Neutrinos are some of the most elusive particles that are well-known to science. These tiny subatomic particles have no electric charge and an extremely small mass, making them incredibly difficult to detect. They are produced in abundance by the sun, as well as by nuclear reactions on Earth and in supernovae. Despite their elusive nature, scientists are keen to detect neutrinos as they can provide valuable information about the processes that produce them.
Neutrinos interact with matter so rarely that it takes a very special kind of detector to catch them in the act. These detectors come in a few different flavors, each employing its unique method to spot these elusive particles. In this article, we’ll take a closer look at how these detectors work and some of the most notable examples of neutrino detectors in the world today.
Patience and Scale
Modern physics tells us that around 100 trillion neutrinos pass through your body every second. You’d think being so common would make these particles easy to find, but it’s anything but the case. These ultralight uncharged particles interact with matter so rarely that detecting them requires a rather specialized experimental setup.
The first successful neutrino detection was achieved in 1956 by Frederick Reines and Clyde Cowan. Two targets were created, using a solution of cadmium chloride in water, with scintillation detectors placed next to the targets. Antineutrinos from a nuclear reactor underwent an “inverse beta decay” with protons in the water. This reaction saw the proton turn into a neutron, and the antineutrino forming a positron. The positron quickly annihilated with an electron, releasing a gamma ray, while the neutron was captured by a cadmium nuclei, itself releasing a gamma ray a few microseconds later. By capturing the gamma ray signature of these events, the duo proved a successful detection of an antineutrino, which would later see them awarded the Nobel Prize in 1995.
This method was useful for detecting neutrinos, but little more than that. To learn more about the universe, physicists needed to study neutrinos in greater detail, determining their natural sources, their interactions, and their behaviour. Thus, a variety of more advanced detectors have been built over the years. Many of these are at a grand scale, involving hundreds of tons of this, or thousands of tons of that. The sheer scale is often required to capture a rare interaction with a neutrino, given their propensity to pass through great expanses of material without any interaction whatsoever.
Cherenkov Radiation
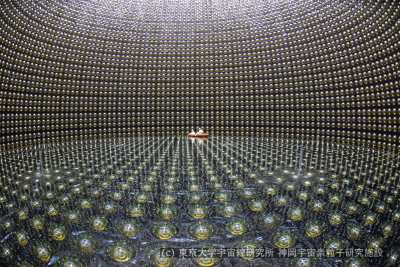
A more modern and popular method of neutrino detection is via Cherenkov radiation, which has netted scientists richer information on neutrinos and their origins. When a neutrino moves faster than the speed of light in a given material, like water, Cherenkov radiation is produced in a sort of optical shockwave, analogous to an airplane breaking the speed of sound in air. The ring of light released can be detected with simple photomultiplier tubes. With an appropriate array of photodetectors, it can be possible to determine the direction and energy levels of incident neutrinos.
These detectors use large tanks filled with water, heavy water, or oil, and are equipped with sensors that can detect the faint flashes of Cherenkov radiation produced when a neutrino interacts with matter. A prime example of a water Cherenkov detector is the Super-Kamiokande in Japan, a massive underground tank holding 50,000 tons of ultra-pure water, lined with 11,000 photomultiplier tubes. The whole experiment is buried a kilometer underground, helping to shield it from other natural phenomena like cosmic rays. It’s set to be superceded by the Hyper-Kamiokande in coming years. Another example is the Sudbury Neutrino Observatory, with 1,000 tons of heavy water surrounded by a cylinder of regular pure water. The experiment is able to capture the gamma rays released when a neutrino breaks up a deuterium atom in the heavy water.
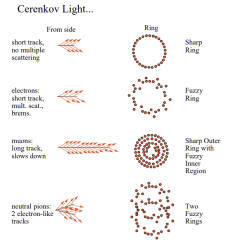
Los Alamos National Laboratory
The MiniBooNE detector works on the same scintillation principal, but uses oil as its medium instead of water. It was designed to investigate the concept of neutrino oscillation, where neutrinos change between several “flavors” over time. The experiment hunted for the signature of an electron neutrino hitting a neutron—which would generate an electron plus a slow-moving proton—which occurs rarely. This would be contrasted against the signature of more common events where muon neutrinos struck protons, creating a muon and a proton. These different events can be determined by patterns of light detected by the experiment’s photomultipliers, as different particles at different speeds create their own telltale patterns of Cherenkov radiation.
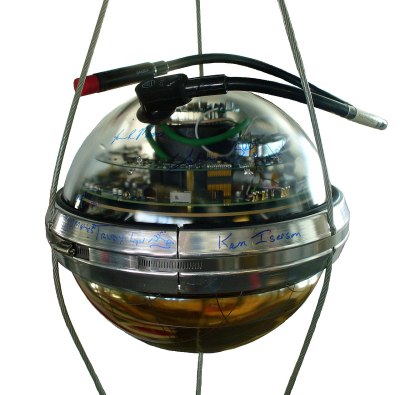
Other Cherenkov detectors eschew the use of a giant purpose-built container, instead electing to take advantage of naturally-existing bodies of water or ice. The Antares experiment sits at the bottom of the Mediterranean Sea, 2.5 km below the surface. As a result, it has to filter out light caused by things like radioactive decays from potassium-40 in sea salt, and bioluminescent organisms. Meanwhile, at the South Pole, the AMANDA and IceCUBE experiments use photodetectors in holes drilled deep into the ice.
