There’s no shortage of ways a satellite in low Earth orbit can fail during the course of its mission. Even in the best case scenario, the craft needs to survive bombardment by cosmic rays and tremendous temperature variations. To have even a chance of surviving the worst, such as a hardware fault or collision with a rogue piece of space garbage, it needs to be designed with robust redundancies which can keep everything running in the face of systemic damage. Of course, before any of that can even happen it will need to survive the wild ride to space; so add high-G loads and intense vibrations to the list of things which can kill your expensive bird.
After all the meticulous engineering and expense involved in putting a satellite into orbit, you might think it would get a hero’s welcome at the end of its mission. But in fact, it’s quite the opposite. The great irony is that after all the time and effort it takes to develop a spacecraft capable of surviving the rigors of spaceflight, in the end, its operators will more than likely command the craft to destroy itself by dipping its orbit down into the Earth’s atmosphere. The final act of a properly designed satellite will likely be to commit itself to the same fiery fate it had spent years or even decades avoiding.
You might be wondering how engineers design a spacecraft that is simultaneously robust enough to survive years in the space environment while at the same time remaining just fragile enough that it completely burns up during reentry. Up until fairly recently, the simple answer is that it wasn’t really something that was taken into account. But with falling launch prices promising to make space a lot busier in the next few years, the race is on to develop new technologies which will help make sure that a satellite is only intact for as long as it needs to be.
The Sky is Falling
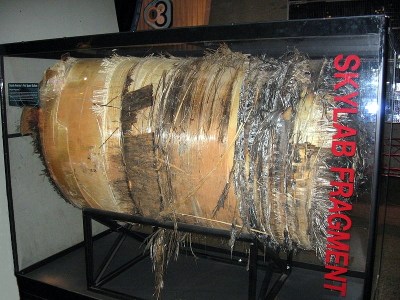
The possibility of debris surviving reentry and hitting the ground is nothing new, and there are documented cases going back for about as long as we’ve been shooting things into space. Arguably the most famous example was in 1979, when wreckage from Skylab was strewn all over the Australian outback. The largest pieces, such as the tanks that held oxygen aboard America’s most persnickety space station, tipped the scales at hundreds of kilograms. Luckily these massive objects came down in one of the most scarcely populated stretches of land on the planet, but had they come down in a major city the damage and risk to human life could have been considerable.
What’s changed is how many objects we can expect to be reentering the atmosphere in the near future. When only the world superpowers had the ability to put something into space, it was relatively easy to keep track of when things were coming back down. But increased competition has dramatically reduced the cost of putting satellites into orbit, and now companies are looking at space investments which would have been simply impossible just a decade ago. Companies like SpaceX, OneWeb, and Samsung are eyeing satellite mega-constellations consisting of thousands of individual spacecraft, and each one of them will eventually come careening back down through the atmosphere at the end of its nominal lifespan.
In a letter dated February 26th, the Federal Communication Commission specifically asked SpaceX to detail their plans for deorbiting the thousands of satellites in their proposed Starlink network. They wanted to know if SpaceX can ensure that the craft will reenter over the ocean, and if not, to estimate the likelihood that falling debris could cause material damage or human casualties. The letter ends by stating that SpaceX’s application for Starlink approval could be dismissed if this information was not provided to the satisfaction of the FCC.
Unacceptable Risk
Directing a spacecraft to renter over the ocean has always been the standard way of ensuring that any debris that survive won’t cause any problems on the ground. Since most of the planet’s surface area is ocean anyway, this ends up being a relatively simple thing to do assuming your spacecraft is functioning normally and is capable of maneuvering itself. Though mistakes do happen; Skylab was actually supposed to reenter south of Africa, a slight error in the calculations caused it to overshoot considerably eastwards.
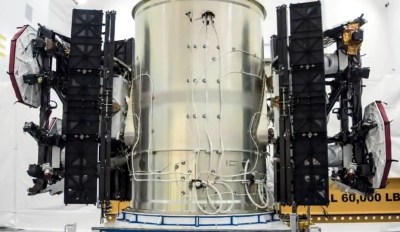
But SpaceX’s proposed Starlink satellites (and others like it) are something of a special case. They utilize high efficiency Hall-effect propulsion which is ideal for occasional orbit adjustments and station-keeping, but lacks the thrust necessary to put the craft on a targeted reentry trajectory.
In other words, the satellites are capable of lowering their orbit enough to ensure they will burn up, but can’t pinpoint where it will happen with sufficient accuracy to make any guarantees. That might be an acceptable risk when dealing with just a single satellite, but long-term Starlink plans call for as many as twelve thousand of them. With that many craft in play, the chances that one of them could break up over a populated area is simply too high.
