If the current Administration of the United States has their way, humans will return to the surface of the Moon far sooner than many had expected. But even if NASA can’t meet the aggressive timeline they’ve been given by the White House, it seems inevitable that there will be fresh boot prints on the lunar surface within the coming decades. Between commercial operators and international competition, we’re seeing the dawn of a New Space Race, with the ultimate goal being the long-term habitation of our nearest celestial neighbor.
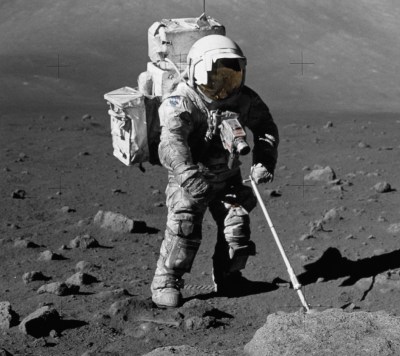
But even with modern technology, it won’t be easy, and it certainly won’t be cheap. While commercial companies such as SpaceX have significantly reduced the cost of delivering payloads to the Moon, we’ll still need every advantage to ensure the economical viability of a lunar outpost. One approach is in situ resource utilization, where instead of transporting everything from Earth, locally sourced materials are used wherever possible. This technique would not only be useful on the Moon, but many believe it will be absolutely necessary if we’re to have any chance of sending a human mission to Mars.
One of the most interesting applications of this concept is the creation of a building material from the lunar regolith. Roughly analogous to soil here on Earth, regolith is a powdery substance made up of grains of rock and micrometeoroid fragments, and contains silicon, calcium, and iron. Mixed with water, or in some proposals sulfur, it’s believed the resulting concrete-like material could be used in much the same way it is here on Earth. Building dwellings in-place with this “lunarcrete” would be faster, cheaper, and easier than building a comparable structure on Earth and transporting it to the lunar surface.
Now, thanks to recent research performed aboard the International Space Station, we have a much better idea of what to expect when those first batches of locally-sourced concrete are mixed up on the Moon or Mars. Of course, like most things related to spaceflight, the reality has proved to be a bit more complex than expected.
The Gravity of the Situation
That you could mix lunar regolith up into a substance that would harden like concrete was never really in question. Chemically it all checks out, and there were even some very promising experiments done with samples returned from the Apollo missions. But while scientists could expose the resulting mixture to a vacuum easily enough, there was no way to study how it would cure in low-gravity environments.
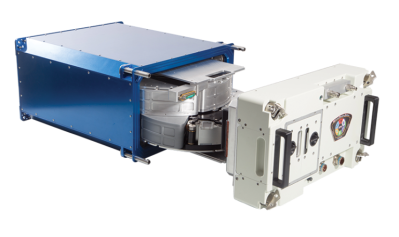
Short of going to the Moon or Mars and trying it in field, the only way to see how extraterrestrial concrete would behave on these worlds is by simulating their gravity in space. Here on the surface of the Earth, there’s no way to lower the force of gravity, but in space you have the luxury of starting from what’s effectively zero. Aboard an orbiting spacecraft, a sample can be placed inside of a centrifuge and spun up to simulate the roughly 17% of Earth’s gravity it would be subjected to on the Moon, or 38% in the case of Mars.
Which is exactly what astronauts aboard the ISS did during the Microgravity Investigation of Cement Solidification (MICS) experiment. Pouches filled with a hydrated cement mixture were placed in the Multi-use Variable-g Platform (MPV), a commercially owned and operated centrifuge that can be run from 0.1 to 2G. Samples were spun up to expose them to artificial gravity equal to what they would experience on the surface of the Moon and Mars, while control samples were left to cure in the normal microgravity environment aboard the ISS.
The Science of Concrete
The impact of varying gravitational strength on curing concrete might not be immediately obvious. Here on Earth the process seems so simple: just mix it up with some water, let it sit out, and in 24 hours or so you have a solid block. To understand the situation better, we first need to look at what concrete actually is, and what’s happening at the microscopic level as it cures.