Time Projection Chambers
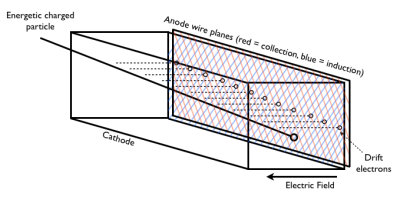
Time-projection chambers represent another method of neutrino detection, where a neutrino interaction ionizes atoms in a gas or liquid, and the resulting trail of charged particles is then detected. The DUNE experiment in South Dakota, USA, currently under construction, is an example of a project employing this method. With its four massive detectors, each holding thousands of tons of liquid argon, DUNE aims to study neutrinos with unprecedented precision. The density of the liquid argon helps increase the chances of an interaction with a neutrino.
Time-projection chambers use light detection just like scintillator experiments, but also go further. The chambers use a cathode plane to create an electric field across the chamber. On the opposite side of the chamber, there are multiple planes of parallel anode wires. Inner planes are typically termed as induction grids, which allow so-called drift electrons from neutrino-particle interactions to pass by. If a drift electron passes by a wire in the induction plane, it produces a bump in the current in the wire which can be picked up. Beyond the one or more induction grids, a collection grid then picks up electrons directly, outputting a signal for collection. The benefit of having multiple planes of anode grids is that it allows a two-dimensional reconstruction of an ionization event to be made as an electron is picked up moving by the multiple grids.
Gallium
If you’re less interested in directionality and more interested in quanitities of neutrino interactions, liquid metal can be a useful tool. In a gallium detector, neutrinos pass through a tank filled with, wait for it, gallium. Thanks to the neutrino-induced inverse beta decay reaction, the neutrino impacting the gallium atom sees one of the atom’s neutrons become a proton, turning gallium into germanium and releasing an electron. The produced germanium can be chemically extracted and due to its instability, its decay can be detected with proportional counters.
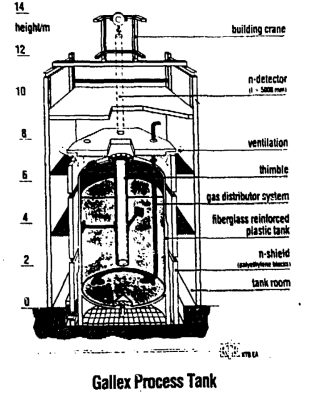
The GALLEX experiment in Italy is one of the notable examples of gallium-based neutrino detectors. Located deep underground to shield it from cosmic rays, the GALLEX detector consisted of a tank holding 30 tons of liquid gallium, and ran from 1991 to 1997. This experiment played a crucial role in studying solar neutrinos, and its results have significantly contributed to our understanding of the Sun and its processes. It’s successor was the Gallium Neutrino Observatory, which was in operation from 1998 to 2003. The Soviet-American Gallium Experiment, or SAGE, was another long-running gallium-based neutrino detector. These experiments were prized for their ability to detect low-energy neutrinos, though they were expensive due to their requirement for many tons of gallium, either in liquid metal form or as a gallium trichloride-hydrochloric acid solution.
Conclusion
The nature of particle physics today is that a wide variety of large-scale experiments are needed to investigate all manner of phenomenon. This is by no means an exhaustive list of neutrino detection methods, but instead a guide as to the many methods that can be used to hunt down these elusive particles. New and more exciting detectors will be built, and hopefully reveal to us more secrets about the sub-atomic particles beyond the basic ones we learn about in high-school physics. If you’re currently studying particle physics at the university level, you may yet find yourself working on one of these advanced neutrino projects!
Interesting article, but neutrinos don’t move “faster than the speed of light.” At least not according to these folks:
https://www.science.org/content/article/once-again-physicists-debunk-faster-light-neutrinos
“When a neutrino moves faster than the speed of light in a given material, like water, Cherenkov radiation is produced ”
Faster than light in the (present) medium. You get Cherenkov radiation when the photos “realize” they are moving too fast for where they are.
It’s actually the medium that produces the radiation, because it’s the medium that generates the slowing of an electromagnetic wave in the first place. Basically the molecular lattice gets “yanked” out of place, and then the radiation produced is the overall response of the medium (simplified, yes, but close enough).
There’s a similar effect which happens when a charged particle crosses between two media with different indices of refraction (like between air and water) called transition radiation. It’s the same overall idea, although transition radiation happens at all particle speeds (because you’re still disturbing the medium).
Yes. At the detailed level, no argument there.
