An unfortunate property of science-fiction is that it is, tragically, fiction. Instead of soaring between the stars and countless galaxies out there, we find ourselves hitherto confined to this planet we call Earth. Only a handful of human beings have ever made it as far as the Earth’s solitary moon, and just two of our unmanned probes have made it out of the Earth’s solar system after many decades of travel. It’s enough to make one despair that we’ll never get anywhere near the fantastic future that was seemingly promised to us by science-fiction.
Yet perhaps not all hope is lost. Over the past decades, we have improved our chemical rockets, are experimenting with various types of nuclear rockets, and ion thrusters are a common feature on modern satellites as well as for missions within the solar system. And even if the hype around the EMDrive vanished as quickly as it had appeared, the Alcubierre faster-than-light drive is still a tantalizing possibility after many years of refinements.
Even as physics conspires against our desire for a life among the stars, what do our current chances look like? Let’s have a look at the propulsion methods which we have today, and what we can look forward to with varying degrees of certainty.
Rulers of Low Earth Orbit
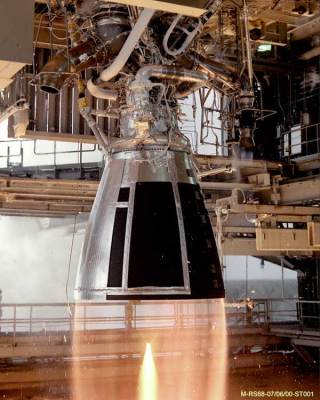
When it comes to getting things into orbit around Earth and keeping them there, we are doing pretty well. Since the early days of the rocket engine in the first half of the 20th century, we have come up with numerous improvements and new technologies. We have developed new solid and liquid fuels and learned to use hypergolic as well as cryogenic fuels. This has made the process of launching new satellites and new probes into orbit around the Earth and on inter-planetary transfer orbits practically a matter of routine.
Once out of Earth’s gravity well, or safely in orbit around the planet, a propulsion method capable of less brute force suffices as the gravitational pull of the Earth is no longer a concern. This is where ion thrusters shine: using relatively small amounts of propellant and the electricity from solar panels or other sources like RTGs, they manage to generate significant amounts of thrust in the form of ion beams. Because ion thrusters have very high specific impulse, they are very efficient in their fuel usage, yet they come with the disadvantage of having very little thrust.
This gets us to the core of the issue with rockets and space-based propulsion: balancing performance between energy required and fuel spent. Whereas a chemical rocket can be easily scaled up to use more fuel for more thrust, its specific impulse is pretty atrocious, meaning that for every unit of fuel burned, most of the energy contained in the fuel is wasted, i.e. not used for the purpose of propulsion.
Specific impulse (Isp) is defined in seconds, where the indicated value specifies for how long the rocket engine or equivalent device can provide thrust to the rocket using the available propellant. This determines the duration of thrust and thus the total acceleration. In addition, chemical rockets get lighter as they use up their propellant, causing the acceleration for the same thrust increases over time. The thrust-to-weight ratio thus determines how well a rocket performs.
As a direct comparison, a chemical rocket such as SpaceX’s Falcon 9 with Merlin 1D (full thrust) engines has an Isp of 311 seconds in a vacuum, and 282 seconds at sea level. Meanwhile the Isp of an ion thruster is measured not in seconds, but in weeks or even months to years. This despite the ion thruster in a satellite or probe having only a fraction of the propulsion that a chemical rocket has. Meanwhile, the ion thruster has a very low thrust-to-weight ratio which prevents it from lifting as much as a sheet of paper out of Earth’s gravity well.
Beyond Earth orbit
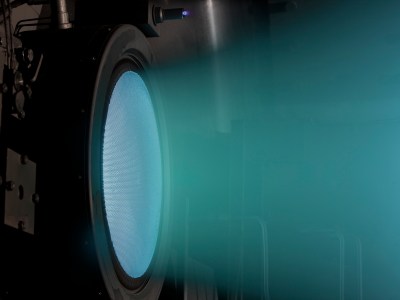
Using these chemical rockets and ion thrusters we can get and keep satellites as well as the International Space Station in orbit, even when they are in lower orbits where atmospheric drag is an issue. And as recently demonstrated by the US, China and UAE by getting the Hope (Misabar Al Amal) & Tianwen-1 (Questions to Heaven) orbiters around Mars, as well as the Perseverance rover on Martian soil, we’re getting pretty good at traveling to at least one of our nearest neighbors in the solar system.
Most of travel within the solar system makes use of orbital mechanics, with the current ESA BepiColombo mission a prime example of this. Instead of traveling in a straight line from Earth to Mercury, this mission spans seven years, during which BepiColombo will use gravity assist: essentially using the gravity of various planets and the Sun of our solar system in order to both gain and lose speed, as well as changing its orbit around the Sun so that it can ultimately align itself with Mercury and park itself in its orbit.
This also shows that another important factor here is one of time. Without the consideration of how long traveling within the solar system or beyond may take, using gravity assist from the Earth and other planets is a valid and very efficient way to travel around in space. The Voyager probes have made it successfully out of the solar system this way, taking only around forty years for this. Of course, outside of scientific missions, discarding the time factor is only an option when one begins to consider something like generation ships.
