In many ways, the human body is like any other machine in that it requires constant refueling and maintenance to keep functioning. Much of this happens without our intervention beyond us selecting what to eat that day. There are however times when due to an accident, physical illness or aging the automatic repair mechanisms of our body become overwhelmed, fail to do their task correctly, or outright fall short in repairing damage.
Most of us know that lizards can regrow tails, some starfish regenerate into as many new starfish as the pieces which they were chopped into, and axolotl can regenerate limbs and even parts of their brain. Yet humans too have an amazing regenerating ability, although for us it is mostly contained within the liver, which can regenerate even when three-quarters are removed.
In the field of regenerative medicine, the goal is to either induce regeneration in damaged tissues, or to replace damaged organs and tissues with externally grown ones, using the patient’s own genetic material. This could offer us a future in which replacement organs are always available at demand, and many types of injuries are no longer permanent, including paralysis.
Everything Begins with Knowledge
Our level of understanding of human physiology and that of animals in general has massively expanded since the beginning of the 20th century when technology allowed us to examine the microscopic world in more detail than ever before. Although empirical medical science saw its beginnings as early as the Sumerian civilization of the 3rd millennium BCE, our generalized understanding of the processes and components that underlie the body’s functioning are significantly more recent.
DNA was first isolated in 1869 by Friedrich Miescher, but its structure was not described until 1953. This discovery laid the foundations for the field of molecular biology, which seeks to understand the molecular basis for biological activity. In a sense this moment can be seen as transformative as for example the transition from classical mechanics to quantum mechanics, in that it changed the focus from macroscopic observations to a more fundamental understanding of these observations.
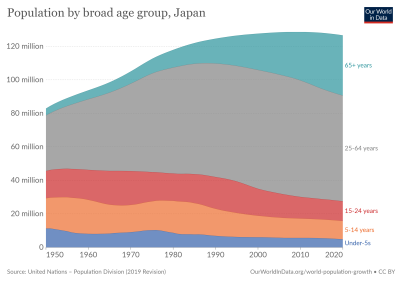
This allowed us to massively increase our understanding of how exactly the body responds to damage, and the molecular basis for regenerative processes, as well as why humans are normally not able to regrow damaged limbs. Eventually in 1999 the term ‘regenerative medicine’ was coined by William A. Haseltine, who wrote an article in 2001 on what he envisions the term to include. This would be the addressing of not only injuries and trauma from accidents and disease, but also aging-related conditions, which would address the looming demographic crisis as the average age of the world’s populations keeps increasing.
The state of the art in regenerative medicine back in 2015 was covered by Angelo S. Mao et al. (2015). This covers regenerative methods involving either externally grown tissues and organs, or the stimulating of innate regenerative capabilities. Their paper includes the biomedical discipline of tissue engineering due to the broad overlap with the field of regenerative medicine. Despite the very significant time and monetary requirement to bring a regenerative medicine product to market, Mao et al. list the FDA-approved products at that time:
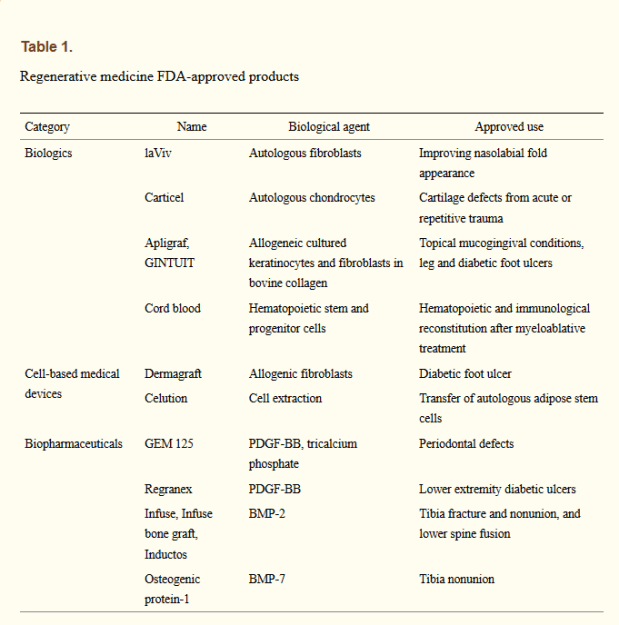
While these were not miracle products by any stretch of the imagination, they do prove the effectiveness of these approaches, displaying similar or better effectiveness as existing products. While getting cells to the affected area where they can induce repair is part of the strategy, another essential part involves the extracellular matrix (ECM). These are essential structures of many tissues and organs in the body which provide not only support, but also play a role in growth and regeneration.
