The 700-horsepower Porsche 911 GT2 RS is already pretty darn fast — over three times faster than the average regular-person car on the road today. For the sports car enthusiast, there’s likely no ceiling on the need for speed and performance. And so, Porsche was able to wrangle another thirty horsepower out of their limited-run supercar by printing a set of ultra-lightweight pistons.
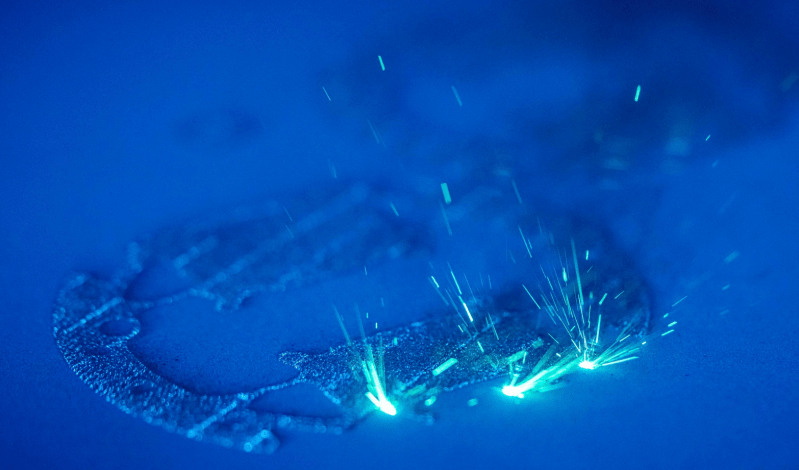
These pistons are printed from high-purity aluminium alloy powder that was developed by German auto parts manufacturer Mahle. Porsche is having these produced by Mahle in partnership with industrial machine maker Trumpf using the laser metal fusion (LMF) process. It’s a lot like selective laser sintering (SLS), but with metal powder instead of plastic.
The machine dusts the print bed with a layer of powder, and then a laser melts the powder according to the CAD file, hardening it into shape. This process repeats one layer at a time, and supports are zapped together wherever necessary. When the print job is finished, the pistons are machined into their shiny final form and thoroughly tested, just like their cast metal cousins have been for decades.
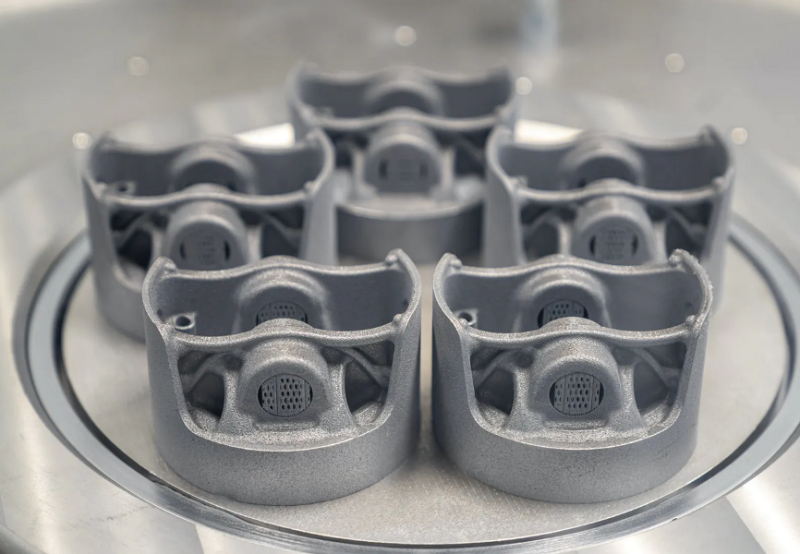
Leaner and Meaner
Generally speaking, prototyping car parts with a printer is much faster than traditional methods. There are no molds to be made, which cuts down on both time and expense. These pistons don’t just have a cool origin story — they have advantages over cast pistons that make them objectively better.