Much like on Earth, we prefer to travel faster and waste less time. After all, who wants to be stuck in a sailing ship at the Cape on a months-long sailing trip when one can just take an airplane to the East, for example? Similarly, we are looking for ways to travel faster in space.
Going Nuclear
There are a few possible destinations which we would like to travel to faster: one is of course Mars, but other planets in our solar system are also of interest, such as Jupiter’s moon Europa. Here we run into a big issue with both our chemical rockets and our ion thrusters: one cannot provide thrust for long enough, and the other doesn’t provide enough thrust. A possible solution here dates back to the 1950s, in the form of nuclear propulsion.
Many are probably aware of DARPA’s Project Orion, which saw the use of Nuclear Pulse Propulsion as a way to fly to Mars and back in the span of four weeks. While that project never got off the ground, new NPP-based concepts have been worked on. Here much of the most recent research focuses on the use of nuclear fusion in some way to create high-speed exhaust. We see something similar in the general scope of nuclear thermal rockets of which NPPs are a part, where the focus has shifted away from fission and towards fusion. Some, like the Direct Fusion Drive, can be thought of essentially an improved ion thruster.
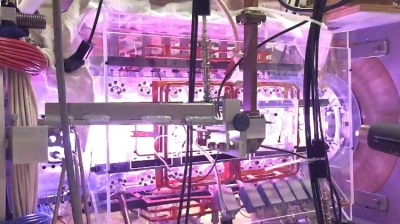
The DFD along with others are some of the concepts which NASA is currently looking at to slash travel times to Mars and other destinations, including for Orion (the spacecraft). The DFD uses findings from the Princeton field-reversed configuration (PFRC) experiments to provide continuous thrust at significantly higher levels than today’s ion thrusters. This would make it suitable for interplanetary travel, with a travel time of four years predicted to travel to Pluto at the edge of our solar system.
Of course, none of this would make interstellar travel outside of our solar system significantly easier.
Making Light of Things
In this universe there are only a few things which are certain. One of which is that space is very big, not to mention very empty. Another is that objects have mass, and yet another being the speed of light (c) exists. The latter two combined dictate a very real limit to how much an object can accelerate. This is problematic in light of challenges such as getting a human being to the nearest star system (Alpha Centauri, 4.37 light years) within that person’s lifespan.
As the most distant human-made object, Voyager 1 is traveling at 1/18,000 of the speed of light, which would mean that it would be capable of reaching Alpha Centauri in approximately 80,000 years. Yet as we’ll see, the solution here is not simply to accelerate more, as this creates two new problems. The first is one of sheer kinetic energy, as the energy required to accelerate to an appreciable fraction of light speed is larger than one could hope to produce with any kind of current or future propulsion method.
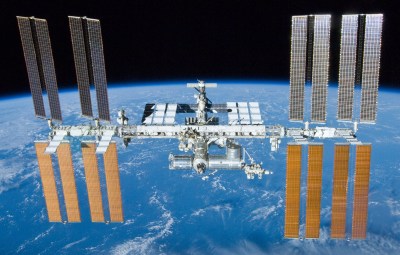
The second problem is defined by general relativity (GR). Simply put, if an object experiences acceleration, then the reference frame of the object and that of any outside observers begin to drift apart. This gravitational time dilation effect in a visual representation means that to an outside observer, a clock held by an accelerating object slows down, while vice versa an outside observer’s clock will seem to move faster than the clock which they are holding.
Although the effect of this time dilation are relatively minor around Earth (e.g. astronauts in the ISS versus people on Earth), the brutal truth here is that we do not want to accelerate significantly at all. That is, unless we wish to deal with situations where the people onboard a spaceship traveling at 0.6c will themselves experience weeks passing during a mission, while back on Earth decades will have passed. This renders even fraction-of-c space unmanned probes relatively pointless.
A potential solution here lies in the concept of a warp drive, also known as the Alcubierre drive and its derivatives. This method essentially allows someone to travel effectively faster than light (FTL), without changing their effective gravity and thus their reference frame. This also avoids the need for enormous amounts of energy.
Physical Warp Drives
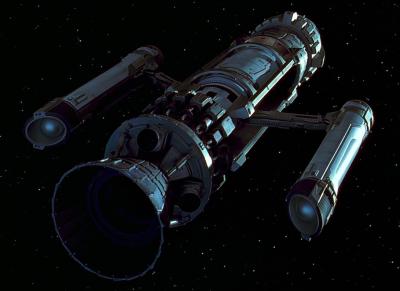
FTL drives are pretty much a staple of science fiction, and take on many forms. Of these, the warp drive is one of the rare few which is both based on scientific theory and which has seen a few decades of study and refinement. At its core the principle is simple enough: the ‘warp drive’ establishes (warp) a shell of space time around the object (‘warp field’), which can then move without having to increase its kinetic energy. Its effective speed would be limited by how rapidly it can warp space time.
A recent addition to the literature on this topic is Introducing Physical Warp Drives by Bobrick et al., which works through the past decades of literature, while creating a classification system for the different warp drive types imaginable.