Neutrinos going faster than the speed of light in a medium do not emit Cerenkov radiation. the article is incorrect. Cerenkov radiation is only created by charged particles. It’s the electrons scattered by neutrinos that emit the Cerenkov light. That or other charged particles produced though charged-current weak interactions with the electrons or nuclei in the medium.
That was a pretty bad error in the article.
So the charged particles emit the radiation…because of the neutrinos moving through the medium. Thus: “When a neutrino moves faster than the speed of light in a given material, like water, Cherenkov radiation is produced”
Nope. It has to interact first.
In fact, if the neutrino is only travelling *modestly* higher than the speed of light in the medium, it’s *impossible* for it to interact. Neutrinos have to be ridiculously relativistic to even have a chance at interacting because their mass is so low.
Nope. The vast majority of neutrinos passing through the medium are going faster than the speed of light in the medium (because neutrinos basically travel at the speed of light in a vacuum) and they DO NOT cause Cerenkov radiation. The vast majority of neutrinos do not interact in any way.
“in a given material.” Not in vacuum.
However, the article is still wrong because it’s not the *neutrinos* that generate Cherenkov radiation (which is EM radiation) – because neutrinos don’t interact electromagnetically.
Instead, it’s the particle that’s produced *after the neutrino interacts* that produces Cherenkov radiation, because the particle produced is an electron, muon, or tau lepton. And because the neutrinos are such high energy, the energy imparted to the e/mu/tau is way more than enough to produce Cherenkov radiation.
And anyway it’s less impressive but quite correct to say that they just have to have a velocity greater than the phase velocity of the light, where the phase velocity is a different thing from the “usual” velocity and can be faster, slower, negative, etc. It gets less intuitive and consequently less fun to think that way of course. But as a result it seems you can get cerenkov radiation in various ways in metamaterials, including propagating backwards instead of forwards.
As for the phase vs group thing, a visual borrowed from wiki commons:
https://upload.wikimedia.org/wikipedia/commons/b/bd/Wave_group.gif
I did my PhD and postdoctoral work on an experiment that had reasonable neutrino sensitivity. One learns vast amounts of esoteric knowledge, the nuance in the design of pretty much every subsystem in these detectors is staggering.
I think you’d be a great candidate for a Hackaday Hack Chat. Seriously.
The fact that there’s already 3 people commenting on this article that have directly worked in this field (I’m the third) is kinda surprising.
Totally agree with the grandparent poster, though, the amount of random knowledge you need to build kilometer (or hundreds of kilometer!) scale detectors is crazy.
And then, of course, 20 years later you look back on the work you did and it’s like “well, that’d be freaking trivial nowadays.” Blah, technology.
Oh please! Neutrino hack chat! Hit up Dan Maloney @ hackaday.com for the invite.
I did a neutrino mass experiment as my PhD thesis and Art McDonald was my advisor. Do I count too? (I left and went elsewhere instead of doing SNO).
I ran AMANDA and IceCube at South Pole for a few winters, and helped build IceCube for two of those summers. So much physics and electronics coming together in the detectors.
I think you’d be a great candidate for a Hackaday Hack Chat. Seriously.
Second!
“100 trillion neutrinos pass through your body every second. ” Sounds like a lot, and it is.
But for perspective, just sitting indoors in a dark room your body is receiving ten million times *more* thermal photons just from the building around you.
Just imagine if we could harness them for power.
Hold up a sec, I think I have an idea…
The sun makes lots of photos, all the time, and it’s free!
We should figure out a way to harness that energy too!
Thank you Captain Obvious. 😁
100 trillion = 1E14
“10 million times” = 1E7
Total = 1E21
Thermal photon at room temperature: about 1/40th of an eV
1E21 times 1/40th = about 4 joules, so every second… four watts.
For reference *you* radiate more power than that, because, of course… your body’s hotter than room temperature.
“For reference *you* radiate more power than that, because, of course… your body’s hotter than room temperature.”
And thus the Seebeck effect quartz watches:
https://quillandpad.com/2018/08/10/watches-powered-by-body-heat-did-the-bulova-thermatron-foretell-a-matrix-powerwatch-heat-driven-future/
The difference is that the photons are hitting you while the neutrinos pass right through you (and the entire Earth below you). There is a huge amount of neutrinos, but they don’t do anything. They are almost totally invisible. That’s why you have to build such huge detectors to see at least a tiny fraction of them.
“…When a neutrino moves faster than the speed of light…”
Really !
No, not really, because the rest of that sentence matters.
Still, the rest of the sentence is also wrong ;-) Cherenkov radiation works with charged particles only, so the secondary particles created in a neutrino interaction with matter will produce the Cherenkov light, not the neutrinos itself.
There is nothing in the “…rest of that sentence…”, or any following sentence for that matter, which would lead to a contradiction of the Special Theory of Relativity. Proof to the contrary would be deeply appreciated by more than just a few people.
This would be a good place to mention the Long Baseline Neutrino Facility (being built at Fermilab) and the Deep Underground Neutrino Experiment (the target of the LBNF); being built at Lead, South Dakota.