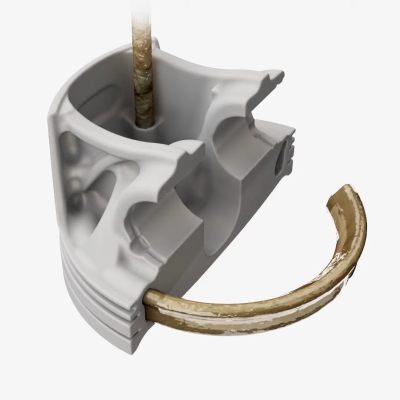
For one thing, additive manufacturing allows for designs that aren’t possible with casting. All of the fat has been trimmed from these pistons — in this design, there is only material where forces will act upon the piston, so it ends up weighing 10% less than regular pistons.
This lean design leaves plenty of room for a built-in cooling system, where oil shoots up through the bottom of the piston head and circulates through the areas that get the hottest. When you have lighter, cooler pistons, the engine can work harder and run faster.
This isn’t Porsche’s first foray into printed parts. Custom bucket seats are an option for two current models, and they also offer certain printed aftermarket replacement parts here and there for models that are no longer in production.
Porsche aren’t using these powerful pistons in production cars just yet. The laser metal fusion process is much better suited for small-scale production, limited run parts, and prototyping, at least for now. Printing a bed of five pistons takes twelve hours, according to this video about the printing process.
High-Stress Print Jobs
What could possibly go wrong with a printed version of something that’s designed to move so quickly under pressure? Probably nothing, though there hasn’t been time for long-term observation. Mahle says the printed pistons are extremely strong, and that they undergo the same rigorous testing as forged pistons. This includes a pulsation test to make sure it won’t crack under stress, and a tear-off test of the area where the piston rod connects. Then they put the pistons through a 200-hour stress exercise on a 911 GT2 RS test engine.
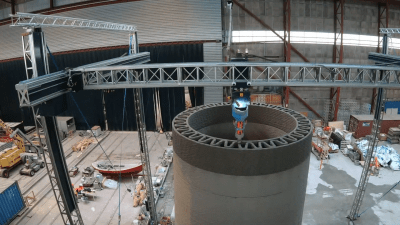
3D printing has been pushing the limits in other industries, too. You’ve no doubt heard of entire houses being printed in a matter of days. Concrete printers are also helping wind turbines to reach new heights by allowing gigantic bases to be printed on-site. The taller a wind turbine is, the better, but the added height necessitates a thicker base. In the US, the base size is limited by, of all things, the height of highway overpasses.
And as far as additive manufacturing for ad hoc replacement parts goes, it doesn’t get much more high-stress than a nuclear reactor. Even so, there’s a project underway at Oak Ridge National Laboratory to create a reactor with as many printed parts as possible. And printing replacement parts for out-of-production reactors is already happening. A few years ago, Siemens switched out a faulty impeller with a printed version, and Westinghouse printed a thimble for holding active fuel rods.
Would you drive a car with printed pistons? You probably won’t be printing those on your own at home anytime soon, but you could already be making replacement badges for your project car, or given enough time, piecing together a full-size plastic Lamborghini.
I don’t think people completely understand that these are not only extremely expensive printers but they also utilize very pure environments to print from that require a host of PPE to use in addition to needing to be machined afterwards. If people are expecting in a year or two to be able to pick up one of these machines for a few hundred bucks, that’s just not realistic. Not only that but there are limited abilities to dramatically increase how quickly they print as well. Don’t get me wrong, they are fantastic for limited environment applications and they can allow you to make a huge amount of well designed but otherwise impossible to machine items but production applications for larger volume items like this are not likely to turn into mass production items and I unfortunately don’t see an easy way for this to become quick and cheap in the short term either. More of another tool in the toolbox than a completely different way of doing most things.
Noted, will save a few hundred and fiddy bucks to make sure.
