energy from fusion. Why? Because there are no heavier nuclei that are stable without neutrons, and pure hydrogen doesn’t have any. So the first thing you have to do is make neutrons – and that requires going through the weak force, which is *terrible*. It’s called “the weak nuclear force” for a reason.
And when I say it’s terrible, I mean *terrible*. Like, 99.999%+ of the time of the full reaction in the Sun is spent waiting for two protons to magically get in the right configuration to flip into a deuteron. And I think I’m missing about 4-5 nines up there.
But we don’t have to use pure hydrogen: we’ve got *plenty* of neutrons available, and so we can creatively choose the fuel to allow producing energy much, much more efficiently.
Basically, if the Sun wasn’t made out of almost pure hydrogen, it’d be generating a *lot* more energy.
This is very helpful thank you
” the Sun is spent waiting for two protons to magically get in the right configuration to flip into a deuteron. ” LOL, must not be to magical. Its been doing that for millions of years, and still going.
Magic’s easy when you get to try so many times it’s got a 1 with 50+ zeros after it.
If your fusion device has lots of neutrons, please don’t build it anywhere around me.
They have a terrible habit of wandering off and causing trouble.
If your fusion device *doesn’t* have a lot of neutrons… it’s a big ball of hydrogen, and we call it a star.
Even ‘aneutronic’ fusion reactors would still *use* neutrons. They just keep them confined in the nuclei.
The problem with ITER is time. Taking nearly three decades to build a technology is a perfect recipe for having new technologies rendering your plan obsolete. A monumental waste of money and resources that could have been put to better use with rapid iteration!
Right? That’s like saying “We are putting a human on Mars in 2040”. So what are you doing until then? Perhaps launching a rocket every year to collect valuable data about what tech is actually needed would be a good idea.
Magnet and superconductor science are both radically different than they were 30 years ago. They are building a Tesla on a model T Ford chassis.
What have they been doing?
A ton of the research that also led to the more efficient designs.
The main research that ITER’s trying to do is stuff that every other design needs anyway. If you read the papers on the stellarator, or CFETR, or almost anything else, they reference ITER studies repeatedly.
It’s a research facility, not a commercial reactor.
>The main research that ITER’s trying to do is stuff that every other design needs anyway.
If that is really so, they’re holding everyone else back. In reality, nobody is waiting for ITER – they’re running circles around it testing the very technologies that ITER was supposed to trial.
2023-25 is “assembly and itegration planned”. You can’t do much research with a machine that isn’t even built yet.
“You can’t do much research with a machine that isn’t even built yet.”
You apparently really, really don’t know how research works, then. *Most* of the engineering research on a project occurs before it’s built.
Look, there will never be a practical fusion reactor anyway. This is not the point of all the fusion research. The point is all the side effects, all the collateral technologies that are being developed in process, and ITER got a stellar track record of producing them.
There’s an excellent series of videos on fusion power by ImprobableMatter on Youtube (https://www.youtube.com/playlist?list=PLbhKQRV6Toq4ocE3C1EwVbeY4ofwrPLn_)
The last one in the series on reactor practicalities really lays it down as to what the challenges are to make a reactor that generates viable quantities of power reliably.
Also one of his videos, on Helion Energy’s efforts (https://youtu.be/3vUPhsFoniw), is a good 7 minutes of press-release bubble bursting.
Have you checked out Helion? They are taking a very different approach tor eco eribg the energy using the magnetic resistance instead of heating steam to turn a turbine generator.
My current favorite contender, just because the approach sounds so bonkers:
https://firstlightfusion.com/technology/our-approach
Rather than using lasers to concentrate energy on the fuel pellet, they’re just gonna use a big fucking gun.
Rad.
Humanity has proved itself more than capable of building big guns. So this sounds reasonable!
How American! And it certainly works for investors. Unfortunately this hammering approach was tried in many different version and it is not so easy. What happens when you hit a drop of water by a hammer? It will just splash. In order to compress it, you must squeeze it uniformly from all directions. And this is what makes it so difficult. Scientists are using lasers and golden hohlraum in NIF project for very uniform irradiation of the DT target and it works. Hitting the fuel by a bullet is just like hitting it by a hammer. The velocity of 60km/s is impressive (if they will ever achieve it), but it is negligible compared to thermal velocity of the fusion plasma, so the plasma will just “splash” (Rayleigh Taylor instability to be accurate), like a water.
I am of the opinion that research needs to revisit the older pre-tokomak linear designs. The things they thought would be easy in tokomaks turned out not to be, but all the control systems and mega magnets they’ve invented for the tokomaks, have I think inch by incremental inch, turned out into greater advancements than that which they thought were necessary to make the linear designs work. Aside from the specific technologies, there’s a bunch of other stuff that’s just “not a problem” any more, computing power, not a problem etc. Synergistically, we might be at a point where we could apply a bunch of basically “off the shelf” techs and solutions and fix the perceived “huge problems” of earlier designs.
Nope, the problems of the privious design were very fundamental. There are some attempts, like this Lockheed project, but they are doomed to failure
@localroger
Yes, in stars, the unbelievably humongous size is part of the mechanism. For one, because of the gravitational containment, for the other, because the reaction taking place has a ridiculously small cross section.
The best reminder of that is that the power generation density at the Sun’s core is roughly that of a compost heap [1], below 300 Watt /m^3.
[1] https://en.wikipedia.org/wiki/Sun#Structure_and_fusion
The heck with Dyson spheres then, we just need to build a really really big compost heap …
Think we’re in the process of doing that…
@Drone, @Observer et al
Could you please take your identity politics elsewhere? This is about fusion, plasma physics and stuff.
I think 4chan is over there.
Thanks.
I don’t think you know what “identity politics” means. Their views are most likely the exact opposite of that. That said I agree we shouldn’t unnecessarily politicize everything. Which includes this discusion, but also “Scientific American”
All this money and talent spent of a pie-in-the-sky plan for controllable fusion. Gosh, we have an almost endless supply of geothermal energy that remains mainly untouched. Geothermal energy also has its R&D and implementation costs, but the energy exists.
Agreed. 99% of most scientists are basically smart little kids. It isn’t fun being practical. It’s fun trying something new before we know what would already work.
We know the costs for extracting the geothermal energy pretty well. And except for a few regions on world, like Iceland, it is not profitable.
Ah yes, geothermal energy on our space stations, geothermal energy on our space ships. Geothermal energy on large ocean and air going vessels. All that geothermal energy on the moon and mars.
We need to be able to build a fusion reactor, so that we can improve it and make it smaller. There are _so_ many applications for fusion.
When I was in graduate school in the late 1970s working on RF heating of plasmas, I asked at a meeting whether anyone there could with a straight face say that any of the major devices could lead to a machine which could be run by approximately the same number and type of people who ran a fission power plant. Take a look at the ITER picture. QED. At last count, there were something like 35 different groups working on alternatives. Fingers crossed that one of them will pan out, but not holding my breath.