Humanity has been wondering about whether life exists beyond our little backwater planet for so long that we’ve developed a kind of cultural bias as to how the answer to this central question will be revealed. Most of us probably imagine that NASA or some other space agency will schedule a press conference, an assembled panel of scientific luminaries will announce the findings, and newspapers around the world will blare “WE ARE NOT ALONE!” headlines. We’ve all seen that movie before, so that’s the way it has to be, right?
Probably not. Short of an improbable event like an alien spacecraft landing while a Google Street View car was driving by or receiving an unambiguously intelligent radio message from the stars, the conclusion that life exists now or once did outside our particular gravity well is likely to be reached in a piecewise process, an accretion of evidence built up over a long time until on balance, the only reasonable conclusion is that we are not alone. And that’s exactly what the announcement at the end of last year that the Mars rover Perseverance had discovered evidence of organic molecules in the rocks of Jezero crater was — another piece of the puzzle, and another step toward answering the fundamental question of the uniqueness of life.
Discovering organic molecules on Mars is far from proof that life once existed there. But it’s a step on the way, as well as a great excuse to look into the scientific principles and engineering of the instruments that made this discovery possible — the whimsically named SHERLOC and WATSON.
Would You Like Some CHNOPS with That?
Defining what exactly constitutes biological life is difficult, and there are plenty of philosophical arguments that muddy the waters even when you reduce life to characteristics such as the transformation of energy or the ability to reproduce. But at the end of the day, such macroscale characteristics don’t help much when looking for microscopic life on other planets — especially when you suspect that you’re just looking for the remains of ancient microbial life, as is likely the case on Mars.
To explore the possibility that Mars once harbored life, the Mars 2020 mission’s Perseverance rover science payload includes a range of instruments designed to search for the smallest remains of past life. Chief among these instruments is SHERLOC, for “Scanning Habitable Environments with Raman and Luminescence for Organics and Chemicals” — a somewhat forced but impressively descriptive acronym.
At the heart of SHERLOC, which rides at the end of the rover’s two-meter robotic arm, is an ultraviolet laser Raman spectrometer, designed to identify the specific signatures of the so-called CHNOPS elements — carbon, hydrogen, nitrogen, oxygen, phosphorus, and sulfur. Something like 98% of the biomass on Earth is composed of these six elements; finding them on Mars will be pretty good evidence that life once existed there. But simply finding the CHNOPS elements doesn’t make a sample biologically relevant. It’s how those elements are organized and the structures they form that determine whether a sample might have the remains of ancient life, and figuring that out is what Raman spectroscopy is really good at.
Scattering Two Ways
Raman spectroscopy takes advantage of what’s known as inelastic scattering, or Raman scattering. Normally, electromagnetic waves interact with particles of matter by elastic, or Rayleigh, scattering. When incoming photons interact with molecules, they excite them from the ground state to a higher-energy virtual state. In Rayleigh scattering, the excited state quickly collapses and the particle returns to the ground state without any loss of the kinetic energy the incident photon had. It’s like a moving billiard ball that transfers all its kinetic energy into a motionless ball, which then goes on to move while the first ball stops dead.
But about one out of every 100 million scatterings results in dropping from the excited virtual state to a state different from where the molecule started. To stretch the earlier analogy, this would be like the moving billiard ball hitting a motionless ball with a crack in it. The cracked ball would still absorb the energy of the incoming ball, but the crack would attenuate some of it, sending the ball off at a different speed than the incoming ball, and perhaps even in a different direction than would occur in a purely elastic collision.
Just as the difference in speed and direction could reveal information about the characteristics of the cracked ball, so too can Raman scattering be used to probe the structure of a molecule. The difference in energy between the incident photons and the scattered photons depends on the vibrational and rotation states of the chemical bonds within the molecule. This results in a population of photons with different wavelengths that represent the different chemical bonds within a molecule. When spread out onto a detector with a diffraction grating, these photons create a fingerprint that’s characteristic of the molecules in the sample.
While Raman has been used for decades on Earth to analyze all sorts of chemical samples, SHERLOC is the first time the technique has been used on another world. And as you’d imagine, it takes some special engineering to package up all the optics and electronics and make it not only robust enough to survive the rigors of space travel, but also to operate autonomously.