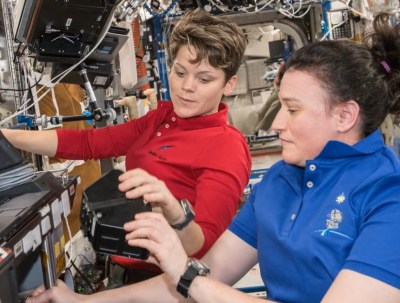
Firstly, while there’s a tendency to use the terms interchangeably in casual conversation, concrete and cement are not the same thing. Concrete is actually the end result of mixing an aggregate (sand, gravel, etc) with cement, which acts as a binder to hold it all together. Put another way, you can have cement without concrete, but you can’t have concrete without cement. Research performed during the MICS experiment has therefore focused on cement, as that’s the first step towards producing concrete strong enough to actually build a structure.
While curing, two different processes are occurring inside the concrete. The aggregate, being heavier than the cement, has a tendency to slowly sink towards the bottom. At the same time, the cement is crystalizing which “glues” the aggregate in place. Striking a balance between aggregate sedimentation and cement crystallization is key to producing a strong final product, but as both processes are heavily dependent on gravity, the strength of concrete cured in low or zero G has always been a topic of debate.
Early Results
Researchers from Pennsylvania State University and NASA’s Marshall Space Flight Center are still analyzing the returned cement samples, but so far they’ve already made some very interesting observations. Further experiments will almost certainly be required before any kind of definitive statement can be made, but clearly we’ve got a lot to learn before the first concrete structures can be poured on the surface of the Moon.
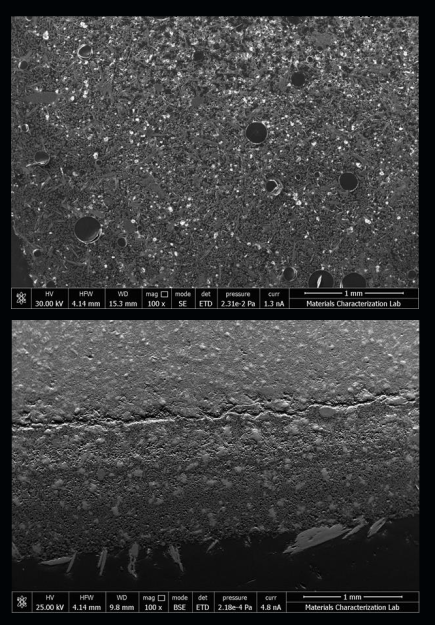
The good news is that lower gravity leads to reduced sedimentation. Without Earth’s gravity to pull the heavier material down to the bottom of the mixture, the cured concrete should be of a more uniform density and therefore stronger. It also means that the ratio of aggregate to cement can potentially be increased, resulting in a denser concrete than would normally be possible on Earth.
Unfortunately, the samples also show that the crystalline structure of the space-cured cement is more porous than its terrestrial counterpart. The hardened cement contains voids which are likely due to the fact that air bubbles in the mixture are less likely to rise to the surface under reduced gravity. Future experiments will likely focus on ways to reduce the number of air bubbles that remain in the cement as it cures, potentially via mechanical vibration or exposure to a low-level vacuum. While it might seem that exposing the cement to the total vacuum of space would solve the issue, previous experiments have shown that this causes the water in the mixture to sublimate; with the water drawn out, the curing process stalls and the cement never hardens.
Put simply, the structure of space concrete is nearly the opposite of traditional concrete. On Earth, gravity causes aggregates to sink to the bottom while air bubbles rise to the top. But in reduced gravity, there’s a tendency for everything to stay in suspension. When cured, this results in a material that’s more uniform but considerably less dense than it is on Earth. How this will affect the final strength of the concrete and its usability as a building material off-world is yet to be seen. Until then, affordable housing will remain one of the many challenges of living on the Moon.
“the cement is crystalizing which “glues” the aggregate in place” – “but as both processes are heavily dependent on gravity”
Just wondering why cement crystallisation would depend on gravity at all?
In high school I saw an experiment to make a crystal from copper sulphate. any knock to the jar would slow the process. I assume gravity slows the brownian motion within. It’s curious that the problem is similar to how we make bread rise. Maybe electrolysis could be used to draw the bubbles away while the cement sets.