In their official response to the FCC, SpaceX explained that while they were unable to guarantee that Starlink satellites would only reenter the atmosphere over the ocean, they had come up with a solution which would make it unnecessary. After “extensive research and investment”, SpaceX says they’ve refined the design of their Starlink satellites to ensure they’ll completely burn up in the atmosphere.
Design for Demise
The idea of designing satellites in such a way that they will burn up entirely during a normal reentry has been floating around for decades, but so far has struggled to catch on. Referred to as “Design for Demise” or D4D in the industry, its biggest proponent has arguably been the European Space Agency which has made it a central theme in their overall “Clean Space” initiative. As you might imagine it’s a complex subject, but at the risk of oversimplifying things, there are essentially two main approaches: designs which break up into smaller pieces upon entering the atmosphere, and the use of materials which cannot survive the intense heat of reentry. These two ideas are not mutually exclusive, and indeed would likely be used together for best results.
For example, rather than holding a satellite together with nuts and bolts, it could be glued together with epoxies which are formulated to break down at high temperatures. Once the spacecraft hits the atmosphere and starts warming up, the epoxy gives way and the structure simply falls apart. Not only does this technique reduce the number of large objects that could conceivably come through the atmosphere intact, but it also opens up the interior of the spacecraft to the flow of hot atmospheric gasses which will promote more complete burning.
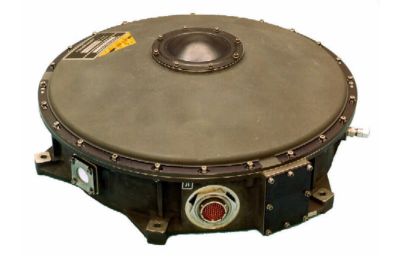
Even still, there are some components of the average satellite which are robust enough that they could conceivably survive. Likely candidates are titanium propellant tanks, silicon carbide mirrors (used in optical telescopes or laser communications systems), the iron cores of the Hall-effect thrusters, and the heavy stainless steel reaction wheels used to provide attitude control for the spacecraft. These components pose a considerably trickier problem, as the materials used in their construction were obviously selected for a reason. As an example, replacing a stainless steel reaction wheel with an aluminum one of the same diameter simply wouldn’t work due to the differences in density. The whole system would need to be redesigned to take into account the material change.
According to their official response to the FCC, SpaceX says they’ve replaced these problem components with versions which they can guarantee won’t survive reentry. If true, it would be a huge milestone for D4D design, and likely the yardstick by which future large-scale satellite constellations will be measured. But not everyone is convinced that SpaceX has solved this complex problem as neatly as they say. In a statement to IEEE Spectrum, professor of mechanical and aerospace engineering at the University at Buffalo John Crassidis said he believes the claims are a bit too bold: “Anything can come through the atmosphere if you hit at just the right angle. If they’re guaranteeing it’s not going to cause an issue, then I’m going to have call BS on it.”
“But with falling launch prices promising to make space a lot busier in the next few years, the race is on to develop new technologies which will help make sure that a satellite is only intact for as long as it needs to be.”
Manufacturers should be good at this. Planned obsolescence and all that.
“For example, rather than holding a satellite together with nuts and bolts, it could be glued together with epoxies which are formulated to break down at high temperatures.”
iPhones in spaaaace!
Just make it out of PLA
Never happen, they like them to work properly for some period before they crash and burn.
Samsung Note 7 then. They can auto-destruct and start a fire.
“Skylab is falling!”
I was 11 years old.
From time to time in late 1979 I would watch the space radar track Skylab as it passed through our field of view.
The local news let us know when it made one of the last passes over NE Pa. I was sad the shuttle was to late to prevent it from deorbiting
Since an ASAT projectile imparts some of its own velocity to the target, could a high-lofted projectile come _down_ on a satellite to force the debris into a downward-angled trajectory?
I’m picturing a Starlink satellite which has slowly lowered its orbit to the point that it’s gently grazing the upper reaches of the atmosphere, so even any stray bits would still decay fairly rapidly, even if not over the ocean below the impact point. But the vast majority of the debris cloud would be streaking downward and entering immediately.