Built to Perform
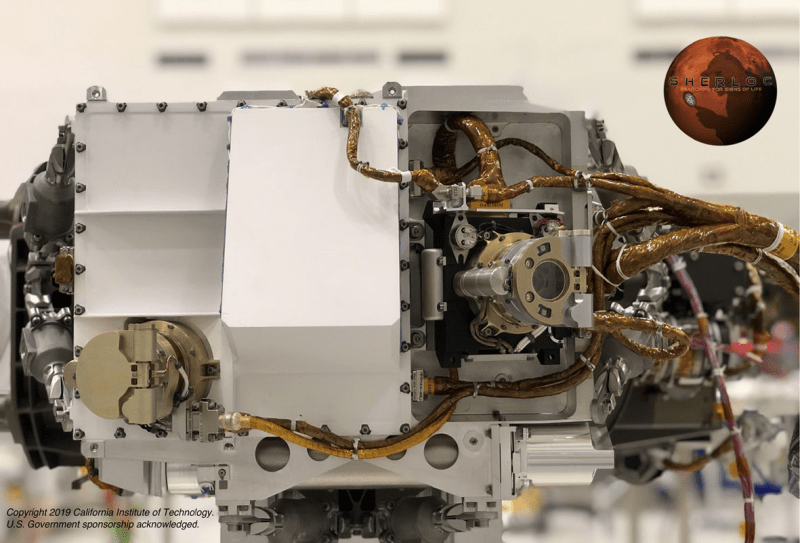
To accomplish all this, SHERLOC is divided into two major assemblies: the SHERLOC Body Assembly (SBA) and the SHERLOC Turret Assembly (STA). The STB is where all the command and data handling circuits are located, and where the power supply lives. The STA is the business end of SHERLOC, and lives at the end of Perseverance’s robotic arm. The heart of the STA is the deep-UV (DUV) laser, a heavily modified off-the-shelf neon-copper metal-vapor laser. It provides a highly stable 248.60 nm pulse and is expected to last long enough to deliver 3 million spectra, which is about seven times the design life of the rover.
As with any Raman spectroscope, the optics of SHERLOC are a complicated set of lenses, mirrors, beam splitters, and filters. Unlike most of its Earth-bound cousins, though, SHERLOC has to handle the “S” in its name: scanning. Rather than rely on fine control of the robotic arm to position its beam, SHERLOC has a scanner subsystem that’s quite similar to the galvanometers used for beam steering in laser shows. The scanner gives SHERLOC control of the beam over a 7 mm x 7 mm sample area with a step size of less than a micron in both dimensions, allowing it to gather data from the smallest of features without having to rely on robot arm moves.
Another way in which SHERLOC differs from other Raman instruments is in the need to correlate spectra with spatial information about a sample. It’s not enough to get the spectral fingerprint of a particular section of a sample; rather, SHERLOC must also determine the context of what that exact spot on the sample looks like in visible light. To accomplish this, SHERLOC requires the help of two cameras: the Autofocus and Context Imager (ACI), a high-resolution grayscale camera that shares the optical path of the Raman spectroscope, and WATSON, the Wide Angle Topographic Sensor for Operations and eNgineering camera. WATSON is a separate, full-color, high-resolution camera with a macro capability down to 1.78 cm focal length. WATSON and the ACI together are basically the equivalent of a geologist’s hand lens, allowing SHERLOC to overlay visible light images with Raman data over a wide range of operating distances.
Finally, SHERLOC’s Raman spectroscope is designed to survive the long trip to Mars, the high-energy landing, and the harsh conditions of the cold, dusty world. While the SBA is nestled safely inside the hull of Perseverance, the STA has to be exposed to the elements to do its job. SHERLOC is mounted on a hexapod arrangement of spring-loaded struts that dampen vibrations encountered both during spaceflight and rover operations. The STA is also equipped with a complex thermal management system, including survival heating elements that keep the electronics and optics warm enough to survive the worst-case Martian cold.
Context is Key
While most of the public’s attention to the Mars 2020 mission so far has understandably been drawn to the wildly successful Ingenuity helicopter, SHERLOC has been busily gathering data pretty much non-stop since Perseverance arrived on Mars back in March of 2021. The confirmation of organics in Jezero crater came from a series of samples analyzed back in September of 2021, and one rock in particular, which was dubbed “Garde.” The rover’s arm-mounted tool assembly was used to grind away some of the weathered rock before SHERLOC was swung into place to analyze the sample.