ECM is however non-cellular, and as such is seen as a ‘medical device’. They play a role in e.g. the healing of skin to prevent scar tissue formation, but also in the scaffolding of that other tantalizing aspect of regenerative medicine: growing entire replacement organs and body parts in- or outside of the patient’s body using their own cells. As an example, Mase Jr, et al. (2010) report on a 19-year old US Marine who had part of his right thigh muscle destroyed by an explosion. Four months after an ECM extracted from porcine (pig) intestinal submucossa was implanted in the area, gradual regrowth of muscle tissue was detected.
An important research area here is the development of synthetic ECM-like scaffolding, as this would make the process faster, easier and more versatile. Synthetic scaffolding makes the process of growing larger structures in vitro significantly easier as well, which is what is required to enable growing organs such as kidneys, hearts and so on. These organs would then ideally be grown from induced pluropotent stem cells (iPS), which are a patient’s own cells that are reverted back to an earlier state of specialization.
Swapping parts
It should come as little surprise that as a field which brings together virtually every field that touches upon (human) biology in some fashion, regenerative medicine is not an easy one. While it’s one thing to study a working system, it’s a whole different level to get one to grow from scratch. This is why as great as it would be to have an essentially infinite supply of replacement organs by simply growing new ones from iPS cells, the complexity of a functional organ makes this currently beyond our reach.
Essentially the rule is that the less complicated the organ or tissue is, the easier it is to grow it in vitro. Ideally it would just consist out of a single type of cell, and happy develop in some growth medium without the need for an ECM. Attractive targets here are for example the cornea, where the number of people on a waiting list for a corneal transplant outnumber donor corneas significantly.
In a review by Mobaraki et al. (2019), the numerous currently approved corneal replacements as well as new methods being studied are considered. Even though artificial corneas have been in use for years, they suffer from a variety of issues, including biocompatibility issues and others that prevent long-term function. Use of donor corneas comes with shortages as the primary concern. Current regenerative research focuses on the stem cells found in the limbus zone (limbal stem cells, LSC). These seem promising for repairing ocular surface defects, which has been studied since 1977.
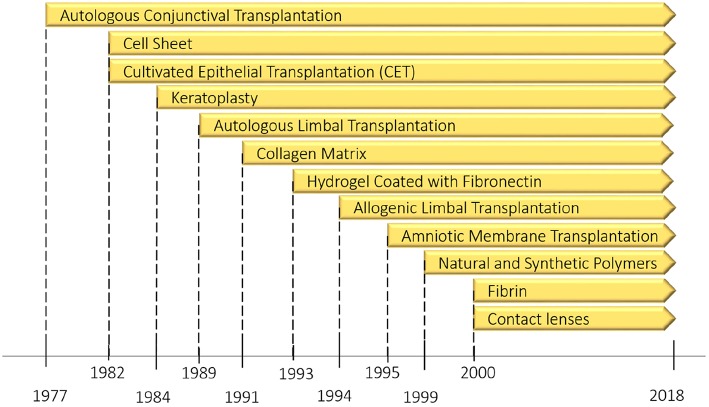
LSCs play a role in the regular regenerative abilities of the cornea, and provide a starting point for either growing a replacement cornea, or to repair a damaged cornea, along with the addition of an ECM as necessary. This can be done in combination with the inhibiting of the local immune response, which promotes natural wound healing. Even so, there is still a lot more research that needs to be performed before viable treatments for either repairing the cornea in situ, or growing a replacement in vitro can be approved the FDA or national equivalent.
A similar scenario can be seen with the development of artificial skin, where fortunately due to the large availability of skin on a patient’s body grafts (autografts) are usually possible. Even so, the application of engineered skin substitutes (ESS) would seem to be superior. This approach does not require the removal of skin (epidermis) elsewhere, and limits the amount of scar formation. It involves placing a collagen-based ECM on the wound, which is optionally seeded with keritanocytes (skin precursor cells), which accelerates wound closure.