Isn’t vacuum enough to remove the bubbles? Same process as vacuum degasing during silicone/polymer casting. If there only was a cheap way to obtain vacuum in the outer space.
Well to quote from the article ” previous experiments have shown that this causes the water in the mixture to sublimate; with the water drawn out, the curing process stalls and the cement never hardens.” So it’s sorta too much of a good thing.
So obviously it is not a good idea to expose the mixture to vacuum for the full curing time. But it is easy imaginable, to expose it for some time to get the bubbles out (vacuum degassing) and repressurize it then. You could even add some extra water at the beginning to compensate for some loss during the degassing process.
But then that madee question: justchownsre they going to NOT expose it to vacuum?! Unless you are making brixks, large structures to serve as dwellings will naturally be built OUTSIDE existing structures.
Vacuum only increases the size of the bubbles and therefore their boyancy: They rise therefore to the surface where they pop. Unfortunately, even large bubbles in zero or low gravity dont rise at all or very slowly…
Maybe mix the concrete in the vacuum of space. Or expose the curing concrete to the vacuum or part thereof
Close, but Not quite. As the article says, full vacuum messes with the water, which is required to cure.
How about mixing at partial vacuum, but just high enough to avoid the issues mentioned?
Then increase to normal pressure, or even higher once it’s mixed, for the duration of the cure.
Any contained air bubbles should be from the near vacuum, and will be squashed significantly by the increased pressure.
I think some industrial processes do something similar on Earth, where they use very high pressure to squeeze any bubbles that were from atmospheric pressure.
The biggest problem is the equipment on the ISS probably isn’t designed to do that.
I suppose you’re trying to be pedantic because the actual process of crystalization doesn’t require gravity, but clearly the resulting crystals are different in it’s absence (or reduction).
Well, you could always put your cement sample in a jar and throw it off a tall tower to get the cement to set in microgravity. You’d just need some VERY fast setting cement.
Or a very huge tower :P
You would probably need a space elevator to get to the top :-D
https://www.zarm.uni-bremen.de/en/drop-tower/general-information.html
Build a structure of metal that will be able to create its own gravity. Then you can produce concrete blocks on a large scale, and then assemble them. The assembling of the blocks is much easier than what it takes to make them.
You mean like the high G-force training simulators that are used to train astronauts and fighter pilots.
Send machines to direct sunlight using a large number of mirrors to melt the lunar regolith into either strips, sheets or even interlocking dovetail blocks.
The moon is roughly the same distance from the sun as earth (~150 million kilometres), so the energy received per square meter would almost be identical to the upper atmosphere (1,413 to 1,321 watts per square meter), which is about 30% more than the peak at ground level on earth (ozone blocks 100% of UV-C, mostly absorbs UV-B and oxygen strongly absorbs UV-V – all of which is good for life on earth, but bad for harvesting energy). We have wind and a much stronger gravity on earths surface so a much larger (~30% bigger) and more rigid structures would be required to test on earth but it is something that could be done prior to testing on the moon.
Like this: https://hackaday.com/2011/06/25/selective-solar-sintering-with-sand/ ?
I just fear the result would be to brittle and fragile to be used for larger structure.
That is melting desert sand which is mostly (~47%) quartz (silicon dioxide). You are forming a glass, and by heating it at one point only and allowing it to cool rapidly you are creating internal stresses which makes glass brittle (it could always be annealed relieve internal stresses later on).
Anyhow with lunar regolith the bulk (99%) of the material is Oxygen, Silicon, Iron, Calcium, Aluminum, Magnesium, Titanium
And the remaining 1% is mostly Manganese, Sodium, Potassium, Phosphorous.
With enough energy the oxygen would be released and the material produced may still be glass like but with high enough temperatures there is a high enough metal content that with slow controlled cooling and the help of gravity it could separate into layers probably the bulk of the iron and silicon would form a ferrosilicon compound and sink to the bottom with the other metals in one or more alloys floating in layers above.
Melting points and boiling points below are at 1 atmosphere, ~1 bar.