The machines are currently expensive, but the cost per part they produce and the materials required to run them doesn’t seem particularly outrageous. Infact I’d already call it cheap compared to machinists time.
So I can see a future where they come down in price rapidly because they are just so damn useful. Will it replace other methods – no more than FDM printers have replaced injection moulds. But it should come down pretty quickly as nothing about its workings or material requirements is that exotic and then you will start to see smaller batch mass produced parts and parts that are too complex to make other ways efficiently using the method because it works out cheaper (though that last one will be partly the other way around, because the process has become cheaper designers won’t worry about designing a part to work best with other manufacturing techniques as much).
I mean how much did the earliest 3d printers cost, its a tech concept that go back a very long way and none of the varieties start out as cheap, but if they work and do things other established techniques can’t manage or can’t manage as cheaply doesn’t take long for the machine to become more common and cheap. I think your right it won’t drop that fast, but like FDM printers the couple hundred price point for a functional machine might not be that far away- and the few thousand affordable enough to become more widespread is probably very very soon..
Your right nothing comes off a printer (or out of a mould) and is ready to use. Everything has post processing of some sort, even the basic bulk injection moulded plastic parts will go through some sort of finishing steps even if its just tumbling around a bit to knock the potentially sharp flashing off. So its worth pointing out this isn’t pure magic – but its also nothing special in that regard.
On the speed front – well how quick do you need, if you get a useful part 90% of the way finished with no man hours invested it works out better on the wallet than employing the skilled machinists, or even the more low skilled labour the hours it would take them to get that far even if it takes ten times as long. Might not be as quick, maybe even a slightly worse product than purely machined from raw stock, but people cost a fair bit even at sweatshop levels of pay…
I agree. I came here to make a similar point.
There is always a complexity of parts, quantity and cost trade off to pick the most appropriate production method. I see additive manufacturing to be similar in many ways to CNC machining of parts, you are batch processing and both require experienced operators and consumables.
In this case I suspect Porsche will start offering it for the low volume top spec engines.
I do think that additive manufacturing will become much cheaper and change the cost, complexity and quantity calculations.
I was part of an EU project & one of the partners (HAGE3D) was working on a filament 3D printer system capable of printing large polymer/ metal powder parts that can be processed (removal of polymers) using metal injection moulding type processes to make sintered parts.
https://hage3d.com/index.php/en/metal-ceramics/
No person hours needed but these LMF machines are *not* fast, which severely limits output volume, even if you set aside their 6 figure and up price tags. Also we are talking about very pure, gas tight containers in which they work. The raw material costs are not absurd but not cheap either and the gas and lasers and so on are not able to get but so cheap. It can all drop a fair bit but my point is that this technology has fundamental limits on just how cheap it can drop to is all, even once patents expire. Plus the raw materials needed are nowhere near as user friendly. Maybe there are other 3d printing methods available or coming soon that could make metal parts less expensive but this LMF process still only very slowly gets you a blank part with metal support materials that need to be machined afterwards to result in a finished and usable part. It’s not as if you can just snap off some FDM parts and call it good.
Again, it is very useful technology but it has it’s place and that place is not currently for anything but very limited production volumes of specialty products. That will eventually change of course but don’t expect this to replace significantly faster and far less expensive metal production techniques anytime soon for bulk or even medium sized product runs.
I agree with you on most of your points, but these powder-bed printers (in all their variety) do not need support. The unfused powder supplies perfect support.
I have next to me a 3D printed V6 engine model made about 20 years ago using a printer that used corn-starch as the powder and an inkjet head to print a binder. That doesn’t make functional (or even edible) parts though.