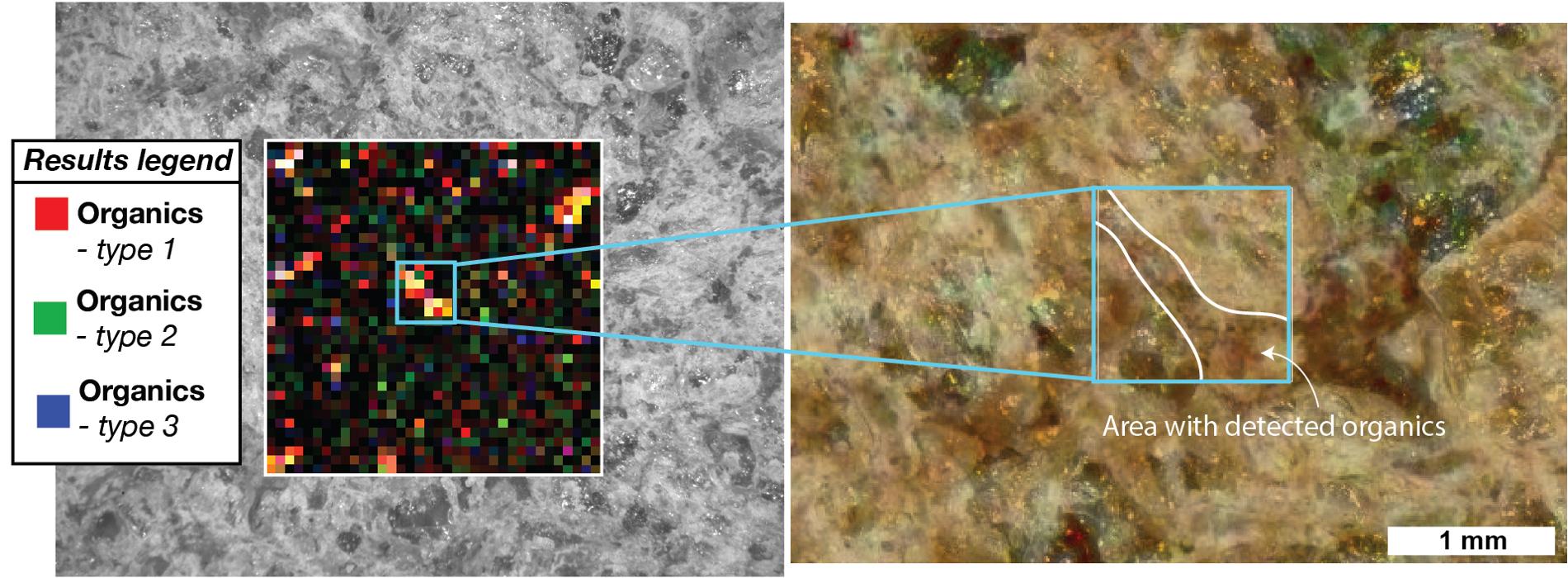
Thanks to the power of SHERLOC and its ability to overlay visible light images with Raman data, planetary scientists were able to determine that Garde contains both olivine minerals, which indicate an igneous history, and carbonate minerals, which suggest a past period of water reacting with the rock. This is consistent with what we already know about the Jezero crater and the river delta that once flowed into it. Finding organic materials in a rock with that kind of geological history is a tantalizing bit of data, and may someday prove to be part of the evidence that life once teemed on Mars.
You can also pick up chlorophyll directly with this. https://www.youtube.com/watch?v=x-xffa_ewnU
A few questions. Why. I mean why does NASA put so much stress on finding signs of life? Do people really care that much? Is there a big space-lobby of molecular biologists? And what happens if you find life on Mars? Do you try to sequester Mars so as not to interfere through some concept of destiny, or do you sequester Mars out of fear of the life form? What if there is microscopic life that is incredibly dangerous. Do you find a way to sterilize the planet?
You find life and declare Mars off-limits until China puts missiles in Mars orbit and claims the planet. Any decision about how to deal with Mars will be meaningless given the number of nations with space flight capability, won’t it?
Yes, I understand the why of exploration. It is the public face of NASA putting the life question front and center that puzzles me. Personally I find the instrumentation built for these missions by the life-forms on Earth to be much more fascinating than finding organic molecules, which are everywhere you point a telescope (To quote Rick Sanchez “There’s literally everything in space Morty!”). And I think Mars with its 1/3g gravity will be really great for big retirement domes.
“What if there is microscopic life that is incredibly dangerous. Do you find a way to sterilize the planet?”
Just to address this part, I’ve always found the idea that there could be “dangerous” life-forms clinging to survival on a barely-active world a bit hilarious. If there was incredibly dangerous life on Mars, Mars wouldn’t be barely active.