Most importantly, it covers how an assumption made with the Alcubierre drive — in that it requires a large amount of negative mass — was merely based on a lack of understanding of the underlying theory. Effectively, this means that the negative mass requirement can be reduced or even fully eliminated, and that within the realm of physics there is so far nothing to stop humanity from constructing actual, physical warp drives and embark on FTL trips through the galaxy and beyond.
Space: The Final Frontier?
It would appear then that at least some part of science fiction could within the near future become science fact, with the starships portrayed in the original Star Trek series (TOS, TNG and VOY) on the side of the Federation providing a tantalizing template for what humanity’s future could be like. Interestingly, in the Star Trek universe it’d take until 2063 for an inventor to test the first warp drive.
What our own timeline will look like is still up for grabs, fortunately. Whether we truly will be able to build warp drives in another forty years from now or not, and what we will find out there if we do are all still open questions. As we find ourselves reminiscing about Yuri Gagarin’s historic flight into space sixty years ago, it’s exciting to look ahead, to what the next decades may bring.
What is so bad about going at fraction of light and realize that where one spends weeks in travel, the folks at home spends decades waiting. So a round trip will cause one to see his great grand kids — the same age as oneself?? As I understand it, one wants to travel to a star system ~ 4 1/2 light years away (at .6c, the travel alone will take ~7.5 years..) Hopefully by the time we contemplate travel to a star system that far away, our politics at home would have calmed down,,,, and we will all be unified, as one common people of planet Earth.
“What is so bad about going at fraction of light and realize that where one spends weeks in travel,”
Everything else in the universe slamming into you at that exact same fraction of light.
Also, in order to get “weeks of travel” = “decades at rest” you’re talking about a Lorentz factor on the order of ~50-100 (assuming “10-20 weeks = 20 years” meets that definition – as in, less than a year travel, multiple decades at rest) which is, uh, *a lot* more than 60% lightspeed. It’s within 50 parts per million of lightspeed.
Right now we can handwave a bunch, ignore economic/practical issues and imagine getting something tiny to ~20% the speed of light. Getting to 99.995% the speed of light is far, far past that.
See your great grand kids… At the price of missing most or all of the lives of your kids and grand kids. Does not seem like a good deal to me.
The Coyote books are a decent, somewhat dark take on what you mentioned. The first colony ship is basically hijacked by a crew of patriots fleeing the corrupt government controll and oppression on Earth wanting to build a new world that is as free as possible only to be attacked by the next wave of colonist from a now united communist Earth that believes all mankind everywhere must be enslaved with their twisted ideology. It’s kind of like Red Dawn in space.
Also the Song Benson Arizona from Dark Star comes to mind.
“What is so bad about going at fraction of light and realize that where one spends weeks in travel, the folks at home spends decades waiting.”
Original Twilight Zone – The Long Morrow
Oops, not relativistic effects. He was hibernating. Some results though.
Amused am I at “Soaring”.
In space, no one can hear you scream, and without air, there is no soaring.
What if the warp drive was indeed invented in 2063? It would raise some existential questions.
Hopefully the Vulcans will have some answers.
I could be wrong, but:
Seems like the explanation of specific impulse is wrong, or at least very unlear. “Specific impulse (Isp) is defined in seconds, where the indicated value specifies for how long the rocket engine or equivalent device can provide thrust to the rocket using the available propellant”
The isp defines the efficiency of engine, kind of like miles per gallon for a car. It does not describe the duration that the engine can run for (like “this car will run out of gas in 4 hours”)
See the Wikipedia page you linked in the article.
But hey, maybe I misunderstood
Isp, as commonly used, is bad and incorrect shorthand for the true parameter. But it’s historically the one used.
Its units really SHOULD be N-s/kg. That is, how many newton-seconds of impulse you get per kg of fuel (or more generally ‘propellant’).
However, common usage is derived from the “pound-seconds of impulse per pound of fuel”, a really dumb way to measure it, since the two “pounds” refer to two very different physical parameters (pound force vs. pound mass).
So, in Rational Units, you need to include the (usually omitted) term “G” = 9.8 m/s^2, and Isp = N-s/kg/G.
If you work through the units you will note N-s/kg / (m/s^2) reduces to ‘seconds’
Please, go ahead, lobby for the use of the correct term, where Isp uses units of N-s/kg. See how far that gets you.
“a really dumb way to measure it, since the two “pounds” refer to two very different physical parameters (pound force vs. pound mass).”
Thrust per unit weight. How is that different physical parameters? It’s force per force.
It’s not force per force. One of the pounds is force, one is mass. Confusion caused by a unit system that uses the same name for two very different physical parameters.
What is the force (weight) of a pound of fuel on the moon? So what’s its Isp there?
That’s if you’re imagining “specific impulse” meaning “impulse per unit mass.” You can shift it to “impulse per unit mass per unit reference acceleration,” as the only thing “specific” means is that it’s an intrinsic version of a nominally extrinsic measure. Doesn’t actually mean “per unit mass.”
Now do the units, and you get “Ns/kg” divided by “m/s^2”, which hey guess what. Back to seconds.
Referencing it to a specific acceleration puts it in simpler units, which happens *all the time* in science. A parsec isn’t *actually* a distance, for instance, it’s an parallax-induced angular separation. But everyone knows that the reference parallax distance is Earth’s orbit, so hey, it’s just distance.