Here the scaffolding proved to be essential in the regeneration of the skin, as reported by Tzeranis et al. (2015). This supports the evidence from other studies that show the cell adhesion to the ECM to be essential in cell regulation and development. With recent changes, it would seem that both the formation of hair follicles and nerve innervation may be solved problems.
Still a long way to go
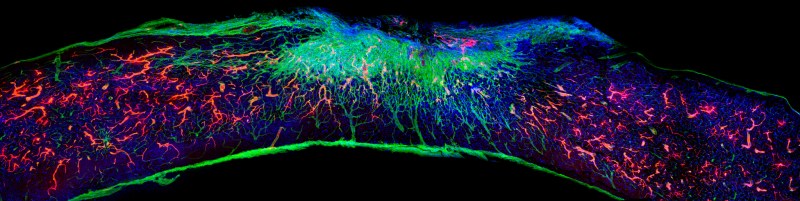
It will likely still be a long time before we can have something like a replacement heart grown from a patient’s own iPS cells. Recent research has focused mostly on decellularization (leaving only the ECM) of an existing heart, and repopulating it with native cells (e.g. Gálvez-Montón et al., 2012). By for example creating a synthetic scaffold and populating it with cells derived from a patient’s iPS cells, a viable treatment could be devised.
Possibly easier to translate into a standard treatment is the regrowth of nerves in the spinal cord after trauma, with a recent article by Álvarez et al, (2021) (press release) covering recent advances in the use of artificial scaffolds that promotes nerve regeneration, reduces scarring and promotes blood vessel formation. This offers hope that one day spinal cord injures may be fully repairable.
If we were to return to the ‘body as a machine’ comparison, then the human body is less of a car or piece of heavy machinery, and more of a glued-together gadget with complex circuitry and components inside. With this jump in complexity comes the need for a deeper level of understanding, and increasingly more advanced tools so that repairs can be made efficiently and with good outcomes.
Even so, regenerative medicine is already saving the lives of for example burn victims today, and improving the lives of countless others. As further advances in research continue to translate into treatments, we should see a gradual change from ‘you’ll have to learn to live with that’, to a more optimistic ‘give it some time to grow back’, as in the case of an injured veteran, or the victim of an accident.
“Much of this happens without our intervention beyond us selecting what to eat that day.”
So things like drinking, smoking, unprotected sex, gambling, lack of safety gear, selecting how much to eat, avoiding exposure to radioactive materials and a host of other things have no impact on and are irrelevant to the repair process?
It’s way, way more complicated than that. Here’s some ATP synthase. That is happening extremely often and extremely quickly and is just one small part of the daily biology required for human life.
https://www.youtube.com/watch?v=bErWGcxnFHo
Gambling? You playing Russian Roulette?
Losing money to criminals can be very bad for your health.
Losing money *to* the criminals is what we do every day, and is mostly harmless.
Criminals losing money to you is the unhealthy one.
Interesting article, thanks. But I find the two graphs (reproduced from elsewhere) very misleading.
On “Population by broard age group, Japan” the coloured bands do not represent the same number of years. Of course the grey band looks larger – it represents a span of 39 years, while the dark blue band represents a span of 4 years. Makes it very difficult to infer anything from the data.
On “The evolution pathway of ocular surface reconstruction…” the horizontal (time) axis is all over the place – the one year spacing between 1999 and 2000 for instance is noticably larger that the preceding 4 years.
Two examples of manipulating the data presentation format to exaggerate a point someone is trying to make. My biggest bugbear in this regard is stock market price graphs that do not start at 0 on the vertical axis, and are sometimes non-linear, making say a 0.5% drop look like a huge and alarming crash.
After the break…???
There is no break, you must read it all, there is no tea for you.
So what qualifies a HAD article for forced distro, dumping the whole silly wetdream all over our screens with no fold?