*We’re* the dangerous life. It’s far, far more likely that Earth life eradicates life on Mars than vice versa.
Says the person during a global virus pandemic. Eating a peck of dirt may kill you if that dirt contains bacteria your immune system has never encountered. Likewise, if you introduce another micro-organism that hasn’t been around before, the others won’t know what to do with it and it goes like cane toads in Australia.
“Says the person during a global virus pandemic.”
Viruses literally exist because life is so ridiculously prolific and energetic on Earth that sticking a fragile molecule in a tiny bag and hoping a dumb organism comes along is a good reproductive mechanism here. Literally less than a day out of a host and the virus that’s causing this pandemic is a bunch of random fragments.
If life does exist on Mars, it’s in tiny pockets and the overall genetic diversity’s tiny, because they never have to face anything new. Hell, they’re barely doing anything! Earth life would destroy it in a heartbeat.
All your examples are of people encountering other *Earth* life and introducing Earth life to other areas. This is not the same. If there is still life on Mars, energetically, it’s terrible. How do I know? Because Mars is *old*. Nothing changes on it. Everything about Earth is new, all the time, because everything’s being ripped up and regenerated constantly.
Life on Earth has millions of different ways to rip apart things for raw materials because there’s tons of energy and other life around. Fragile isolated ecosystems don’t have that, because there’s no need to.
If there are bacteria on Mars, there are sure to be viruses. It’s part of the evolutionary pathway.
But that wasn’t even the point. You take an organism from Mars and put it on earth, and you don’t know what will happen. The cane toads for example are poisonous and the local animals don’t know to avoid them, so they die attempting to eat the toads. They’re not even particularly prolific – they just lack natural predators to control the population. Same thing here: suppose a Mars bacterium has evolved to exhale hydrazine – it’ll kill everything in its path without even trying.
“Virus” is an overbroad term. If you mean just any stray genetic information which uses a host to reproduce, sure. But the super-fragile, rapidly mutating bits that cause problems with humans? No, those do not have to exist. Extremophile viruses, for instance, are very different, and they’re non-pathogenic. They don’t cause problems in humans at all because the ecosystem is very different. In some sense, viruses attack ecosystems more than they do individuals.
“The cane toads for example are poisonous and the local animals”
Again, you’re using Earth analogs. The environment for the two creatures are nearly identical. The cane toads can survive happily in the same environment as the local animals, and of course the local animals attempt to use the cane toads.
“Same thing here: suppose a Mars bacterium has evolved to exhale hydrazine”
The point isn’t what a Mars bacterium does. It’s what it needs to survive. There’s no way a Mars bacterium could survive in an Earth environment, so it won’t be able to proliferate here. It can’t. It won’t have any of the metabolic pathways to survive efficiently on Earth. It’d get beaten out for resources in a heartbeat.
I’m not saying that niche life in struggling ecosystems can’t be dangerous to people. Extremophile bacteria are pathogenic in humans, for instance. But they’re not dangerous to *ecosystems*. They’re too highly specialized to resource constrained environments. Almost any ecosystem on Mars would have to be *incredibly* slow, because the resources available are minimal, and because we just don’t see any changes whatsoever.
No, of course we don’t know what life on Mars could be like, but we do know what it can’t be like.
>Again, you’re using Earth analogs.
Sure – why not? You’re making an unwarranted assumption that the organisms on Mars couldn’t find much nicer environments. That’s the entire point: you don’t know that the Mars bacterium won’t survive on Earth.
“You’re making an unwarranted assumption that the organisms on Mars couldn’t find much nicer environments. ”
Unwarranted?? Have you *seen* Mars?
Earth changes constantly. The biosphere cycles carbon, water, nitrogen, oxygen, sulfur, iron, phosphorus, and God knows what else (and hey look, those are, again, the most common reactive elements). A huge portion of our atmosphere is only there because of life. Energy’s everywhere. Earth’s biosphere has genetic coding information for practically any molecule you can imagine. Any alien who got even a glance at our planet would be like “yeah, that place is a death trap.”
Mars is static. Nothing changes. The atmosphere’s nonexistent, so there’s nothing cycling through it. The best you could hope for would be a pocket of subsurface liquid, but the resources for that would be extremely limited and the only active life that could be there would be extremely specialized and slow. There aren’t enough different environments on Mars for a huge range of available proteins.
Biology isn’t magic. They have to use energy, and Mars has none. Evolution has to have a reason to develop proteins. Mars has none. It’s not unwarranted to believe that any potential organisms on Mars aren’t adaptable. If they *were*, the planet wouldn’t look the way it does!