Parochial unit shorthand like that promotes confusion and sloppy thinking. Just look how many people keep asking the question.
But it is what it is. There are better fights to pick.
It’s *literally just a definition*. If textbooks define specific impulse as anything other than Isp = thrust/(exhaust mass rate * g), they’re the problem, not the “parochial unit shorthand.”
The version without “g” in the denominator (thrust/exhaust mass rate) already has a name (effective exhaust velocity) it doesn’t need another one.
My rocket engine gets 400 dyne-fortnights to the slug, and that’s the way I likes it!
On Earth, an object of 1 unit of mass at rest on the surface has a mass of one unit, a weight of one unit, and is making a downward force of 1 unit. Take that same object and set it on the Moon. Its mass remains the same but its weight and force are 1/6 of what they were on Earth.
But with rockets, the fuel isn’t just sitting there. The exhaust products of combustion exit the nozzle really fast due to taking up a lot more space than the original fuel and oxidizer. That increases the available force despite the mass not changing.
A down to Earth example. There’s a tall object you want to knock over. You push on it with one hand with all the effort you can muster, but can only *almost* get it to tip. So you make a fist and punch it. Down it goes.
The *mass* you applied didn’t change but the *force* you used increased. Glucose and other chemicals in your muscles provided the energy more rapidly with the punch than with just pushing.
Say pushing for 10 seconds “burned” the same amount of “fuel” as a punch that took half a second. In rocket terms that’s like the difference between a slow burning fuel that can move a craft a long distance with slow acceleration VS the same mass of fast burning fuel that can move a craft a short distance with very fast acceleration.
Rockets use a fast “punch” to lift off then slower “pushes” to keep going.
The ISP is basically how long a unit of fuel will last while the rocket produces thrust equal to the weight of that fuel.
Close: it’s how long it can produce thrust equal to the *initial* weight of that fuel. Obviously over time the fuel’s weight drops in rocketry. It’s usually thought as “how long can a rocket accelerate its initial weight at 1 g.”
Of course, translating that to an actual time means for that to be useful the weight of the fuel would have to be minimal compared to the rocket. So, for instance, if you had a 10 second Isp fuel and the rocket was only 1% of the weight of the rocket (so the mass it’s accelerationg is 100x its weight), it could accelerate it at 1g for roughly (10/1000) s or 0.01 seconds.
Basically, take the rocket equation, expand the logarithm, and if the fuel mass is very small relative to rocket mass, the rocket equation becomes delta-v = Isp * g *(fuel mass/rocket mass). Treating delta-v as acceleration times time, setting a = g, poof, time = Isp * (fuel mass/rocket mass).
I used the words “that fuel” to imply the initial weight.
I watched Scott Manley talk about a nuclear salt water rocket the other day. That actually didn’t sound too far fetched. Maybe something we should seriously consider.
Other than that I really like the Direct Fusion Drive. If it works it seems great. Thrust aside, all that electricity can definately be useful in a probe/crewed ship. Also, strong “The expanse” vibe from that… :)
BUT, ultimately I really really want a warp drive… Humans tend to realise everything we dream up. I wouldn’t be surprised if we one day produce a working warp drive. Preferably in the year 2063. That’ll raise a few eyebrows.
Dammit. This was not meant as a reply.
You’re not wrong. Whereas laymen often have difficulty with scientific concepts (especially when math is involved!) perhaps it could have been expressed differently:
Specific impulse **can be thought of** as the length of time that, ideally, one pound of the propellant could produce one pound of thrust. For example, specific impulse of the Space Shuttle’s SRBs was 266 seconds, so one pound of that propellant in a rocket motor that is designed to produce one pound of thrust would operate for 266 seconds.
You left out the key problem with warp drives: insanely high power requirements. Generating that much energy is going to require harnessing some sort of nuclear reaction quite effectively.
Also, matter-antimatter propulsion was omitted entirely! This is a mistake for sure because it holds a lot of promise. The big problem we face is generating sufficient quantities of antimatter.
Finally, time-dilation is only problematic for non-travelers. Actually getting to the speed where it has a non-marginal impact requires either levels of acceleration our bodies cannot withstand or a very long time under heavy acceleration. Experiencing either is problematic (with our mostly liquid bodies) so we may need to solidify ourselves in some manner. However it’s done, it’s unlikely we will be aware of the passage of time. Ultimately, traveling like this requires dedicating your future making leaving everything behind is inherent. This is bad news for people on Earth but not for those who chose to travel.
One just needs an inertial damping field. (the one ships’ system in all of Star Trek that always seems to work properly)
or you could try reversing the polarity.
Actually, at continuous 1G acceleration (So you have comfy onboard gravity. Neat!) you can reach light speed within a year. At 1.5G acceleration, which is bearable, even if inconvenient, acceleration time is reduced to 7 months. So you don’t need a crazy amount of acceleration to reach a substantial fraction of light speed within a reasonably time frame.
For a sense of scale, at 1G continuous acceleration and deceleration you can reach Neptune in just over 2 weeks!
