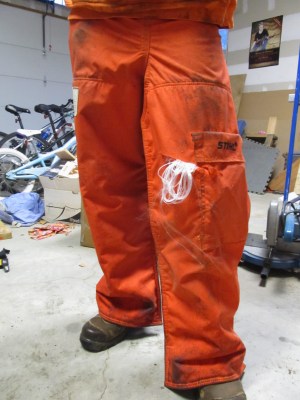
Like most accidents, it happened in an instant that seemed to last an eternity. I had been felling trees for firewood all afternoon, and in the waning light of a cold November day, I was getting ready to call it quits. There was one tiny little white pine sapling left that I wanted to clear, no thicker than my arm. I walked over with my Stihl MS-290, with a brand new, razor sharp chain. I didn’t take this sapling seriously — my first mistake — and cut right through it rather than notching it. The tree fell safely, and I stood up with both hands on the saw. Somehow I lost my footing, swiveled, and struck my left knee hard with the still-running chainsaw. It kicked my knee back so hard that it knocked me to the ground.
In another world, that would likely have a been a fatal injury — I was alone, far from the house, and I would have had mere minutes to improvise a tourniquet before bleeding out. But as fate would have it, I was protected by my chainsaw chaps, full of long strands of the synthetic fiber Kevlar.
The chain ripped open the chaps, pulled the ultrastrong fibers out, and instantly jammed the saw. I walked away feeling very stupid, very lucky, and with not a scratch on me. Although I didn’t realize it at the time, I owed my life to Stephanie Kwolek.