If Mars was like, *recently* dead, I’d say sure, there might be a worry you could find, I dunno, some spore or something. But my God, we’re talking about *three billion years* ago. Just not going to happen. The amount of protective biology that you’d have to develop in order to survive that long just is not going to happen by accident. It’s doubtful we could do it technologically!
On Earth we have to protect the hell out of resource-constrained ecosystems because introducing anything from outside can completely destroy it. Mars isn’t dangerous to us. We’re dangerous to it.
The difficulty in this conversation is that of course I can’t prove this. You can’t prove something *doesn’t* exist. And of course people *worry* about this stuff – they quarantined astronauts from the freaking Moon, for crying out loud. But it’s a question of statistics. The chance that something on Mars that’s dangerous is active is beyond miniscule.
Viruses exist because Earth is so teeming with life that putting an unstable chemical in a tiny bag and tossing it randomly is a viable reproductive strategy. The only reason we *have* a global virus pandemic is we’ve got so much migration around the planet. That virus that’s causing the problem? Shove it outside for a day and it’s shredded.
You’re comparing the effect of unknown Earth life on you to unknown Mars life, and that doesn’t make sense. Earth life’s ridiculously evolved. It has millions of ways of ripping down any random thing for resources because there’s so much energy available. Earth is constantly changing because life is always shredding it in new and different ways.
Mars… isn’t. Its atmosphere, geology, and hydrology, from a global perspective, are basically static. Even if there’s a pocket of life somewhere, Earth life would rip it to shreds in an Earth environment because it’s far, far more diverse.
Am I wrong or isn’t it because Mars lacks Earth’s magnetosphere that any kind of Earth like organism would quickly die there of lethal radiation? I think that’s what i read in a Scientific American article-and that’s why Nasa-and perhaps eco-idiot Musk as well-are setting their sites on one of Jupiter’s moons.
Not quickly. Quicker than on Earth, sure. But you don’t need a lot of ground cover to cut that radiation down. So below the surface you could possibly find something. Except in that case you’re cutting down your resources dramatically. I can’t imagine a stable biosphere over hundreds of millions of years confined to a small part of a planet. It’d be like living on a leaky spaceship.
Generally though people usually talk about finding evidence of past life on Mars.
Same argument on Europa, though. Even with a subsurface ocean it’s just not an active enough area in my opinion. But that’s certainly more a fuzzy opinion than Mars.
I was imaging something that is quite different from our life. Maybe more like nano-replicators as pictured by a number of fiction writers. Maybe they are the reason all the iron on mars is oxidized. They find Earth life useful for energy production and it into goo. Of course it likes the metals in your ship as well.
But seriously, an enzyme or the end of a structure that looks electro-chemically like an enzyme that splits hydrocarbons (carbohydrates) of all sorts and builds copies somehow could be pretty bad. And our circulatory systems do a great job of spreading such things to every cell.
Often the NASA and bio types will point to Earth life from hydrothermal vents or hot springs and other unusual places as evidence we can find life on planets with those environments. I have a beef with them because they know their argument is false. All we know is that once you have life like we have, it can adapt to those extremes. The likelihood of it originating in boiling water or acid pools, etc. is basically (Ha!) nil.
The joke, and it may even have come from Hackaday, is that Saturn’s moon Titan is probably the largest proven reserve of natural gas we’ve ever encountered. All we have to do is get it declared a terrorist regime, invade it, and take the gas for ourselves. The incomprehensibly massive advances in science and engineering required to accomplish are merely a necessary evil.
And boom – full funding. :-)
So I’m thinking this means Mars must be fairly crawling with coal just below the surface. Maybe even diamonds. We’ve got to get the stuff back before ${COUNTRY} does… work with me here. We absolutely cannot grin like idiots when we say this.
(also vis. automatic sarcasm machine a few articles newer than this one)
> get the stuff back
I’ve always wondered how much mass could be brought back to earth until the increased mass would reduce the orbital distance to the sun enough and raise the global temperature by 1°C (1.8°F).
Mass of earth: 5.972 × 10^24 kg
Mass of sun: 1.989 × 10^30 kg
Average distance from earth to sun: 1.4732 x 10^11 m
And then I guess I would need to use the two formula from these pages.
https://en.wikipedia.org/wiki/Effective_temperature
https://en.wikipedia.org/wiki/Gravitational_constant
But like all planetary scale maths any answer would be an gross approximation. Reality would always be different due to poor assumptions. See the first link where the calculated global effective temperature of the Earth is 252 K (−21 °C ; -5.8°F), when greenhouse gases are ignored, in reality the average temperature of the Earth is closer to 288 K (15 °C ; 59°F).