Oxygen (~42% by weight), atomic weight 15.999, melting point 54.36 K, boiling point 90.188 K
Magnesium (~6% by weight), atomic weight 24.304, melting point 923 K, boiling point 1363 K
Aluminum (~7%by weight ), atomic weight 26.981, melting point 933.47 K, boiling point 2743 K
Silicon (~21% by weight), atomic weight 28.084, melting point 1687 K, boiling point 3538 K
Calcium (~8% by weight), atomic weight 40.078, melting point 1115 K, boiling point 1757 K
Titanium (~2% by weight) atomic weight 47.867, melting point 1941 K, boiling point 3560 K
Iron (~13% by weight), atomic weight 55.845, melting point 1811 K, boiling point 3134 K
There may also the possibility of distilling Magnesium or Calcium from the mixture, since their melting and boiling points would be lower in the near vacuum of the moons surface.
I don’t have melting and boiling points for the moons surface pressure of 2 x 10^-12 torr (3 x 10^-15 bar)
All I have is a datapoint for the vapor pressure (boiling point) at 10^-4 Torr (ref: search for “Critical Melting Points and Reference Data for Vacuum Heat Treating” )
Magnesium boils at ~604 K
Calcium boils at ~736 K
Aluminum boils at ~1081 K
Silicon boils at ~1389 K
Iron boils at ~1468 K
Titanium boils at ~1523 K
All I’m saying is that there may be a few interesting lines of research.
The material is not a mixture of these elements, but a mixture of compounds of these elements. Oxides, silicates, titanates, etc. By heating it you probably could sinter it together to a more or less crude ceramic. But you not just distill off it’s components. Metallurgy and metal refining is not that easy. Although the processes are understood on earth for decades or even centuries.
Yes I agree, but at a much lower vapor pressure (like on the surface of the moon) all the intermolecular bonds in the compounds may break apart with less energy. While the material is in a molten state, MOE (Molten oxide electrolysis), could possibly be used to to liberate the oxygen is another possibility. And what is left behind may then be easier to process into more useful materials.
It all comes down to the level of energy supplied and in what from. With a little energy you can end up without a ceramic being produced.
near vacuum pressure on the moon should help mitigate the cooling problem, as there’s no atmospheric convection.
Crystallization does not depend on gravity, but the crystals do form differently making this is part of why zero gravity can be useful and has had a lot of study.
Why would you try to create Earth designed concrete in space? Am I the only one not shocked by the results? The philosophy of designing for the environment was proven well back in the Apollo era. (The lunar lander’s landing gear could not support the craft on Earth, because it was only designed for the moon’s gravity.) Why would “space concrete” be expected to be created using Earth-bound methods?
No one is shocked at the results. These experiments are to see how our current understanding needs to be modified for the different conditions. They had to start somewhere, and starting with what we know how to do is often best.
I think for many applications the increased porosity could be accepted, as the weight is lower. Perhaps it could be even an advantage, if you need heat insulation.
Sorry if I’m misreading your tone as “Stupid post-Apollo NASA wasting money testing something we already know, again!” but I think you’re not giving these tests credit where credit is due.
For the Apollo landing gear, all the mechanical engineers knew “gravity will be ~0.165G” so they could take that information and run back to their blueprints, get out their textbooks and sliderules, and see “the stress on this member in 0.165G is going to be… and the strain on that in 0.165G will be…”. Perfect. Design for environmental constraints achieved.
So why aren’t the dummies at NASA doing the same for concrete now? It should be simple. Need to tweak your concrete mix to cure in a lower-pressure environment? Talk to some civil engineers in Denver and ask what they recommend. Need to cure at low temperature? Ask some Scandanavian/Russian/etc. civil engineering firms. Need to handle salt water? Ask someone with experience in dockwork and port building. Need to know about high temp? Ask some geothermal guys whats up. Worried about ionizing radiation? There’s probably a national lab or two with some experts who can give you advice on that. Need to know how to tweak your concrete mix for zero G? Just ask… umm… well… shoot.