What is the purpose of these experiments? What would we like 5o know about neutrinos? How can we use them?
Clickbait answer: neutrinos are the only stable particle that interacts only via the weak force, and the weak force has broken physics multiple times and directly led to more Nobel Prizes than the study of any other fundamental force in nature.
They are also the only particle that has known non-gravitational interactions outside of the Standard Model, and may be responsible for all life in the Universe.
Twice over.
(Truthfully, nothing that’s written before this is actually terribly exaggerated.)
For anyone interested in neutrinos, back in 1976 there was a song written about them…
“I am someone you’ll never know
I am the little neutrino
And now I’m passing through
The one who’s known as you
And yet you’ll never know I do”
‘Littile Neutrino’ by Klaatu (the band that wasn’t the Beatles)
https://www.youtube.com/watch?v=3dkKcf40YfE
And there is a funny video made by the Italian INFN institute (it has English subtitles):
https://www.youtube.com/watch?v=dhkCMO1lG7g&t=66s
That link didn’t work in my area for some uknown reason so…
https://www.youtube.com/watch?v=pSfCQNW00dk
There is one step missing in the section about Cherenkov radiation. It works with charged particles only. Neutrinos do not have a charge, so if the Neutrino passes through water or any other medium, there won’t be any Cherenkov radiation – otherwise neutrinos would not be elusive at all and our world would be a lot brighter ;-)
What is missing is the part where the neutrino interacts with matter. Even though the cross section of neutrinos is rather small, every once in a while one out of many neutrinos interacts with a nucleon inside the nucleus of the atoms. When this happens, the neutrino is converted into its charged counter-part. Electron-neutrinos will be converted into electrons, muon-neutrinos will be converted into muons, and tau-neutrinos into tauons. While these particles travel of course slower than the speed of light in vacuum, they often are faster than the speed of light in the medium. In this case, Cherenkov is emitted and can be observed by the photo detectors at the walls.
Interestingly, there was once a paper which claimed neutrinos were faster than speed of light in vacuum. The OPERA experiment measured the time neutrinos took to travel from CERN to the Gran Sasso lab in Italy, which is a distance of ~732km. Due to some measurement error, the time was measured a bit wrongly and as a consequence the neutrinos were thought to be faster than light. Because I was doing my PhD at that time at the OPERA experiment (with a different topic) my name is actually on that paper :-D
That paper can be found here:
https://arxiv.org/abs/1109.4897
and you can still find the original (wrong!!!) version here: https://arxiv.org/abs/1109.4897v1
Keep in mind, this is how science works. You do a measurement as carefully as you can, you find a surprising result. Then you check again for yourself if you can find an error, and if you cannot you will start asking the community. Back at that time, there were tons of “papers” posted on arxiv.org which attempted to explain the Physics behind neutrinos being faster than light, but also some trying to explain what the experiment could do wrong. In the end, another re-calibration of the timing system revealed that a lose connector caused some shift of the time measurement – we are talking about 60ns time difference, which needed to be measured over the distance of 732km.
In any case, this effect would have been totally unrelated to Cherenkov radiation, since it only works for charged particles, and only refers to the speed of light in a medium.
And btw: scintillation and Cherenkov radiation are two completely different things, too…
“Keep in mind, this is how science works. You do a measurement as carefully as you can”
Rant time. OPERA was never *designed* to measure the speed of neutrinos. It was a side result. In anything else, you’d call it a systematic error and a curiosity and maybe talk to colleagues at conferences to figure it out. If you can’t, *eventually* you’d turn around and *design* something to figure out if it’s real or not, and *then* you’d hold the press conference.
What do I mean that it wasn’t designed to measure the speed of neutrinos? Just think back to high-school physics: you measure velocity as the slope of a position vs time graph. The *minimum* number of points you need is 2. The minimum number of points you need to have an *error* estimate independent of your error estimates in the original points is 3.
OPERA actually only had 1. They inferred the start time – they didn’t measure it, because there was no neutrino detector at the near end. So they only had the arrival time, and errors on that time. Calling it a velocity measurement with the precision they did was just bizarre.
Reporting this the way they did was just weird. I’ve been part of multiple “weird anomaly” papers, mind you, and none of them were explained as an “oops, my bad” 6 months later.
Plus, keep in mind, as a colleague of mine (John Learned) said, “[a screw-up] is probably in the details not accessible to outsiders.” And it was. “Asking the community” didn’t actually help, because *we couldn’t* help, because they *hadn’t done* enough tests to confirm what they were measuring.
The most damning detail is that there’s another detector (ICARUS) which uses the same neutrino beam. These guys are *colleagues* of OPERA. They metaphorically work down the hall (in some cases literally). It took them a handful of months to do the same experiment, and golly gee, guess what.
There is *zero* reason that OPERA should have released that result without talking to ICARUS first. Now, I have no idea, maybe they did and the ICARUS guys weren’t interested or something (but that’d be a separate failing). It took them no time. They would’ve immediately seen that it was a systematic on their part.