Striking the satellite in the retrograde direction (i.e. to slow it down in its orbit) would be optimal for causing quick reentry, but it’s difficult for a missile to do that unless it’s nearly capable of reaching orbit itself, which would make it impractically big. Striking the satellite downward has some beneficial effect, and is what India did in their antisatellite test a few years ago, to try to minimize the debris hazard (though not enough that other space agencies didn’t complain). But using so many antisatellite missiles (one per satellite?) sounds expensive. If you just want to break up the satellite once it’s already skimming the atmosphere, so that the smaller bits burn up more quickly, and you can’t easily build it from weak materials as discussed in the article, why not just build a bomb into it?
>it could be glued together with epoxies which are formulated to break down at high temperatures.
Atomic oxygen in LEO can reactive with epoxy and other composites. They’ll need to be coated.
https://digitalcommons.usu.edu/cgi/viewcontent.cgi?article=2354&context=smallsat
The temperature at reentry should be high enough to melt/burn some of the regular light weighted metals/alloys.
Whether or not an object burns in the atmosphere has to do with its ballistic coefficient.
You drop a ton of feathers down from orbit, and they would probably float down unharmed, because the surface area to mass is just so great that they burn that kinetic energy off very gradually.
But if you drop them down in a big lump, that’s going to create a bow shock that lets the lump fall deep into the atmosphere where it suddenly experiences high resistance and explodes, at which point the individual feathers will be incinerated.
That means, if you break down the satellite too early, the smaller bits may slow down enough that they survive through re-entry and then you got partially molten nuts and bolts raining on people’s heads.
Whatever happened to good, old-fashioned explosive bolts?
The problem is they require the spacecraft be operating normally or you at least have control over it. An ideal system should be completely passive, so even if the satellite “dies” (hardly a rare occurrence), it will still fall apart at the proper time.
The entry of the satellite into the atmosphere could set off the bolts from heat, if you use the right type of explosive.
That would be neat, although I would worry about detonation on atmospheric exit.
The satellite is protected during launch anyway.
The energy density of anything entering atmosphere from orbit is around 30 MJ/kg, seven times the energy in TNT. An explosive bolt will be a damp squib in comparison to the fury of reentry.
And besides, I imagine the discussion around them in the engineering FMEA meeting would be very short.
Put a can of high expanding urethane foam inside the satellite. The last command as it’s coming down is popping the valve on the can. The foam flows out, expanding a huge amount, popping the pieces of the satellite apart, just like it can warp door jambs and pop drywall loose.
A thousand tons of meteors rain on Earth every day. Sure, some hit the ground. Once in a while an odd one causes damage, like Chicxulub, Tunguska, Chelyabinsk, and even Kamchatka just last month. But even that last one that you probably didn’t even hear about was a few thousand tons, and released ten times the energy of the Hiroshima bomb. Virtually all of it you never notice.
It’s absolutely impossible for humanity to rain even a tiny tiny fraction of that much stuff down from orbit.
Sure, it’s responsible not to leave your garbage laying around in orbit where someone might drive into it, but it’s a little weird to get yer undies in a twist worrying whether it’s going to fall on someone’s head when you cause it to make the great high dive. The chance it it doing any damage is vanishingly tiny.
Lloyds would laugh all the way to the bank if they could underwrite it.
The stuff that rains down on earth is mostly tiny particles smaller than grains of rice, but more importantly, it’s less dense than what parts you find in satellites. A steel bolt has a higher ballistic coefficient than a piece of rock the same mass, because the steel is more dense, so it survives deeper into the atmosphere.
And since most of the material coming in at the earth is coming in at such ridiculous speeds, it’s very unlikely to survive the atmospheric entry – any object of any significant mass and size will simply explode about 10-20 km from the surface if it gets that far.
Meanwhile, a satellite with its slower re-entry speed, and shallower re-entry angle, can more easily burn off kinetic energy without vaporizing, and it’s much more likely to actually reach the ground.
I hope that they make SpaceX prove it in a test chamber on Earth before they let them launch.
I would very much like to see a test chamber capable of reproducing the reentry flight regime.
I can answer that: MEDIASE setup installed at the solar furnace in Odeillo in France mimics reentry (disclaimer: I work there…). We have also reproduced Mars reentry or near sun conditions (NASA Parker Solar probe).
See for example https://conference.sdo.esoc.esa.int/proceedings/sdc7/paper/360
The setup looks like that: https://www.promes.cnrs.fr/index.php?page=mediase-fr (a vacuum chamber with stuff around, not very sexy) and google “solar furnace odeillo” for the furnace itself.
Such tests are also done at smaller scale at other research solar furnaces: DLR at Köln for Germany, DoD at White Sand for the USA, CIEMAT-PSA at Almeria for Spain… and USSR built and used the Tachkent solar furnace in Ouzbekistan notably for this (not anymore today AFAIK).