The problem is, that although we’ve been mixing concrete for millennia, and tweaking the ratios and processing to suit the applications as we go, we just have near-zero experience on how gravity skews the results and therefore can’t just take out our textbooks and sliderules to adjust the ratios and design optimal “space concrete” given the known low-G environmental constraint. These NASA tests aren’t just “testing whether ‘Earth concrete’ works in space”… they’re determining the effect of varying the G parameter between 0-1 when making concrete, now making NASA the first and only expert to call when you need to tweak your concrete mix for a low-G environment.
One advantage of conctrete is that it doesn’t require much energy for the amount ot material you get. Energy is required for the cement but for the aggregate, you just have to move it around (and sort for size).
Using energy to melt and fuse the regolith into blocks would be wasteful. What would make more sense would be to use the energy to melt the regolith and spin it into fibers. Basalt fiber is used here on earth instead of carbon fibre for some applications. These fibers could be fused into rods (my hypothesis when investigating lunar concrete (on paper) decades ago) then used as reinforcing rods in the concrete.
My investigation years ago was about using lunar regolith in zero g to make structures orbiting the moon. I think the general conclusion that most came to was that I was crazy… but I get that a lot.
I’ve read a basalt fiber abstract. Fascinating stuff.
Concrete on Earth requires steel rebar reinforcement to achieve lateral strength. Will we be shipping steel or iron to the Moon, mining iron asteroids, or finding other ways of providing strength, assuming it is needed?
We would look to the Romans for that, they didn’t use rebar, and their structures last millennia. Partly, they designed in such a way so that lateral strength was much less necessary. Think arches and columns, where the whole thing is under mostly compressive loads. Also their mixes were a bit different, and more labour intensive in the pouring stages, as a tradeoff for increased strength.
It seems like this would be a much better use case for something like rammed earth or compresses earth blocks rather than poured concrete. To begin with it seems more reasonable because the amount of water needed for rammed earth is a fraction of that needed for poured concrete. The structure is formed through compaction and is immediately structural, though it increases in strength as it cures/dries. We have done structures with as little as 5 percent cement, which at that point is primarily for erosion control not structural strength. With a combination of strong compaction force and vibration it should be possible to create blocks ready to use directly from a press. Especially in a low gravity environment. The compaction effort should eliminate the voids.
That might work, but only if you can seal each block against the water sublimating out.
This is interesting. Down yet another rabbit hole for the evening, I suspect.
I think there was research into alternate building materials. Does anyone know what every became of the self-sintering ceramic bricks? I seem to remember reading about those as building material a while back.
Obviously, mix it without voides to begin with.
I think we should abandon concrete and dig some holes. Make everything underground and seal it airtight with some spray-on truck bed-liner stuff. The ground will reinforce it and the bed-liner is very strong against scratches and impacts and air/water proof. Seal airlock doors to the ground from below using the same stuff and some dirt piled around the edges to help hold it down.
Many of the spray on sealants would require atmosphere to cure and likely wouldn’t handle the temperature swings on the moon. Many polyurethanes need moisture from the air to cure. Even if you mix in water it will evaporate off due to the lack of atmospheric pressure. Most curing processes are very sensitive to temperature and pressure in one way or another. I’m not saying it can’t work, there might be a suitable product out there. But regular bed liner is unlikely to be suitable.
Good point. I didn’t intend to say it had to be bed-liner. I meant more of the notion of a spray-on sealant, and bed-liner provides an apt example for clarifying my point due to its properties. I’ve read of ideas involving a cement sealant made of moon materials. That would be nice, but I don’t know any of the details.
The thing is you have to lug that spray on liner stuff from earth to the moon. The theory here is that we can get most of the stuff we need for concrete from the moons surface (possibly even some of the water) without having to lug it all the way out of earths gravity well and then down into the moons gravity well.
That’s a fair point. I believe the original argument was against heavy things like enough concrete to build a building, so this would be much lighter since you would have the moon as a structure, and you’d only need to spray it with the small amount of spray you brought from home. I’m attempting to find middle ground between great idea and doable as an actual moon base. The natural protection from micrometeors and radiation alone makes me think underground is best however you decide to go about it.