Metal printers like these require some support structures built in, depending on the geometry. Also corn starch isn’t anywhere near as heavy as metal powders. Powder acts like a support material but there are still some limits if you want to ensure the end result geometry if you are making larger parts. You also most definitely have to machine them afterwards if you want accurate parts as well, these are limited in both accuracy as well as surface finish. They get you close at least but that’s just step 1 towards a usable part. You also have to physically remove the part from the base once printed which can be done manually but still needs to be machined just to start with. There is going to be machining involved, the idea is that it gets you closer to what you need and for parts such as titanium or superalloys, you don’t need to start with a huge blank and machine (throw) 70% of the material away. It allows you to rapidly iterate arbitrary geometries. It is not fast per part. It is not inexpensive per part. That’s just not where these specific types of printers excel.
These and other related metal powder based 3D printing processes allow you to make better geometry than can be machined, parts that can be “rapidly” iterated compared to traditional processes as well as less expensive parts (for some use models). They serve a niche for applications that can benefit the most from things where the cost is not the core factor but performance is (F1, aerospace, etc) or where time to production counts the most while the material must be a high performance metal.
Having done some research (YouTube) I see that you are correct, it seems that SLS and SLM metal parts are printed fastened to the bed and with extensive support. I can only assume that this is because the layers tend to curl up while thin due to thermal stresses.
https://youtu.be/00yub5L4I18
This is not a problem with the powder/binder processes.
SLM process requires supports at any overhanging feature, the “A fresh plate of pistons” picture even shows support material in the wrist pin holes, and the ‘printed’ pistons also have a somewhat rough appearance and have no piston ring grooves. So, yes, the parts not only need support, but will require machining and polishing. SLS on the other hand, does not require additional supports.
“Infact I’d already call it cheap compared to machinists time.”
I beg to differ. These take lots of time to be printed and then still need to be machined afterwards. Right now with mill/turn centers with automation a single center could knock out a bunch of ready-to-go pistons long before a machine like this could print them.
I was meaning the process in general – but there is no way a machinist could make some of these pistons details in one part, you’d still want a casting first before handing it to the machinist, which means another skilled workman.
To machine such complex geometries just as a machinist would take I would think at least 4 parts, that would then need brazeing, welding or high temp interference fitting to make the finished part. That is a huge amount of work. And man hours – making a lawn-mower piston yeah knock those out by dozen or more in the same time but this has real engineering inside making this method I would suggest very very cheap for this part.
looking at the conformal oil cooling in that piston (4th pic from the top), I’d say – no.
The only way you can make this using traditional methods is casting with a core. A very thin and long core, that can’t be held in place with much material and it’s dab-smack in the middle of the casting. QC and the post processing on this would be a small nightmare.
While 3D printing nowadays suffers from hipsters trying to use for everything, even on things that subtractive machining can do orders of magnitude better while being faster and cheaper, the pistons in the article are the perfect usecase. Most projects involving complex internal passages are, especially for low-volume production or one-offs.
Such cooling galleries are entirely standard in conventionally-cast diesel engine pistons. Made with a salt core, as described elsewhere in this thread.
Agreed, these are made on a very high-end SLM machine, and the cost of one piston is more than most hobby-grade 3D printers. While it’s pretty much impossible for this tech to become as accessible as desktop FDM printing, there are developments in binder-jet and bound deposition metal printing which are pushing costs into the 10’s of thousands range, where it is feasible that someone would be able to print and sinter a part in their garage (although even then it would still be less common than people with big VMCs in their garages).
Agree with most of your points. I remember seeing occasional videos on SLA/resin printers in the 1990’s on TV shows like Beyond 2000 and Movie Magic, and it took until the past few years before the tech became truly accessible. And the requirements for post-processing amount to rinsing parts off in IPA, not carefully extracting parts from super fine powered metal or other materials that you really, really don’t want to be breathing.
On an industrial level though, I can definitely see this process being used more widely over time. When you take into account the time and skill to work out and program tool paths, the machine setup, the raw material waste and the cost of tooling with finite lifespans, SLS has some major advantages compared to traditional CNC machining. We’re still talking multiple decades, if not longer, but I can definitely see this transitioning from a niche manufacturing technique to more mainstream… again, in select areas and in industrial settings.