I used these:
https://www.calculatorsoup.com/calculators/physics/displacement_v_a_t.php
https://www.omnicalculator.com/physics/acceleration
Except it LITERALLY takes forever to reach lightspeed. Unfortunately all of the values of those equations change as you get faster and experience relativistic effects.
Correct. However, even 1 percent of c is quite fast, and relativistic effects wouldnt kick in that much yet. In my neptune travel example, you’d reach 2%c halfway, after which you’d start to decelerate.
It’s almost guaranteed that any “warp drive” type option would require *much more* than a nuclear reaction. Think about it – the energy densities have to vastly exceed the densities of, say, a neutron star, since a neutron star doesn’t do things this anywhere near this screwy to space-time.
Wasn’t there a problem with the “bow shock” of the Alcubierre drive basically toasting everything in the bubble with hard gamma rays?
Yeah, basically. Curved space in general produces particle creation, which freaks some people out when they first learn about it – the best analog I can give them is “Hit a drum, listen to the sound. Pinch a drumhead somewhere, hit it again. Does it sound the same? The difference in sound spectra is the same idea as particle creation.”
I’ve never really considered that a ‘fatal’ issue because c’mon, you’ve got “magic matter” in the front and back, you don’t know what the heck it’s *actually* going to do.
I thought the issue included particles picked up along the way. The issue being that they’re released from the ‘warp bubble’ with incredibly high energy when the ‘bubble’ is collapsed.
I hadn’t seen anything such as that – in Alcubierre’s original idea a worse problem was that the interior was causally disconnected, so you couldn’t even ‘collapse’ it (or direct it at all!) from the inside – and of course you couldn’t model how to “create” or “collapse” the bubble at all because you don’t know the dynamics of creating/destroying the “magic matter” in any case. I’d be highly skeptical of anyone trying to claim modeling stuff like that.
The particle creation issue was pointed out by Finazzi et al. in 2009, and they’re specifically talking about the issues caused by the strong curvature. Their “simple” analog is to point out that the front/back curvatures look like a white/black hole respectively, and so they’ll radiate like they would, as well (realistically the ‘analogy’ isn’t needed, you always get particle creation in curved spacetime, and obviously in this case the curvature is Wacko Huge).
But again, the concerns there don’t hit *too* hard with me because you’re still trying to work in a weird hybrid “semi-quantum-semi-GR” regime. It would’ve been better if Finazzi, for instance, would’ve tried to determine what properties of “magic matter” would be needed to *make* it stable (if you’re going to ask for unicorn pixie dust, might as well ask for *blue* unicorn pixie dust).
Looking forward to the EN drive – an alphabetical increment on the EM drive and many times more thrust (still nill though). Or space shoes that allow you to walk on dark matter.
Any shoes will suffice, fresh asphalt is pretty dark matter. But the expensive ones with the swishes on them might help you gain a little more velocity.
Just don’t moonwalk in them or you’ll violate causality.
The next drive will probably be something like EM++.
Or in 1984 Newspeak ++EM.
Maybe it’ll be a space time anchor.
The Earth is moving pretty fast.
Imagine if you could stand still and let it fall out from under you.
> the Alcubierre faster-than-light drive is still a tantalizing possibility after many years of refinements.
Warp may work, but only below light speed.
https://www.youtube.com/watch?v=8VWLjhJBCp0
Talking about what may or may not work in terms of Alcubierre-type “things” is a bit pointless until both dark energy and inflation are understood. Both of those have/are screwing with space in similar ways to what we would need, but we have no idea how they came about nor if they can be manipulated. This may or may not be equivalent to asking about quantum gravity as well, who knows.
For reference, Lentz’s soliton solution effectively requires a *huge* energy density, to the point where quantum issues may start coming into play (certainly higher than anything in nature – pretty sure if it was purely a matter density it would collapse to a black hole).
Put another way: if you’re writing some hard sci-fi thing and you want to use technobabble that actually has some sanity behind it, say something like “generate an inflaton-catalyzed Alcubierre bubble” or “propelled via a Lentz phantom-energy wave”. Then go and grab some terms from particle physics and incorporate those, too.
If you accept that there are no gravity particles, and gravity is the deformation of space-time caused by mass, or equivalently, mass is the result of deformation of space-time, “light speed” can also be understood also as the speed of causality.
If you caused localized inflation, that would create a huge amount of mass! Other side effects and energy requirements aside, that’s not going to help you accelerate.
” and gravity is the deformation of space-time caused by mass,”
The cosmological constant/dark energy part literally is deformation of space-time *not by mass*. In GR it’s just a magic constant that Just Is and you can’t change it, period. So yeah, that wouldn’t help. But we literally don’t have any idea about that yet.
Also, to be clear, the way warp drives work is completely compatible with general relativity, and all of the statements you made. Matter has to follow causality – the Universe itself does not. That’s literally what inflation was proposed to solve – the CMB appears to manifestly violate causality.
“If you caused localized inflation”
If you completely replicated a little bubble of *original* inflation, that would be a little silly, yes. But again, we have exactly zero idea about what caused inflation in the first place, so it’s hard to say how that field can and can’t be manipulated.
Or just say we’ve licensed the tech from big corporations and nobody really knows how they work and it’s still a thousand years to wait till the patent (that is classified to protect profits from pirates) expires. Done. And it’s a socio-economic scify instantly!