Honestly, I’m ranting a bit on this, but… that OPERA result was *not* actually how science should work. Theoretically, yes, but from a practical perspective, no way.
> and *then* you’d hold the press conference.
You are right, there should not have been a press conference. Some people obviously got too excited. Apart from this, everything was done as it should have been. You are wrong in a couple of points here.
> OPERA was never *designed* to measure the speed of neutrinos.
Even this is not entirely true. OPERA was primarily designed to measure nu_mu -> nu_tau neutrino oscillations, but considering the effort and money put into an experiment of that scale, it is very common and actually expected to try to make use of the experiment as much as possible. Some parts of the detector (the data acquisition system) were actually designed at least to a certain extent with the possibility of such measurement in mind.
> OPERA actually only had 1.
Totally wrong. OPERA had a huge number of data points, several millions I think. The only point is that all measurements were taken with the same distance, but that doesn’t mean you cannot derive any uncertainties. In fact, it would be even more curious if the neutrino speed were changing over the distance of the travel than any observable deviation from the speed of light. Hence it is perfectly ok to keep the distance constant.
> They inferred the start time – they didn’t measure it, because there was no neutrino detector at the near end.
I remember we actually discussed this in my group and also within the collaboration before releasing the false result to the community. We measured precisely the time when the protons hit the target producing the neutrinos (this can be done very precisely with the standard diagnostics of every particle accelerator, nothing special here). It would violate causality if the neutrinos were produced before the protons hit the target. Since we measured a too short time, not a too long time, any special time behaviour in the production process would make the neutrinos only even faster. Also that special time behaviour would be even an equal important discovery. So this point is really no argument against publishing the result. There is nothing bizarre about this.
> The most damning detail is that there’s another detector (ICARUS) which uses the same neutrino beam. These guys are *colleagues* of OPERA. They metaphorically work down the hall (in some cases literally). It took them a handful of months to do the same experiment, and golly gee, guess what.
Well. Now consider the opposite outcome. Just assume for a second the measurement would have been correct and neutrinos were faster than light. Waiting for another experiment to confirm the measurement before formally publishing would mean the other experiment would take the Nobel price. So why should OPERA *not* publish this result?
I think you have a misguided understanding of the meaning of a publication. Releasing the result to the press was wrong, yes. A scientific publication is not a press release, though. Publications mainly address the peers in the scientific community. They are a necessity for another group to repeat the experiment and hence confirm or reject the result. Without publication, no comparison between results is possible, because the publication *is* the result.
Triggering another experiment to redo the measurement was wanted. It was very welcome that ICARUS did that, they actually got even help from OPERA as far as I remember, and certainly they would not have been able to do this so quickly without OPERA doing it first. (I am pretty sure ICARUS took the measurement of the distance from OPERA, because OPERA could measure it more easily when the highway tunnel was closed due to maintenance – at least redoing that part of the measurement would have been extremely difficult in that short period of time.)
Yes, in this particular example the community was unable to pinpoint the problem, but I can tell you (because I read almost all of the replies on arXiv back then) there were quite helpful comments, and some of them even changed the result slightly.
If you make a big discovery, you have to publish it some day. You cannot redo the measurement over and over again and keep it a secret forever. Sorry, I repeat: This is how science should work. Yes, mistakes were done obviously, but that’s normal. The scientific system was able to catch that (without problems). This is a good thing. Lesson learnt: Don’t call out a press conference that early (or at all)!
My rant really falls back on the comment we both agree on: there should not have been a press conference. That’s the entire problem. I don’t fault people for publishing weird results. Heck, I have several weird results published. I have some weird results published that *were* the focus of the experiment, and there’s literally no other explanation for them other than “we just got extremely unlucky.”
If you want a good example of what I mean: there’s a seminal paper on the density of asteroids out there that contains a bunch of density measurements that are obviously complete nonsense (like, 100x lead and stuff like that). They still published it, but there’s a footnote there marking them as “unreliable” or “unphysical.” That’s absolutely the right way to do it.
What I meant by “you only have 1 point” is more fundamental: your model for the propagating neutrinos is just high school: “d = vt”, fixed d, measure t, you’re done, right? But that’s your *model* – that’s what you *believe* you’re measuring – a straight line on a distance vs time graph.
With one location on that graph, you can’t measure your *model* uncertainties – how well you’re *actually* measuring what you think you’re measuring. All of your uncertainties are experimental: statistical and estimated systematic.
“Waiting for another experiment to confirm the measurement before formally publishing would mean the other experiment would take the Nobel price.”
Um. No. First, “if we don’t publish our terrible result quickly we won’t get a Nobel!” is… not a great argument. Second, if OPERA shares the results internally and asks ICARUS to do the measurement and they publish it without referencing OPERA, there’d be a lot of ethics investigations going on.
The problem was found in *months*. Not years. Penzias and Wilson worked for years scraping bird poop before publishing. If someone in OPERA was claiming we have to publish this because we might get scooped for a Nobel, they weren’t evaluating the quality of the measurement properly.