I have always found it interesting that using slide rules and computers no smarter than our wrist watches, we were able to put men on the moon in less than ten years time back in the 60’s. Now, even having the data and results from previous moon launches, and with today’s technology that makes the 60’s look like the stone age, we are unable to put a man on the moon? Yeah… seems legit.
When Rich (of the Rich Rebuilds channel on YouTube) was buying a used Tesla Model X, and it was taking forever to get the car, Rich’s mother had something to say about how Elon Musk could put a car into space buy couldn’t put Rich in a car.
In part you are ignoring the increased funding NASA received back then.
The shear drive and manpower that went into the space race was just incredible. People were not just working on it for a pay cheque. It was war effort level drive involved. That combined with higher risk tolerance and mountains of cash made it happen.
In many ways technology has made us lazy and mentally weak. Where we once had to do all the math in our head or on paper, we now depend on computer to do it for us. This has lead to very few people actually understanding things at a deep level. We have also largely stepped away from manufacturing. In the 50’s and 60’s many more people in the US were directly involved with manufacturing high quality things. Today those skills have been largely lost to China.
The 60’s were far from the stone age. The big difference between then and now is just mass production of electronics. There haven’t been many big science breakthroughs since. The science, math and engineering they used back then is the same stuff we use today. It’s just computers have gotten cheaper and much more complex thanks to mass production.
Clow57 has and idea I was thinking about and have watched a little regarding lately again since seemed like was popular when I was younger in certain places. Great way to make use of local cost effective resources and Matt Resinger has a good video regarding an design using rammed earth.
I was wondering about the segregation of the mixture as is an issue underwater when pouring and trying to reduce surface area in contact with the water since will cause desegregation of the cement mixture.
Interesting reading to see the desegregation isn’t an issue apparently. Wondering about the method optimization to prevent cavitation or whatever is causing the air bubbles?
“Processing of the MICS samples include removing a sample from the kit and squeezing the burst pouch to rupture the burst seal ejecting the water solution into the “cement” powder compartment. After the water solution is injected into the cement compartment, the crew “mixes” the constituents using their hands in kneading fashion as well as using a provided spatula type mixing aid in order to create a paste like consistency of the cement mixture. After about 90 minutes of mixing the crew applies a “clip and seal clip” to contain the paste in a single compartment of the MICS sample for a prescribed amount of time before application of isopropyl alcohol (IPA), from a third compartment, is completed to arrest cement hydration.”
I’m not reading where the centrifuge was used in detail. Are there curves or something still being calculated to observe relationships to properties at different gravity and whatever other variable like time or something else?
Air pressure isn’t digital, though; you don’t need to restrict the process to either hard vacuum or Earth normal. The air pressure could be lowered until it is just above the water sublimation point and thereby reduce air contamination without water loss.
Does that make sense, or am I missing something?
Concrete need water, send water from earth to moon !!!! I’m shocked :-O
Wouldn’t it be pretty easy to construct a centrifuge that subjects the concrete to earth-like gravity, on the moon? Like, a bar, a bearing and a small motor, more or less? Obviously more robust and since it’s mission critical it needs to be overengineered a bit, but it’s a solution that doesn’t weigh much, require a lot of energy and can be packed very small.
The concrete doesn’t need to be in there until it sets, just long enough that most of the airbubbles can get vibrated out, and it can be set down in the 0.17g on the moon to set.
Trapped air in the cement? The mix comes from the moon sans air. Hydrate the evacuated mix and then stir and mold at pressure. Unless you lunar mix is generating some gas when moistened.
I see that rammed earth was mentioned… Another option might be expanding foam. This could be applied either on its own or in combination with surface materials.
Wasn’t this issue solved by earthbag construction in like the 80scool experiment but why does itt feel like we’re repeating third grade for the second time?
Using some form of a surfactant would reduce the surface tension of any liquid used thereby making the resulting ‘concrete’ harder. It is done on Earth.
With the Apollo missions we landed, took some pictures, picked up some rocks, then went home. Nasa intends that this time we’ll be going there to stay.
“current Administration”
Is there any reason you won’t say “Trump”? Calling out the name of the president didn’t seem to be an issue when Obama was president.