Yes, the politics of earthly human induced climate change (at least 40% of the global total said UCLA climatologist Parker Williams-and of course growing >> 100 million humans/yr https://www.youtube.com/watch?v=3UAzGUtA-Pk) and extraterrestial capitalism-assuming Apophis, some comet, Moon wobble or the other 18,000 potential asteroid events don’t occur. Didn’t the genius Musk propose a low cost and easily maintained and operative orbiting robotic army some years ago, at least for deflecting asteroids? Sorry for straying off topic.
“A few questions. Why. I mean why does NASA put so much stress on finding signs of life? ”
Because it gets headlines aka funding. It answers ‘are we the first ones here’ in some small way.
I don’t know that there’s a ‘big space-lobby’ but astrobiology / xenobiology is a field of study that frequently is part of these missions. There are lots of scientists researching life on Earth to figure out how it started and many see Mars as a way to increase their sample size as well as to better understand the conditions needed for life to form. As to why? It’s not for immediate gain, it’s for the knowledge we gain. Studying extremophiles helps us understand life in general, viruses, and generate new technologies. The chemicals that we take for granted in this global pandemic that let them identify signs of covid in a few smears of snot; were originally found in microbes that live in hot springs. Think of finding new life like dumping the ROM from a chip you found. If you can read the code, you can learn it, and eventually write your own, or modify existing code.
Mars is already ‘sequestered’. Every lander by every nation is sterilized to the best of our ability before we send it to land on the planet. It’s not much use to look for signs of life with dirty fingers. That said, we as a species have almost assuredly contaminated Mars already. There are dozens of reports and publications on the types of life that live in satellite clean rooms used to prepare landers.
“carbon, hydrogen, nitrogen, oxygen, phosphorus, and sulfur. Something like 98% of the biomass on Earth is composed of these six elements; finding them on Mars will be pretty good evidence that life once existed there.”
I mean, CHON are the four most common reactive light elements in the Universe. They’re literally everywhere. And sulfur’s not exactly far down, either. It’d be more surprising if you *didn’t* find them.
Phosphorus is the primary oddball (it’s *way* more common in life than in the Universe) but it’s not clear that it’s actually “necessary” for life as opposed to seriously energetically favorable. As in, you might get life without it, but once life gets adaptable enough to find enough of it, it’ll just take over.
In our solar system the relative distribution, as opposed to the average abundance ( https://commons.wikimedia.org/wiki/File:Elements_abundance-bars.svg ), of elements is going to be related to temperature more than anything else. The further away from the local star you go the more gases can exist as liquids or solids.
I thought that was due to the mass of the elements as they spin around the sun. Heavy stuff falls further down the gravity well, and lighter gases can fall further up. Of course there are rocks in the oort cloud, and gases on Venus, but it’s all about concentrations. Then again, maybe I’m wrong.
It’s slightly more complicated. CHONPS is important*, but if you look at just CNP the ratios they’re found in are also important*. CNP is consistently found in a ratio of 106 :16:1 (a more median value 163:22:1) among Earth plankton. So finding ratios similar to those found on Earth would suggest similar environments possible even lifeforms.
* Important if we take the narrow, unjustified, view that metabolisms of Earth are not limited to just Earth, or that Earth-like life can evolve independent and converge on other planets.
The downside to looking for ratios is that on Earth, biospheres are stable and churning. Looking on places like Mars you’re more likely to find dead biospheres, which almost certainly ended by running *out* of one of the various cycling compounds, which means the ratio will be well out of whack.
I’m really skeptical of the idea that finding *active* life will be all that difficult. On Earth, life radically transformed this planet and continues to do so. If active life gets discovered elsewhere, I think it’ll be flamingly obvious.
I hope that there is no life on Mars, yet, because anything that tough is going to see us as a delicacy.
any life on mars is very likely going to be closely related to earth life,the highest probability bieng a blue green algae or other single celled life of a species present on earth.
there has certainly been transfer of ejecta from mars to earth and
earth to mars over billions of years,some of that had spores on it,
life is tough.
the chance of a truely alien,dangerous life form bieng viable on earth is realy small,but supper extra awsome for getting all worked up about ,oh and china and russia,etc,are going, so declaring a lack of evidence as bieng too risky isnt going to pan.
so its off to mars to hunt aliens with laser beams