For home use, yea, I just don’t see it anytime soon.
3D printing isn’t a prerequisite for internal cooling passages, those have been standard on diesel engines for some time now. They are made by a really interesting process (which is why I am bothering to mention it). Instead of traditional sand casting cores, they use cores made of salt. Then when the casting has hardened they can wash the cores out with hot water.
Actually making salt in to cores is not trivial, it is quite a complex formulation of salts to achieve a strong enough core which still dissolves readily.
I have seen 3D-printed cylinder heads where the internal water passages are filled with a complex web of fins and struts, to increase strength and heat transfer.
My employer has a Renishaw aluminium printer, and we quite happily print functional suspension parts for test cars. The materials properties are quite as good as a casting.
Fascinating, I had never heard of salt cores, makes alot of sense though – got to be much easier to get out than sand, and removable from shapes you could never blast the sand out – just let it dissolve…
Damn now I want to try it for no other reason than I know its possible, don’t even have a project that could benefit in my head, and that’s not happened in ages (Who here doesn’t have cool idea lists they would like to get done, and lists of things they are supposed to be doing for the friends/family several miles long all waiting to make it to the in progress list (and maybe/hopefully eventually to leave that list as finished))..
On further thinking I assume you have to be careful what salts you use on the chemical reactiveness front too.. No point casting something to have the insides eaten away by reactions between the salts/metal and water…
I’ll do more reading before I ever try it (if I do) but I assume this method will work for most metals as long as you don’t have salts in the mix trying to breakdown and release gasses at much less than the pouring temp and that are not massively reactive to the metal you are using (a little reactiveness won’t hurt much as the salts will be flushed rapidly).
Seems like a much harder method to master than sand cores – which doesn’t seem trivial to master either…
I would guess that the salt does not have time to react with metal so corrosion will be slight and can be cleaned up with an acid wash later.
I suspect there are still geometries additive manufacturing can handle that this technique would struggle with. But perfection is the enemy of good enough and there are probably other production considerations.
I think the coolest casting method I’ve seen uses disappearing styrofoam.
Much like a lost-wax casting they start with a full-sized model of a part – say an engine block – made of expanded polystyrene. This model can be made of multiple pieces glued together so it can have really weird internal cavities. The foam is then dipped into a ceramic slurry which is allowed to harden and then molten aluminum is poured into the mold. The styrofoam inside just kind of… vanishes.
Granted, you can’t get the kind of complex internal passages you’re describing for salt cores, with lost foam you’re still limited to geometries where you can mechanically clean off the ceramic mold material, but still, it’s just immensely cool to see the foam just go poof !
I’m kind of amazed that all that carbon inside the mold doesn’t adversely affect the cast metal, but hey, I guess that’s all part of the art form.
Yep- I cast iron occasionally at Carry Furnace in Pittsburgh, and I learned that technique from the casting crew there.
You can cut styrofoam quickly with hot wire or cnc, flame smooth, and then build a one piece sand mold around the object- and molten iron just evaporates the stuff.
You can do some pretty complex stuff this way without making multi-core molds, or even having no parting lines- and it works. I’m actually going to do my next casting session this way for some custom mechanical furniture pieces.
It’s not as much detail as lost wax casting, which can be done with iron, but wax needs to be baked out of the mold overnight- white styrofoam doesn’t. Granted, you gotta be ok with having a mottled finish, like the surface of styrofoam, but it makes a lot of complex stuff easier.
Yeah that technique I knew about, its neat of course – superb for quick and dirty build and test type cycles. But I don’t know of anybody making good quality finishes out of it. Where packed sand or investment lost wax style castings are really tidy end results.
There is no reason you could not combine the two-
Do styrofoam to fill 99% of the mass, then glaze it with wax- I bet that if you just skim coated the styrofoam with just enough wax to give a smooth, hard surface, you could just burn it all out together, and get the benefit of both.