> They still published it, but there’s a footnote there marking them as “unreliable” or “unphysical.” That’s absolutely the right way to do it.
All right. Btw: The first publication was just on arXiv.org and hence does not really count as a true publication. This was before peer-review. Publishing articles at that stage is very common in that area of Physics, because you cannot keep the results a secret anyway for that long and it basically broadens the peer-reviewing group. I consider this a good thing. If that stupid press conference would not have existed, one could say nobody seriously claimed the result was true…
> your model for the propagating neutrinos is just high school
This is not *my* model. This mode is generally accepted and expected. If no force is applied to a particle it will travel at constant velocity. Since neutrinos only interact weakly, there is no force. I repeat: any deviation from this would be even more surprising than travelling faster than light. Also: if the experiment would have measured a longer time, i.e. slower speed than light, it would have been almost equally surprising. You are suggesting to go several steps ahead before doing the first step. This is not what you can do if an experiment costs billions of Euros.
> With one location on that graph, you can’t measure your *model* uncertainties
Are you a theorist (or what is your background anyway)? The model under test in this experiment is so damn simple that we experimentalists do not and should not care about model uncertainties. This is a direct measurement of the neutrino speed. There is no model needed to derive this value from the measurement data. If the result were true, it would be up to the theorists to work on a model which explains the measurement. It is never the other way round!
Btw: Last sentence of the original pre-print: “We deliberately do not attempt any theoretical or phenomenological interpretation of the results.”
> “if we don’t publish our terrible result quickly we won’t get a Nobel!” is… not a great argument.
Yes and no. I took it to the extremes of course. An unpublished result is not a result. This is how scientific communities work.
> Second, if OPERA shares the results internally and asks ICARUS to do the measurement and they publish it without referencing OPERA, there’d be a lot of ethics investigations going on.
Maybe. On the other hand, handing results of that possible magnitude over to another big collaboration is probably equal to publishing it on arXiv.org. It will be a matter of days before the result would have leaked out to the public. That situation would have been even worse, because the only thing spreading would have been ‘neutrinos are faster than light’ without any details. If you put the stupid press conference aside: “publishing” the result on a preprint server like arXiv.org is basically exactly the step you propose. We just did not ask only a single experiment but the entire community.
> The problem was found in *months*. Not years.
Wrong. The problem was found in month after the pre-print. The researchers did investigations internally before that, and yes this was years. Even the majority of the OPERA collaboration (including me) was totally unaware. There was even a PhD thesis on the topic which was (with some special exemption) held back even after the student got her title. They had already redone every step of the measurement after the first surprising result to verify it internally, this was even before the rest of the collaboration was informed. This was heavily criticised by many collaborators because it is somewhat against the idea of a collaboration, but they justified this with the extremely high probability of a leak (170 physicist involved IIRC, of course with a certain fluctuation).
This is a difficult situation. You cannot keep such a result under the radar for long. The only avoidable mistake was calling in a press conference.
“This is not *my* model. This mode is generally accepted and expected. If no force is applied to a particle ”
No, no, that’s not what I’m saying. I’m saying that this is the model you’re thinking *applies to your setup*. You’re thinking “these are the only effects going on here” as opposed to a time offset, some extra delay somewhere, some weird propagation mode, etc.
“Are you a theorist (or what is your background anyway)? The model under test in this experiment is so damn simple that we experimentalists do not and should not care about model uncertainties.”
Ha, wrong way: I’m a *heavy* experimentalist, which is why to me, the response is that you *never* stop thinking about whether you’re measuring what you think you’re measuring. It doesn’t matter how “damn simple” your idea is, the experiment is a *thousand times* more complicated, and you’re just *hoping* that everything reduces to being that simple.
Systematic error measurements has to come from somewhere, and if it’s only coming from your *estimates*, it’s fundamentally unbound. That’s why a second experiment was needed in the first place!
Saying “oh, that measurement was wrong” is actually unfair. It wasn’t a wrong measurement. It’s not like OPERA mixed up the data or something (*that’s* happened too). It had systematics that couldn’t be measured with the way it was done.
Seriously – in one experiment of mine, colleagues were trying to calibrate the gain of an amplifier. It’s an amplifier! You divide the output by the input, there’s your gain! People did detailed analyses and months of work and showed up, plotting “out” vs “X*in” and were like “yeah, there’s this systematic we don’t understand, something must be wrong.”
Except after a long time, you realize – wait, the amplifier’s got a very slight *delay*, and I have to fit for that *delay* too, even though it was tiny. You do that, the problem entirely goes away.
I mean, again, being clear – it was already obvious that if OPERA *was* measuring something real, it was not as simple as “neutrinos travel faster than the speed of light” because there were *already bounds* that would’ve prevented that. Yes, you could stretch things and make it work… but remember, your model is supposed to be “damn simple” right?
“This is a difficult situation. You cannot keep such a result under the radar for long. The only avoidable mistake was calling in a press conference.”