I vote for dyson sphere powered laser sailor
Here’s an interesting antimatter design from Positron Dynamics’ Ryan Weed. https://youtu.be/n2pWv-D84W0
We can send some decent amount of mass to solar system objects by refueling a traditional chemical engine in low earth orbit
We threw a half ton at Pluto with an Atlas launched from sea level more than a decade ago. How much could we have sent if we refueled in orbit? For simplicity in comparing, assume the launch total launch mass from the ground.
*”assume the same total mass launched from the ground”…
Why the same total mass ? That makes no sense. You would launch multiple times that mass.
Keep the launch mass constant to compare fairly. Or normalize it to mass sent vs mass launched from earth if you want; doesn’t matter.
Point being: If I want to launch a specific mass to an outer planet, how much stuff do I need to build and launch from the ground? If I’m not going to save anything in launch mass, why should I build a bunch of piddly little launch vehicles and go through the complication of trying to rendezvous and transfer fuel in orbit?
It’s fun to work through the scenario, and I encourage you to do so. Be sure to account for the delta-V to enter a parking orbit to refuel, vs. a direct shot out. Don’t forget to include the tanker mass and fuel in all that.
“why should I build a bunch of piddly little launch vehicles and go through the complication of trying to rendezvous and transfer fuel in orbit?”
Because, and this might be surprising, it’s harder to control a big giant bomb than a little bomb.
Flippancy aside, your basic question (and the original poster’s point) is wrong. I can get anywhere in the solar system so long as I can get it to a Lagrange orbit and I’m patient enough. Getting to Pluto versus getting to the Moon is just a matter of time, not a matter of launcher capability. Nominally the original poster should’ve said “send some decent mass in a reasonable time”. And a half-ton of mass to Pluto in a decade isn’t either of those things.
NASA’s Gateway presentation has good diagrams on this: you send the fuel the long way, you send the humans the quick way, and have them dock and meet, and it actually saves you significant fuel.
The “piddly little launch vehicles” could be the size of the Starship + SuperHeavy, which are 120 meters high when stacked together. Making something even bigger would be a insanely wasteful project, whereas refuelling in orbit would be just repeating the same process a bunch of times.
The vehicle is design to be fully reusable, so the launch cost would be low. The extra fuel for the launches is cheap.
I don’t think the parking orbit costs anything extra in terms of delta-v compare to a straight shot, except for a bit of fuel used for safe orbital matching and docking.
OK. Sorry I distracted you with the “piddly” comment.
Turn it around: Why should I build a starship-scale rocket, when I could just build a bunch of proven F9’s and refuel in orbit?
Run a model for it. It’s fun and instructive. Don’t forget to include the Oberth term, particularly if you’re considering departing from L4 or L5.
Because a Falcon 9 doesn’t have enough payload capacity to launch humans anywhere useful. Now, bump it up to a Falcon Heavy and it’s basically viable.
This isn’t a fundamental rocketry issue. It’s a practical engineering issue. You need to take into account larger rockets are inherently more difficult/unreliable/costly and only the *humans* need the rapid transit time. Once you add those constraints in, multiple rocket launches and in-orbit refueling becomes much more of an obvious option.
Honestly a slightly modified version of your argument (multiple FH vs single Starship) is essentially what the difference is between NASA and SpaceX’s current approach. Yes, NASA’s got a Big Freaking Rocket in development too, but that’s politics: the entire Artemis program is evolving towards a “pure Falcon Heavy” approach.
And 1) you could do a *lot* of manned Solar System exploration with just that design, and 2) it’s actually quite fuel *efficient* once you take into account the human constraints. It becomes a giant obvious win once you’ve got enough infrastructure at your target that all you need is fuel.
There are plenty of presentations on Artemis/Gateway which show the delta-V budget advantage for in-orbit assembly and refueling when taking human constraints into consideration.
Screw this nonsense, all we actually need for ftl is an improbality generator and a nice cup of tea.
Don’t forget your towel….
And you need the processor, Bambleweeny 57 or higher.
Pangalactic Gargleblaster is always good….
One nit to pick: specific impulse is about the mass of fuel carried vs total thrust, not *energy*. Ion drive ISP would make no sense – the atoms being accelerated don’t “have” energy at rest. Ion drives have high ISP becase they take a relatively small mass and produce higher thrust per mass expended. The rocket equation sucks :)
If you’re going to pick nits, then please get your terms correct.
Specific impulse is about the mass of fuel carried vs. total *impulse*, not thrust. Says so right on the tin. Further, for a given amount of energy, the thrust *decreases* with increasing Isp.
Isp for a ion drive is perfectly sensible, and defined exactly the same way as for chemical rockets. It’s the impulse you get per unit mass of propellant. In an ion drive the energy source isn’t the propellant, but Isp doesn’t care about that.
Another problem with interstellar travel is the communication. The radius of the receiver dish on Earth must grow at the same rate as the distance, which quickly gets impractical. We’re already struggling to get data from pioneer/voyager craft.
Use stars to focus, problem solved.
Won’t work if you’re still on your way to the first star.