Never seen or heard of anyone doing that- but don’t see why it wouldn’t work.
yeah I could see that working, though getting the wax skim to actually be the right thickness to make the part you wanted could be tricky. I’ve never seen anybody burn out wax with the pour intentionally either. Might be even in a thin layer there will be too much mass of wax to vaporise effectively leaving waxy mess on the surface of the part..
Thanks for that info. I was totally not aware of techniques like that!
There is lots of info on that, you just have to know what to search for :)
https://www.bdguss.de/fileadmin/content_bdguss/BDG-Service/Infothek/Sonderpublikationen/Int._Druckgusstag/13._Druckgusstag/LostCore_Sindelfingen.pdf
https://www.ceramtec.com/files/press_mention_2016-09-01_en.pdf
It’s my understanding that metal 3d printing is effectively a sintering operation. I’m really impressed that a sintered part is outperforming a forged one. I guess geometry outweighed metallurgy here?
It’s probably something to do with parts that remain hotter longer end up with larger grains, even if the forging process aligns them favorably, larger grains are weaker. Whereas a powder that already has fine grains, spot heated to fuse together, may cool quickly and not develop larger grains.
There can be a serious problem with residual stresses as the material is heated locally so you get big heat gradients. Often parts need to be annealed to relieve the stress. You can have some control of texture so can control the direction the grains grow which can lead to improved properties.
https://hal.archives-ouvertes.fr/hal-02064113/document
Maybe next they can make a super light hand crank for a Model T Ford while they are duplicating obsolete junk
We don’t need a crank, we’ve already got one. :)
+1
As cranky as you sound, seems like you could be the stand-in.
So you are enjoying those exhaust fumes?
Irrelevant comment.
To be fair, I can see a market for 3D printed parts for obsolete classic cars, where cost is rarely an object.
I know Jay Leno has got parts for some of his more obscure cars 3D printed.
Even when cost is an object. A friend recently had a brass part investment cast from a CAD model. (The advance-retard lever detente quadrant from a 1908 Singer pickup truck). Ordered online by submitting an STL. They printed in wax, investment cast, and sent him the part. All-in cost was £60.
Some processes are much cheaper than others. SLS steel is much cheaper than SLS aluminium. I got a quote for an SLS inlet manifold from Shapeways and it was £1400. The same part in SLS (then bronze fill) steel would have been £300.
It’s interesting to see new tech applied to what is, in effect, a relic. Pistons: very quaint.
+1
You can joke all you like, but guess where the primary R&D money for those printers come?
Yeah, automotive industry actually has aerospace and airplane industry beaten last time I checked.
Things are only a relic when it hasn’t been refined and improved for so long that it’s essentially forgotten.
Is it cold up there, the early peak of the D-K curve? What you may not realize is that lighter, tougher pistons could make internal combustion hydrogen fed powerplants more efficient than fuel-cell electrical. That’s because the upper theoretical limit on hydrogen efficiency in an IC engine is 100% … best we can get at the moment is about 50%… you know why that is? Daft question. Flame front speed.. for hydrogen it’s damn fast, we can’t make the pistons move quick enough, inertia is part of the problem, and strength, they fly apart. So to capture all of the energy from the hydrogen burn, we need really high RPM engines, which piston tech like this may enable…. Should hydrogen infrastructure ever become a thing of course.
Dibs on the Miata, since I’m not the kind who prefers a self-driving appliance over a car.
Gimme AV to tow a fun car to the track and get me home when I blow the engine seeing how hard I can push :)
Engineering Explained has a pretty good video explaining why the ICE will be around for a while longer.
A properly maintained ICE vehicle should not have the issues you mention.
That’s one of the tradeoffs of an ICV vs an EV. The ICV needs constant, but relativity inexpensive maintenance while EVs need very little maintenance but the bill is pretty big if it’s part of the power-train.
