Every high school physics student knows c, or the speed of light, it’s 3 x 10^8 metres per second. More advanced or more curious students will know that this is an approximation, and the figure of 299,792,458 metres per second that forms the officially accepted figure comes from a resonance of the caesium atom from which is derived a value for the second.
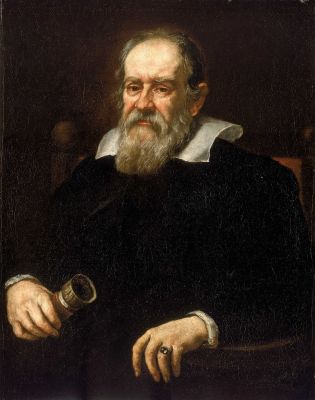
But for those who are really curious about measuring the speed of light the question remains: Just how did we arrive at that figure and how long have we been measuring it? The answer contains some surprises, and some exceptionally clever scientific thought and experimentation over the centuries.
The nature of light and whether it had a speed at all had been puzzling philosophers and scientists since antiquity, but the first experiments performed in an attempt to measure it were you will not be surprised to hear, performed by Galileo sometime in the early 17th century. His experiment involved his observation of assistants uncovering lanterns at known distances away, and his observations failed to arrive at a figure.
Later that century in 1676 the first numerical estimate of the speed of light was made by the Danish astronomer Ole Rømer, who observed an apparent variation in the period of one of Jupiter’s moons depending upon whether the Earth was approaching it or moving away from it. From this he was able to estimate the time taken for light to cross the Earth’s orbit, and from there the mathematician Christiaan Huygens was able to produce a figure of 220,000,000 metres per second.
Spinning Cogs And Mirrors: Time Of Flight
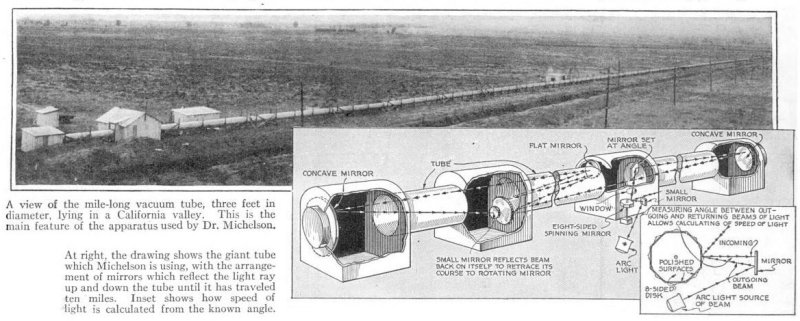
The experiments with which we will perhaps be the most familiar are the so-called time of flight measurements, which take Galileo’s idea of observing the delay as light travels over a distance, and bring to it ever higher precision. This was first performed in the middle of the 19th century by the French physicist Hippolyte Fizeau, who reflected a beam of light from a mirror over several kilometres, and used a toothed wheel to chop it into pulses. The pulses could be increased in frequency by moving the wheel faster until the time taken for the light to travel the distance from wheel to mirror and back again matched the separation between teeth and the returning pulse could be observed. His calculation of 313,300,000 metres per second was successively improved upon through the work of succession of others including Léon Foucault, culminating in the series of experiments by the American physicist Albert A. Michelson in the 1920s. Michelson’s final figure stood at 299,774,000 metres per second, measured through a multi-path traversal of a mile-long evacuated tube in the California desert. In the second half of the century the techniques shifted to laser interferometry, and in the quest to define the SI units in terms of constants, eventually to the definition mentioned in the first paragraph.
The most fascinating part of the story probably encapsulates the essence of scientific discovery, namely that while to arrive at something takes the work of many scientists building on the work of each other, it can then often be rendered into a form that can be understood by a student who hasn’t had to pass through all that effort. We could replicate Fizeau and Michelson’s experiments with a pulse generator, laser diode, and oscilloscope, which while of little scientific value nearly a century after Michelson’s evacuated tube, is still immensely cool. Has anyone out there given it a try?
Header image: Tommology, CC BY-SA 4.0.