That’s a wavelength issue. Boost yourself up to optical frequencies and the receiving capabilities are definitely there. Not easy, but definitely not impractical.
With the 22 watts Voyager transmits, the received power on a whole 70-meter DSN dish is less than the power of a single optical photon per second. Can’t carry much data with that.
Yes, you can increase the transmitter power and increase its antenna gain and size (and improve its pointing accuracy accordingly), but you’ll still need huge antennas (telescopes) — The Friis equation rules here.
It’s a tradeoff, for sure, but going all the way up to optical frequencies is going way too far — the shot noise (Poisson statistics) kills you.
Can you make an ion drive into a spark gap transmitter? If so, a one way transmission can be encoded by modulating on and off the ion drive.
If you have the electrical power to run an interstellar-class ion drive, I’m sure you’ll have a few kilowatts to spare for a K-band transmitter, and the mass budget for an antenna a few dozen meters in diameter.
Besides, I’m sure the FCC would consider even interstellar space under their authority, and they banned spark transmitters decades ago. :-)
I can’t remember what kind of drive, but something like this is mentioned in one of the Venus Equilateral stories, by George O. Smith.
“With the 22 watts Voyager transmits, the received power on a whole 70-meter DSN dish is less than the power of a single optical photon per second.”
Yeah. Because the beam from a 4 meter dish at 8 GHz has a spot size on the order of half a degree. Project that to Earth’s distance and the power’s spread over a *huge* area. Spot size at optical frequencies is obviously thousands of times smaller, power gets spread over an area that’s thousands-squared times smaller, power goes up correspondingly.
“The Friis equation rules here.”
Yah. It does. Use the original one, from 1946. Keep antenna sizes fixed. Received power goes as 1/lambda^2. In other words, a thousand times smaller wavelength, a million times higher power, because the beam can be focused more and spreads out less.
Yes, that’s counter to how most people think about it, because you usually imagine comparing equal gain antennas, which have different sizes. Gain of a fixed-size antenna goes up as the wavelength shrinks.
The laser communications system for Breakthrough Starshot was probably one of the few things in it that I thought was viable. arXiv:2005.08940. 50 dBm starting power, +140 dBi transmit gain, +156 dBi receive gain, -476 dB path loss, -3.5 dB relativistic loss, resulting power of -133 dBm, or 288 photons/sec at the laser wavelength. And honestly, a 30 meter receive telescope is pretty conservative.
But that’s not terribly surprising, since the benefits of optical communication for interstellar communications have been around since the 1960s by Cocconi and Morrison.
What? We got interstellar traveling capability but no sub-space radio capability? I feel cheated…
This is actually an interesting point that most of the people messing around with, uh, “creative metrics” in general relativity strangely ignore. They’re all focused on stable warp bubbles with human-survivable conditions on macroscopic scales, which is a weird starting goal to have. Just being able to demonstrate you can send *any* spatial distortion at faster-than-light speeds – of *any* scale, for any length of time – would be a breakthrough.
A review of this scope is no easy task. I could do without the distracting royal “we” because I didn’t do any of these things. Here are a couple notes.
In low Earth orbit “the gravitational pull of the Earth is no longer a concern.” Definitely an idea from cheap TV space fiction.
Ion engines “generate significant amounts of thrust in the form of ion beams” –> generate thrust by accelerating ions.
I suspect fusion reactors that are not huge will be a game changer.
In English the pronoun “we” doesn’t necessarily include “you”, all you know is that the speaker includes themselves and someone else.
True, but the other(s) is never mentioned or credited. It doesn’t engage me like “I” or “you”.
As a professor of aerospace engineering, let me just say that there are portions of this article that could be cleaned up, as they are misleading. “meaning that for every unit of fuel burned, most of the energy contained in the fuel is wasted, i.e. not used for the purpose of propulsion”
That is highly misleading. That fuel is not at all “wasted.” The rocket thrust equation has two terms, a momentum ejecta term (mass flow rate x nozzle exit velocity) and a nozzle exit pressure differential term. The pressure differential term is very small compared to the momentum ejecta term, and indeed it becomes zero once you have left any atmosphere that creates opposing aerodynamic drag. The bottom line is that the momentum ejecta term needs to be large for a launch vehicle in order to generate sufficient amount of thrust to not only overcome aerodynamic drag, but also to accelerate the vehicle to orbital velocity. So it is by no means “wasted.”
This and other areas of this article could really use some improvement so as to not misinform the non-technical audiences that may read it.
The mass of the fuel is used, but the chemical energy in the fuel is not, it would be less wasteful if all of the chemical energy were released in the rocket engine before ejecting its mass.
How about communication by entangled quantum spin? Separate particles located at source and on the vehicle may theoretically sync immediately without time lag. Just develop a nice binary coding protocol and detector, and … https://quantumxc.com/is-quantum-communication-faster-than-the-speed-of-light/
The problem with the usual descriptions of entangled communication is that it definitely does not work faster than light. It works at the same speed as the receiver. You can’t send information until you carry the entangled object to the destination, so add in the trip time.
It’s also important to note that in entanglement cannot send information faster than light because the two ends need to communicate over light-speed channels to compare their measurements with what was sent.