The Voltec power-train really isn’t simpler than that of an ICE car because of the 3 separate sources of torque plus the regenerative braking system. It is a decent plugin hybrid car but not perfect. (BTW, checkout some of he tear down videos of the transaxle, it’s pretty interesting tech in there).
For EVs, electric motor tech is fairly mature. The next set of innovations will probably be around making induction motors behave more like permanent magnet motors (something Tesla has already started doing with the model 3). That reduces the demand for certain rare earth minerals that are mined in Africa in pretty irresponsible ways.
Control electronics are a big part of that and sensors will be important for that too.
There might be some materials innovations int he future that shave some weight off the motors as well.
Batteries are going about as fast as they can at the moment. Solid state batteries are the most exciting thing that appears to be manufacturable, but unless that’s what Tesla is announcing on Sep 22, we are still probably at least a few years away (then a few more years for manufacturing capacity to ramp up). Other battery techs require things like graphene or carbon nanotubes to be manufacturable in quantity and qualities that are currently not possible.
As for using extra battery capacity, I see the promise of it being used in a number of ways. Increased range or reduced vehicle weights are the two obvious ways. A third is to use the capacity to run a motor that uses electromagnets instead of permanent magnets.
Future driver assist technology based on deep learning is likely to consume a fair amount of power too. There are the additional sensors to run as well as the raw computing power needed. (Consider Nvidia’s AGX Pegasus runs around 500W).
The extra capacity may be needed as a charging buffer for fast charging tech as well.
So we will need to see what tradeoff the car companies make and what the market responds to.
In the Model 3 Tesla does not “making induction motors behave more like permanent magnet motors”. They just use rare earth (NdFeB) magnets in the motor. Although the 4WD models have an induction motor as second one.
Let’s just forget about the stream of deadly gases coming out of the tailpipe.
To be clear, I am not advocating for the ICE to remain in use for general transportation indefinitely.
Would you happen to have a reference with the specific emissions product and the amount of emissions allowable to meet various emissions standards?
I was trying to find the details for California as those will be generally targeted by manufacturers as it’s easier to have a single version of an engine for the vehicle to manufacture.
Regulations on the fuel will also impact those emissions which is why California also has regulations on things like sulfur allowed in fuel.
Resources form Europe would probably be useful too.
If we assume that cars meet these standards (cheaters are caught and punished), then I’d like to see just how ‘deadly’ a properly engineered and functioning ICE vehicle is. This should be considered for various timescales as an informed way to help create EV policy. This is something I’ll bet a number of government agencies have done (though some are pressured by science denying politicians).
Again, I am not advocating for the ICE to remain in use for general transpiration indefinitely. But the hyperbole does not help EVs gain ground.
Also of note, a person placed in a sealed space will eventually die from the deadly emissions they create too.
… Pens Panos.
I read the many of the comments above and see a lot of folks that have misconceptions about how supposedly easy this process is. I happened to watch a YouTube video by Dan Gelbart yesterday regarding this subject and there’s a lot of things required beyond simple printing of a part that are required to complete a part made from this process. Check the video out at
https://www.youtube.com/watch?v=nyYcomX7Lus.
Dan has a series of videos regarding fabrication that are well worth the watch if you build almost anything. I highly recommend grabbing your favorite beverage and watching them all. It’s unlikely that you won’t learn something useful.
While I understand the comparison to plastic sintering, metal sintering is a real and quite distinct 3D printing process. Sintering requires infusion of another, usually much softer metal, into the base metal matrix after the fact. This leads to mechanically more fragile and less predictable results. Laser melting actually leads to (very nearly) full strength parts which can then be processed as if it’s a billet machined part. It’s the pinnacle of current printing technology and if and when it becomes more available it’s bound to revolutionize many fields, as impossible parts become feasible and very worthwhile optimizations (think flow chambers, injectors and such) and weight reductions can be realized.