Say what you want about the wisdom of keeping a 50-year-old space mission going, but the dozen or so people still tasked with keeping the Voyager mission running are some major studs. That’s our conclusion anyway, after reading about the latest heroics that revived a set of thrusters on Voyager 1 that had been offline for over twenty years. The engineering aspects of this feat are interesting enough, but we’re more interested in the social engineering aspects of this exploit, which The Register goes into a bit. First of all, even though both Voyagers are long past their best-by dates, they are our only interstellar assets, and likely will be for centuries to come, or perhaps forever. Sure, the rigors of space travel and the ravages of time have slowly chipped away at what these machines can so, but while they’re still operating, they’re irreplaceable assets.
bacteria24 Articles
Where No E. Coli Has Gone Before
While we’re still waiting for ET to give us a ring, many worlds might not have life that’s discovered the joys of radio yet. Scientists ran a two-pronged study to see how bacteria might fare on other worlds.
We currently define the Habitable Zone (HZ) of a planet by the likelihood that particular planet can host liquid water due to its peculiar blend of atmosphere and distance from its star. While this doesn’t guarantee the presence of life, its a good first place to start. Trying to expand on this, the scientists used a climate model to refine the boundaries of the HZ for atmosphere’s dominated by H2 and CO2 gases.
Bacterium Demonstrates Extreme Radiation Resistance Courtesy Of An Antioxidant
Extremophile lifeforms on Earth are capable of rather astounding feats, with the secret behind the extreme radiation resistance of one of them now finally teased out by researchers. As one of the most impressive extremophiles, Deinococcus radiodurans is able to endure ionizing radiation levels thousands of times higher than what would decisively kill a multicellular organism like us humans. The trick is the antioxidant which this bacterium synthesizes from multiple metabolites that combine with manganese. An artificial version of this antioxidant has now been created that replicates the protective effect.
The ternary complex dubbed MDP consists of manganese ions, phosphate and a small peptide, which so far has seen application in creating vaccines for chlamydia. As noted in a 2023 study in Radiation Medicine and Protection by [Feng Liu] et al. however, the D. radiodurans bacterium has more survival mechanisms than just this antioxidant. Although much of the ionizing radiation is neutralized this way, it can not be fully prevented. This is where the highly effective DNA repair mechanism comes into play, along with a range of other adaptations.
The upshot of this is the synthesis of a very effective and useful antioxidant, but as alluded to in the press releases, just injecting humans with MDP will not instantly give them the same super powers as our D. radiodurans buddy.
Featured image: Survival mechanisms in Deinococcus radiodurans bacterium. (Credit: Feng Liu et al., 2023)
Life Found On Ryugu Asteroid Sample, But It Looks Very Familiar
Samples taken from the space-returned piece of asteroid Ryugu were collected and prepared under strict anti-contamination controls. Inside the cleanest of clean rooms, a tiny particle was collected from the returned sample with sterilized tools in a nitrogen atmosphere and stored in airtight containers before being embedded in an epoxy block for scanning electron microscopy.
It’s hard to imagine what more one could do, but despite all the precautions taken, the samples were rapidly colonized by terrestrial microorganisms. Only the upper few microns of the sample surface, but it happened. That’s what the images above show.
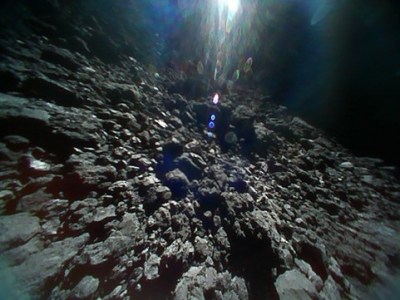
Obtaining a sample from asteroid Ryugu was a triumph. Could this organic matter have come from the asteroid itself? In a word, no. Researchers have concluded the microorganisms are almost certainly terrestrial bacteria that contaminated the sample during collection, despite the precautions taken.
You can read the study to get all the details, but it seems that microorganisms — our world’s greatest colonizers — can circumvent contamination controls. No surprise, in a way. Every corner of our world is absolutely awash in microbial life. Opening samples on Earth comes with challenges.
As for off-Earth, robots may be doing the exploration but despite NASA assembling landers in clean room environments we may have already inadvertently exported terrestrial microbes to the Moon, and Mars. The search for life to which we are not related is one of science and humanity’s greatest quests, but it seems life found on a space-returned samples will end up looking awfully familiar until we step up our game.
Some Bacteria Could Have A Rudimentary Form Of Memory
When we think of bacteria, we think of simple single-celled organisms that basically exist to consume resources and reproduce. They don’t think, feel, or remember… or do they? Bacteria don’t have brains, and as far as we know, they’re incapable of thought. But could they react to an experience and recall it later?
New research suggests that some bacteria could have a rudimentary form of memory of their experiences in the environment. They could even pass this memory down across generations via a unique mechanism. Let’s dive into the latest research that is investigating just what bacteria know, and how they happen to know it.
Continue reading “Some Bacteria Could Have A Rudimentary Form Of Memory”
Ecological System Dynamics For Computing
Some of you may remember that the ship’s computer on Star Trek: Voyager contained bioneural gel packs. Researchers have taken us one step closer to a biocomputing future with a study on the potential of ecological systems for computing.
Neural networks are a big deal in the world of machine learning, and it turns out that ecological dynamics exhibit many of the same properties. Reservoir Computing (RC) is a special type of Recurrent Neural Network (RNN) that feeds inputs into a fixed-dynamics reservoir black box with training only occurring on the outputs, drastically reducing the computational requirements of the system. With some research now embodying these reservoirs into physical objects like robot arms, the researchers wanted to see if biological systems could be used as computing resources.
Using both simulated and real bacterial populations (Tetrahymena thermophila) to respond to temperature stimuli, the researchers showed that ecological system dynamics has the “necessary conditions for computing (e.g. synchronized dynamics in response to the same input sequences) and can make near-future predictions of empirical time series.” Performance is currently lower than other forms of RC, but the researchers believe this will open up an exciting new area of research.
If you’re interested in some other experiments in biocomputing, checkout these RNA-based logic gates, this DNA-based calculator, or this fourteen-legged state machine.
AI Creates Killer Drug
Researchers in Canada and the United States have used deep learning to derive an antibiotic that can attack a resistant microbe, acinetobacter baumannii, which can infect wounds and cause pneumonia. According to the BBC, a paper in Nature Chemical Biology describes how the researchers used training data that measured known drugs’ action on the tough bacteria. The learning algorithm then projected the effect of 6,680 compounds with no data on their effectiveness against the germ.
In an hour and a half, the program reduced the list to 240 promising candidates. Testing in the lab found that nine of these were effective and that one, now called abaucin, was extremely potent. While doing lab tests on 240 compounds sounds like a lot of work, it is better than testing nearly 6,700.
Interestingly, the new antibiotic seems only to be effective against the target microbe, which is a plus. It isn’t available for people yet and may not be for some time — drug testing being what it is. However, this is still a great example of how machine learning can augment human brainpower, letting scientists and others focus on what’s really important.
WHO identified acinetobacter baumannii as one of the major superbugs threatening the world, so a weapon against it would be very welcome. You can hope that this technique will drastically cut the time involved in developing new drugs. It also makes you wonder if there are other fields where AI techniques could cull out alternatives quickly, allowing humans to focus on the more promising candidates.
Want to catch up on machine learning algorithms? Google can help. Or dive into an even longer course.