99% right, in my opinion. Personally, I would’ve published the paper just saying “time-of-flight measurement” blah blah, said “our current error estimates appear to suggest that a simple velocity of ‘c’ is disfavored, however, additional measurements are ongoing.”
A lot of people would disagree with weasel-wording like that, but that’s what *I* would do. If someone comes out with a bolder statement in the paper, I wouldn’t rant or anything, just chuckle and be like “yeah, keep workin’ on that.”
The rant portion is solely reserved for the press conference. The rest of the disagreement is just because I disagree about phrasing results you don’t understand as possibly groundbreaking, but that’s a disagreement I lose frequently.
I do enjoy the comments on hackaday articles!
Right!!! I’m sitting here like an excited kid at a tennis match, eyes tracking the ball back and forth, unwilling to make a sound and interrupt this wonderful exchange.
I was a submersible pilot back in the 70’s and participated in a neutrino experiment where we would fabricate and develop photo plates at a depth of 1000 ft. in the ocean off of Grand Bahama island. The experiment took place in the dive chamber of the Johnson Sea-Link submersible and lasted 10 to 14 hours at depth while the plates were being fabricated and developed. The plates were placed in multiple glass spheres and left at depth for 6, 12 and 18 months. We would go back at the appropriate intervals, retrieve the plates and develop the emulsion at depth. I’ve forgotten the name of the physicist but he was able to determine whether the neutrino had passed through the earth or only the 1000 foot seawater column.
“…The first principle is that you must not fool yourself—and you are the easiest person to fool. So, you have to be very careful about that. After you’ve not fooled yourself, it’s easy not to fool other scientists. You just have to be honest in a conventional way after that…
“It’s a kind of scientific integrity, a principle of scientific thought that corresponds to a kind of utter honesty—a kind of leaning over backwards. For example, if you’re doing an experiment, you should report everything that you think might make it invalid…
“In summary, the idea is to try to give all of the information to help others to judge the value of your contribution; not just the information that leads to judgment in one particular direction or another.”
—Richard P. Feynman, 1974 Caltech commencement address
Exactly, let’s stick to fundamentals that are established fact. The neutrino has matured from a dubious birth to a greatly inflated mature existence. Please people, try to find a better explanation.
Ok you all can roast me for being cranky, uneducated or even ignorant, but the whole existence of the neutrino is dubious at the outset.
It was invented by Pauli to have a mechanism to take away the unexplainable excess (read as missing) energy after the event of beta decay
Looking now not much has changed. Supposedly the only way to infer neutrino existence is to assume reverse beta decay (probably not unique to neutrino interaction) is a neutrino detected.
Let’s have a discussion about the reality or lack thereof in the idea of the neutrino. All the hype from very scarce and fringy data from long exposure investigation is entirely dubious.
Thanks
“but the whole existence of the neutrino is dubious at the outset.”
No. The existence of neutrinos is very well proven in many experiments. Most of them do not use the reverse beta decay. In modern experiments we can detect tracks of charged particles. We can make beams of neutrinos, and these beams can be switched on and off. We have created a beam of neutrinos at CERN in Switzerland and placed a detector in a distance 732km in Italy 1400m deep below a mountain, and we could clearly see charged particles created “out of nothing” in the middle of our detector, flying away from CERN and perfectly correlated in time with the beam generated at CERN. We even measured the time of flight of these neutrinos (at first with an error which led to the false claim of neutrinos being faster than light – see my other post above).
How can you explain this without the existence of neutrinos? In particular: Wolfgang Pauli not only postulated that particle just for fun, but due to an unanswered question at that time. He was able to derive several measurable properties of the particle, and all of these properties have been confirmed. You can say, this is still not the neutrino but something else, but than you are just given it a different name.
Ok fair enough, you’re way more educated on this than me. I read all the comments above. That matter was interesting about publishing or not publishing. Because I’m not very well educated I haven’t been able to tell by Google search where the gazillions of neutrinos come from. I thought they only came from beta decay. Also cherenkov radiation described above seems to be from secondary particles like electrons, positrons and muons etc. There seems to be a lot of certainly that nothing else can produce what has been observed. However the chain of detection seems to be several steps removed from the actual detection of a neutrino. I find it astonishing that a couple of dozen germanium atoms from hundreds of tons of gallium can be chemically separated and then have absolute certainly this was not contamination or another decay process at work. Humbly
It’s ok, I won’t blame anyone for lack of knowledge :-) If you want to learn more about particle physics, I recommend Wikipedia rather then Google, most articles there are quite good.
“where the gazillions of neutrinos come from”
Most of them are coming from the sun actually and they are produced in the nuclear fusion. This is somewhat related to the inverse beta decay. Even though the understanding of the processes inside the sun is always an indirect one, it does not mean it has a big uncertainty and is “just a theory”. In fact, all knowledge of things we cannot see with our own eyes is obtained indirectly. Still, if you have a theory (which is a very narrow-defined term in fundamental science and in *no way* something minor and/or opposed to facts) derived from a couple of observations (let’s say the theory is the existence of neutrinos which have a couple of well-defined properties), you can test this theory in experiments. If people are unable to make experiments which contradict the theory, the theory becomes generally accepted.