Once you have both the sent values and the received values, you can verify that information was sent across the entangled connection, but without both sets of data, the received information is indistinguishable from noise.
This is the fundamental reason why entanglement cannot be used for instantaneous communication. It’s something that the sci-fi authors ignored in creating the ansible.
I feel that in this point in space travel we are like a group of cavemen who have just floated across a small river on a log and then seeing the ocean and say “We’ll never be able to get across that!” Of course we did get across oceans and into the air and then into space. Interstellar, I believe is just a matter of learning and time, maybe we won’t go to the stars but our descendants will.
A few nitpicks. First this one: “Meanwhile the Isp of an ion thruster is measured not in seconds, but in weeks or even months to years.” Current off-the-shelf ion drives have an Isp of around 3000-ish seconds. Some still-in-the-lab designs push this to the low 10,000’s. An Isp of “years” is not actually possible as it would require and exhaust velocity higher than the speed of light.
Next, “…a spaceship traveling at 0.6c will themselves experience weeks passing during a mission, while back on Earth decades will have passed.” Also not quite, but definitely the right idea. The gamma factor from the Lorentz transform at 0.6c is only a little above one. To get to that level of gamma (decades in weeks), one would need to travel in the neighborhood of 0.99999something-c.
And yes, I am actually a rocket scientist.
Seems to me there’s a confusion between I_sp and running time, with Deep Space 1 having fired its thrusters for ~10k hours by end of mission, and Dawn ~15k. That works out to ~1.1 and ~1.7 years.
Also, just for fun: The minimum speed for the gamma factor to convert weeks to decades (51 weeks to 20 years) is 0.999C. For 2 weeks to become 2 decades, it’s 0.999998C.
One thing no one has mentioned. Warp drive is unnecessary if we can end aging. Most talk of space travel is in terms of a human lifespan. “It would take more than a lifetime to reach the nearest star.” etc. But if we extend the average human lifespan by a couple orders of magnitude, trips to nearby stars will be quite reasonable. I’m willing to bet that human life span extension will happen before practical warp drive.
If you want to be stuck in a box for 80 thousand years, be my gues.
Loved the article, Maya! It was a great intro for a lay person like me to get a good overview of the state of space propulsion.
propulsion systems have a ceiling, the next frontier is spatial/gravitational force warp. The basic concept is not moving to a location, but moving the location to you.
To help visualize such a thing.,,
Imagine a paper map…
Now, you can either move a toy car across the paper map and reach the destination that way (simulating propulsion systems)
or
You can fold the map and position your destination right in front of you. (Spatial/Gravitational force warp)
You should consider reading the whole article.
I am kind of astounded that no one has mentioned that Alpha is not the closest star; Proxima Centauri is.
“The third member, Alpha Cen C (also known as Proxima), is a much smaller red dwarf star that travels around the AB pair in a much larger orbit that takes it more than 10 thousand times farther from the AB pair than the Earth-Sun distance. Proxima currently holds the title of the nearest star to Earth, although AB is a very close second.” (https://www.nasa.gov/mission_pages/chandra/images/alpha-centauri-a-triple-star-system-about-4-light-years-from-earth.html)
Did… did you not notice that what you quoted said Proxima Centauri’s name is actually “Alpha Centauri C”? It’s the smallest star in Alpha Centauri.
Alpha Centauri is a star system, not a star, which is exactly what the article said. If you go to Proxima, you’re going to Alpha Centauri.
There are about 30 videos on Google of an ion thruster that lifts its power supply against Earth’s gravity… It has also lifted optional propellant tanks! It may need lightweight solar panels or better batteries to reach orbit etc., however, there are solar cells that can produce 23 watts per gram. Please see US Patent number 10,119,527 to find flight footage and other information.
Won’t work in vacuum will it?
it will work in a vacuum and will have fantastic performance!
The future will be antimatter propulsion for future space travel using on-board antimatter production.
Probably must more credible to sent a (minuscule) 3D printer for living creature at close to light speed and enable it once it has arrived. You can communicate at light speed (not so fast in space, but, AFAIK, the fastest we can reach) and start replicating your colony from here.
kinda the storyline of (the very good) https://bobiverse.fandom.com/wiki/We_Are_Legion_(We_Are_Bob)_Wiki
https://pronewsreport.com/2021/05/31/antigravity-flying-saucer-for-solar-system-exploration/
antigravity that can use antimatter for propulsion.
It’s been a while since I replied to this thread.
I forgot to announce earlier, 2 of my grandsons built rocket engines in college, and the younger one is now hired by Blue Origin or something. His title actually is “Rocket Scientist”. The other one designs navigation software and travels around the globe. The youngest one (third one) designed a “Particle Detector” for his school science project. (He was 8) He’s 12 now, (13 in January)
My wife, (R.I.P) built position thrusters for satellites. (I can’t say which ones)
I may or may not have performed some computer work involving *****************
Happy New Year..
I am building an Impermeable Plasma Barrier (shaped like a bubble) – that also has a new form of magnetic propulsion called Tubular Linear Induction Motors.
Please have a look – design and construction photos are located at :
https://drive.google.com/drive/folders/1-3TDKlwpr2n6tQp0ypg-qxa6P_vOIytA