Back to the current topic: if blasted to small parts as described above, some materials build an oxydation layer that protect them during the reentry (think alumina at the surface of aluminium that stops its corrosion in normal conditions, and similar for silver). Of course, depending on the heat level and its duration, this layer may be too weak or building/being replaced not fast enough, leading to the destruction of the component.
As I remember, titanium burns in most “usual” re-entry conditions (understand usual deorbiting angles and civilian reentry rate, not nukes warhead, much faster), but for example some stainless steels build an oxidation layer that require a specific range of angle & speed (i.e. temperature and heat) to be destroyed.
The “usual” heat resistance behavior doesn’t apply here as we have a dynamic effect with varying condition: different temperature, heat levels, atmosphere chemistry over time. That’s why our lab and other are doing as real as possible tests to characterize this and improve the design and the reentry strategies based on component materials, shape, weight, coatings…
And of course, a satellite is made of many parts, and all *should* be burnt: nobody is going to sue a meteorit, which won’t be the same if the damage/injury is caused by human tech…. $$$$$$
Excellent information. Thanks very much.
Odeillo is a little off the beaten path, but next time I’m in the region I’m going to make an effort to visit.
I still think it amusing a solar furnace is located in the Pyrenees (fire mountains…).
I can answer that: MEDIASE setup installed at the solar furnace in Odeillo in France mimics reentry (disclaimer: I work there…). We have also reproduced Mars reentry or near sun conditions (NASA Parker Solar probe).
See for example https://conference.sdo.esoc.esa.int/proceedings/sdc7/paper/360
The setup looks like that: https://www.promes.cnrs.fr/index.php?page=mediase-fr (a vacuum chamber with stuff around, not very sexy) and google “solar furnace odeillo” for the furnace itself.
How it works (shortly): we use concentrated solar energy to reproduce the correct heat and plasma in a vacuum chamber.
Such tests are also done at smaller scale at other research solar furnaces: DLR at Köln for Germany, DoD at White Sand for the USA, CIEMAT-PSA at Almeria for Spain… and USSR built and used the Tachkent solar furnace in Ouzbekistan notably for this (not anymore today AFAIK).
Back to the current topic: if blasted to small parts as described above, some materials build an oxydation layer that protect them during the reentry (think alumina at the surface of aluminium that stops its corrosion in normal conditions, and similar for silver). Of course, depending on the heat level and its duration, this layer may be too weak or building/being replaced not fast enough, leading to the destruction of the component.
As I remember, titanium burns in most “usual” re-entry conditions (understand usual deorbiting angles and civilian reentry rate, not nukes), but for example some stainless steels build an oxidation layer that require a specific range of angle & speed (i.e. temperature and heat) to be destroyed. The “usual” heat resistance behavior doesn’t apply here as we have a dynamic effect with varying condition: different temperature, heat levels, atmosphere chemistry over time. That’s why our lab and other are doing as real as possible tests to characterize this and improve the design and the reentry strategies based on component materials, shape, weight, coatings…
And of course, a satellite is made of many parts, and all *should* be burnt: nobody is going to sue a meteorit, which won’t be the same if the damage/injury is caused by human tech…. $$$$$$
NASA never paid the $400 littering fine either
http://mentalfloss.com/article/70708/nasas-unpaid-400-littering-ticket-skylab-debris-australia
Send them to the moon or park them in a Lagrange position, Might be handy to have a scrapyard for future missions, ya know, just in case.
Wow, just occurred to me why my garage looks the way it does.
LEO satellites don’t have the energy or propellant reserves to do anything but deorbit. Conversely, satellites in higher orbits (e.g. geostationary) don’t have the propellant reserves to deorbit; instead they move to a slightly higher parking orbit and stay up there for a very long time.
I’m surprised nobody has mentioned using a Magnesium Alloy for structural components, it is light and would burn up very well when exposed to reentry conditions (depending on the alloy).
I could imagine that the reaction wheels could be made of a carbon composite belt which binds together a ring of many individual sector shaped weights. The weights do not need to be of a high strength material and they are comparatively small pieces.
During reentry the carbon composite burns up, the the thing flies apart and the weight pieces also can burn up.
Further one, it may be possible to spin them up to max. rpm before or during the reentry to maximize the internal stress and ensure the most complete disintegration.
Is uhh, that a picture of Beavis? … As an adult? … Working at NASA?
I was wondering the same thing!! Beavis working at NASA… Good for him I guess? (Jealous not jealous).