A theory is always very specific. In case of neutrinos, the neutrino properties are an important part of it. Most importantly there is the lepton number, which is known to be conserved in many experiments. Leptons are e.g. electrons and their heavier “brothers” muons and tauons, which are all equally charged. Without neutrinos, the beta decay would violate the lepton number conservation, which would make the entire theory of particle physics a lot more complicated. So in fact there were many more hints in the direction of the existence of neutrinos than just the beta decay. There are so many pieces of the puzzle fitting together.
In the end, there are many symmetries and conservation laws. That’s why physicists search for even more symmetries, like super symmetry, because it would allow to explain the whole world in a single theory. Right now there are basically two theories, one for small scales (quantum theory) which applies to particles including neutrinos, and one for big scales (general relativity). It’s not like we had many theories which would be independent from each other. All fits together nicely – except these two huge theories. But people are working on it to close even that gap!
“It doesn’t matter how “damn simple” your idea is, the experiment is a *thousand times* more complicated, and you’re just *hoping* that everything reduces to being that simple.”
I don’t understand why you want to make the theoretical part of the experiment even more complicated in that case :-) OPERA was measuring an average speed of the neutrino plus some other particles, but because the neutrinos travelled 732km and the others only a few meters, this is heavily dominated by the neutrino speed. Since every sane theory in the world would predict all particles going damn close to the speed of light at the given energies, the strong expectation (null hypothesis) is to measure the c (speed of light) over the given distance. Any deviation not coming from systematics from that would point to new physics.
For various reasons, it is completely out of the question to quickly build another detector e.g. mid way. At least nobody would grant any money for this unless there is some real hint that this experiment could measure something different from the speed of light. So the goal was to do a best effort measurement, spending a very limited budget. The clear expectation was to simply set a limit, meaning the expected result would be compatible with speed of light but you would have a smaller uncertainty for neutrinos deviating for that.
It is a good practise to try simple measurements first before doing more complex experiments, especially if those cost easily billions of Euros.
PS: The rest is a play of words in my eyes. If I disregard systematic effects which completely change my result, then my result is wrong. Every experimentalist should know that this can always happens and it does, so a single experiment is never ever fully trusted. This is especially true the more surprising the result is. Extraordinary claim requires extraordinary evidence. Don’t blame a single experiment for not delivering extraordinary evidence, because no single experiment can do that by definition.
“I don’t understand why you want to make the theoretical part of the experiment”
This isn’t the theoretical part of the experiment. It’s the experimental side, and it’s the side that almost always gets dropped because building realistic models and determining systematic error is boring and very, very few people do it in sufficient detail.
“So the goal was to do a best effort measurement, spending a very limited budget.”
Yup! I totally agree! And you know what you *don’t* do with a best effort measurement and a very limited budget?
Hold a press conference.
Again, like I said – this is the root of the problem. You just don’t report it this way. You don’t even come out and say “superluminal neutrinos,” you say “inconsistent with current theory, but systematics need to be further studied.” Because it’s *the actual truth*.
Again: see the Feynman quote above, which is actually above my desk. “The first principle is that you must not fool yourself—and you are the easiest person to fool.” If you’re so worried about the result leaking, fine, write it up incomplete but *state* that it’s incomplete and extremely preliminary. Don’t conclude *anything*. Let others do that. Just report the data… and you *absolutely* don’t hold a press conference.
@Pat: I still cannot reply to your posts properly, sorry ;-)
I think we agreed several times now, the press conference was a mistake. My point is, the rest wasn’t fundamentally wrong. The wording in the paper was rather cautious (“anomaly” was the strongest description for the observation). In every measurement you can have unaccounted systematic effects, all scientist must know that and take it into account. Since it can never be excluded, the possibility for unknown systematics cannot be any reason not to publish.
Sorry, somehow this ended up in the wrong thread now…
The threads are messed up now, sorry… :-) I replied to Pat somewhere else by mistake…
Ok, it’s not my mistake. The comment system seems to put everything down here now :-/
Ooo more of this discussion, keep it up guys!!
Wait now wha… Hold’up real quick……neutrinos move faster than the speed of light??? Maaaaan i dunno hackaday….. i aint no fizzycist or no engine knee’r or nothin like that, but somethin just don’t sound right bout that…
Best story ever on hackaday.
Where can I find more threads on particle physics like this, preferably with some of the current greats students gently correcting, elaborating and suggesting directions of thought.
The hardest particle to detect.
The Egyptian pyramids were explored for hidden cavities by neutrino sensors in cavities below.
So I have learned that we can reliable generate neutrinos, I’ll have to do my research on this, but are we able to tune these neutrinos to enhance detection of them, eg giving them the exact energy excess to excite electrons in a phosphor or whatever the equivalent in terms of nuclioili’s (gibberish I know) and thus we could make a worldwide communication network without satellites?