There is already a proven allograft spinal cord repair method, yet AFAIK it has only been used on one human, after a long research period starting with mice, then rats, then dogs. It takes ensheathing cells from the olfactory nerve (which in anyone who doesn’t suffer from anosmia is a nerve that rapidly regenerates) and injects them at the site of spinal cord damage. The one human it’s been done on had his spinal cord completely severed when he was stabbed in the back a few years prior to this treatment. The last info I can find on him, from some years ago, was he was able to get around with a walker. I’d expect that treatment given ASAP after injury would have better/quicker results.
Another treatment that’s worked in testing to halt self damage of the spinal cord after an injury is a large dose of Brilliant Blue G food dye. The dye binds to the same receptor sites as the inflammation causing chemicals released by nerve cells when they’re injured. Those chemicals cause swelling and rapid firing of the spinal nerves until they “burn out” at the injury site, often to the point they recover little, if any. Blocking the swelling and other bad responses to injury preserves the nerve cells so they can heal. As for side effects, in rodent tests the dye temporarily turned their skin and the sclera of their eyes a bright blue. Would you rather be in a wheelchair for life or look like a Fremen Smurf for a while?
For treating injured corneas, it’s been shown that as long as part of the limbus (edge) of one cornea is intact, stem cells can be harvested, cultured to grow more cells, then spread across the injury where they’ll go to work replicating and replacing the injured tissue. Yet this hasn’t become common practice despite first being done many years ago.
For treating presbyopia an eyedrop has been developed in China which restores the flexibility enzyme almost every human eye lens starts losing around the age of 45. So what does an American company produce? An eyedrop that does the opposite of belladonna. It constricts the iris to make the pupils shrink super small to increase the depth of field, just like stopping a camera lens way down. But then the amount of light entering the eye (as it is with a camera) is reduced a lot.
Lens replacement for cataracts is big business in the USA. That’s despite a treatment existing for infants born with cataracts. All but the outermost layer of lens cells in the lens capsule are removed then the lenses will fully regenerate, free of cataract and clear. Tests have been done on some lizards where their lenses were removed and regenerated multiple times. The procedure has been tested in China on dogs. So why is this procedure not available for adult humans? MONEY! Getting artificial lenses costs a lot of money. Then there’s the life long need to buy reading glasses, whether you’ve have lens replacement for cataracts or just have presbyopia.
Regarding the eye drops for lens flexibility… they were in stage 2 trials for FDA approval last I heard.
Novartis just announced a related investment:
https://www.aao.org/headline/novartis-to-buy-topical-presbyopia-treatment
Logarithmic vertical axis for stock price graphs is the most useful. By its very nature, it can’t descend to zero.
It will be a long, long time before we see entire organs grown in vitro. The primary issue has always been the need to deliver nutrients to the growing cells. Typically this is limited by diffusion, which only allows a few layers of cells to develop before the concentration of nutrients drops below the level required to sustain cell growth. This is why skin graft materials exist: they conveniently grow in large, thin sheets. To successfully grow thicker organs, a distribution system (blood vessels) must accompany the growth of the organ, but again, the entire culture gets limited by diffusion until you can actively pump nutrients through that distribution system. It’s kind of a chicken before the egg thing. In recent years, some promise was shown in 3-d printing live cells into structures. Taken to the extreme, this approach could allow entire organs to be fabricated from numerous types of cells. Significant challenges remain however, getting the printed cells to adhere to each other, and assembly and implantation of an organ before it dies are two such hurdles. Take good care of your body, we won’t be seeing spare parts anytime soon.
Two Maya articles in a row? What is this, Christmas?
To get more advanced regeneration of (parts of) our body, we need more funding and awareness for the field of biogerontology/healthy longevity. Some people will indeed lose body functions due to external damage, but for most of us it is more likely due to ageing.
People living in the EU can demand more funding and awareness for healthy longevity from the European Union, by endorsing this proposal on the official Conference on the Future of Europe forum: https://futureu.europa.eu/processes/Health/f/3/proposals/826 .
I did btw not know that our liver can regrow! That’s pretty cool and maybe a there is a common mechanism here to use in other body parts as well. I am still studying biology, so I don’t know everything there is to know yet. But epigenetics might be the thing here that allows the liver to do this.
I’ve worked in the Medical Engineering world and High Level Network Engineering all my life…. at the same time. The claims here seem to be that we are evolving to adapt. Sorry… You are stuck with what you inherited when you were born. Add another 100 yrs and we got it.